Figures & data
Figure 1. Mathematical equations for hyperthermia survival curves. A typical hyperthermia survival curve is illustrated in the left panel. Two equations to describe the curve mathematically are shown on the right. While there is no there is no empirical difference between the equations in terms of fitting the data, there is a difference in the number of model parameters that are simple functions of absolute temperature (see text).
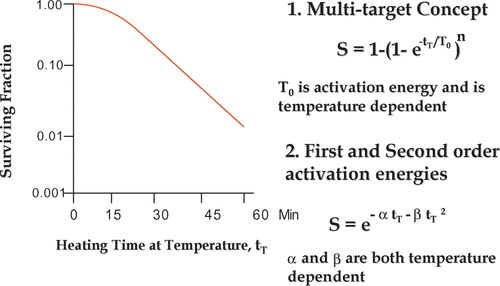
Figure 2. Thermal effects on protein folding. Panel A is an illustration of a model for protein unfolding under hyperthermic conditions, in contrast to the conditions that apply for thermal ablation. As part of the evidence for this model, Panel B shows differential scanning calorimetry data. The peaks represent endothermic transitions. (See text for additional evidence.) Panel C shows the integral of all the endothermic transitions. It can be seen that more than 60% of the endothermic cellular transitions occur in the thermal ablation temperature range 60–70°C. In contrast, less than 10% of the transitions have occurred in the hyperthermic range even for temperatures that reduce survival by 10−2 in less than 15 min Citation[14].
![Figure 2. Thermal effects on protein folding. Panel A is an illustration of a model for protein unfolding under hyperthermic conditions, in contrast to the conditions that apply for thermal ablation. As part of the evidence for this model, Panel B shows differential scanning calorimetry data. The peaks represent endothermic transitions. (See text for additional evidence.) Panel C shows the integral of all the endothermic transitions. It can be seen that more than 60% of the endothermic cellular transitions occur in the thermal ablation temperature range 60–70°C. In contrast, less than 10% of the transitions have occurred in the hyperthermic range even for temperatures that reduce survival by 10−2 in less than 15 min Citation[14].](/cms/asset/9af52b65-6878-4e29-9559-c1548e5664f8/ihyt_a_276976_f0002_b.gif)
Figure 3. Two disparate heat effects on S-phase progression. Hyperthermia causes a greater delay in the completion of DNA synthesis (Panel B) than in the transition from a type I DNA replication pattern to a type II DNA replication pattern. This differential delay causes mis-timing of DNA replication within S-phase and means that early S and mid S DNA replication factories are synthesizing DNA at the same time indicating a loss of check point control. (From Citation[47], used with permission.)
![Figure 3. Two disparate heat effects on S-phase progression. Hyperthermia causes a greater delay in the completion of DNA synthesis (Panel B) than in the transition from a type I DNA replication pattern to a type II DNA replication pattern. This differential delay causes mis-timing of DNA replication within S-phase and means that early S and mid S DNA replication factories are synthesizing DNA at the same time indicating a loss of check point control. (From Citation[47], used with permission.)](/cms/asset/04d56973-2cbc-4676-89c2-834800a2b005/ihyt_a_276976_f0003_b.gif)
Figure 4. Effects on hyperthermia on DNA Supercoiling Ability. (a) illustrates a model of how hyperthermia induced MAR DNA binding can impact DNA supercoiling and the recognition of DNA damage. In the absence of the heat-induced MAR binding proteins single-strand DNA breaks will inhibit DNA supercoil rewinding, but when these proteins bind MAR DNA the effects of these breaks on DNA supercoiling are masked, see (b) which shows some of the supporting data in which DNA damage induced by ionizing radiation is masked in heated cells. The excess halo diameter is illustrated in (c), showing the approximate point at which the difference between control and irradiated samples were measured and added to estimate the difference between the curves. Note that in the samples from heated and irradiated cells the inhibition of DNA supercoil rewinding is reversed due to the masking of the DNA damage. Panel C reprinted from Citation[39].
![Figure 4. Effects on hyperthermia on DNA Supercoiling Ability. (a) illustrates a model of how hyperthermia induced MAR DNA binding can impact DNA supercoiling and the recognition of DNA damage. In the absence of the heat-induced MAR binding proteins single-strand DNA breaks will inhibit DNA supercoil rewinding, but when these proteins bind MAR DNA the effects of these breaks on DNA supercoiling are masked, see (b) which shows some of the supporting data in which DNA damage induced by ionizing radiation is masked in heated cells. The excess halo diameter is illustrated in (c), showing the approximate point at which the difference between control and irradiated samples were measured and added to estimate the difference between the curves. Note that in the samples from heated and irradiated cells the inhibition of DNA supercoil rewinding is reversed due to the masking of the DNA damage. Panel C reprinted from Citation[39].](/cms/asset/9981d6c2-8cbb-4c67-9e45-4fa0ece46707/ihyt_a_276976_f0004_b.gif)
Figure 5. Heat-induced mitochondrial damage and oxidative stress. (a) shows an overview of heat effects on mitochondria leading to a burst of reactive oxygen radicals (ROS). (b) shows novel functionalized indoles. (c) shows the effects of VJ112-OH in combination with 41°C hyperthermia. (d) shows that increasing the burden of ROS destabilizes proteins as measured by differential scanning calorimetry using a model system, in which the redox status of a C++ dependent ATPase had been altered so that the effects of redox status on the endothermic transitions characteristic of unfolding could be observed.
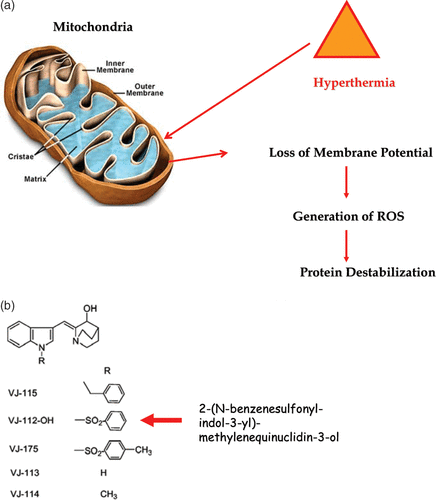
Figure 6. An illustration integrating the examples of hyperthermia effects discussed in the text. (a) is a simplified drawing of the effects described. (b) is a statement of an integrating model linking effects in the plasma membrane and the mitochondria to protein effects in the nucleus, cell killing and radio- or chemo- sensitization.
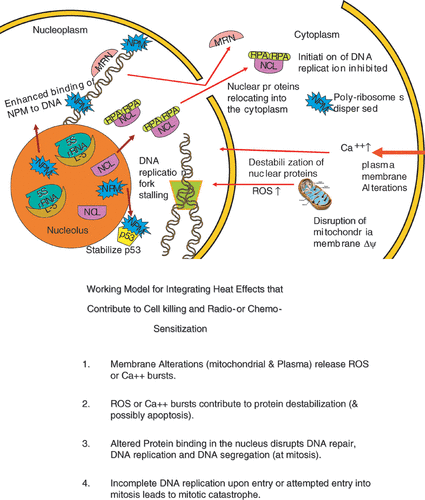