Figures & data
Figure 1. Correlation between chromosome aberration formation and cell killing in cells exposed to radiation alone (X), or to heat plus radiation (DX), in different phases of the cell cycle as indicated. Heat exposure (45.5°C) time was adjusted to give 40% survival in all cell populations tested. The results are from Dewey et al. Citation[1]. (A) Survival curves and the corresponding chromosome aberration induction curves (deletions plus exchanges) for synchronous CHO cells treated at Mitosis, as well as the G1- and S-phase of the cell cycle. The data show a good correlation between hyperthermic radiosensitization as measured by cell lethality and hyperthermic radiosensitization as measured by chromosomal aberrations. This provides indirect evidence that heat sensitizes cells to radiation by impairing the repair of lesions that have the potential of causing chromosomal aberrations. On the basis of their properties, DSBs are likely candidates for this effect. Note the enhanced radiosensitization of S-phase cells as compared to G1 cells, as well as the lack of radiosensitization in M-phase cells. The relevance of the observations with M cells is discussed later in the text. (B) The data of panel (A) re-plotted for survival as a function of chromosome aberrations per cell. Note that at a survival of 37%, which corresponds to one lethal event per cell, nearly one chromosome aberration is also recorded per cell.
![Figure 1. Correlation between chromosome aberration formation and cell killing in cells exposed to radiation alone (X), or to heat plus radiation (DX), in different phases of the cell cycle as indicated. Heat exposure (45.5°C) time was adjusted to give 40% survival in all cell populations tested. The results are from Dewey et al. Citation[1]. (A) Survival curves and the corresponding chromosome aberration induction curves (deletions plus exchanges) for synchronous CHO cells treated at Mitosis, as well as the G1- and S-phase of the cell cycle. The data show a good correlation between hyperthermic radiosensitization as measured by cell lethality and hyperthermic radiosensitization as measured by chromosomal aberrations. This provides indirect evidence that heat sensitizes cells to radiation by impairing the repair of lesions that have the potential of causing chromosomal aberrations. On the basis of their properties, DSBs are likely candidates for this effect. Note the enhanced radiosensitization of S-phase cells as compared to G1 cells, as well as the lack of radiosensitization in M-phase cells. The relevance of the observations with M cells is discussed later in the text. (B) The data of panel (A) re-plotted for survival as a function of chromosome aberrations per cell. Note that at a survival of 37%, which corresponds to one lethal event per cell, nearly one chromosome aberration is also recorded per cell.](/cms/asset/e73b8479-cea0-4362-a8f9-e4779a6da8fb/ihyt_a_278504_f0001_b.gif)
Figure 2. Outline of the main pathways of DNA DSB repair. In addition to HRR utilizing genes of the RAD52 epistasis group, cells of higher eukaryotes also employ NHEJ. There are two pathways of NHEJ: the classical pathway utilizes Ku and DNA-PKcs and carries out ligation using DNA Ligase IV (D-NHEJ). Other known components of this pathway include factors involved in end-processing (Tdp1, PNKP, Artemis) and DNA polymerization (Pol μ, λ). In addition to D-NHEJ, cells are capable of mediating end joining via a pathway operating as a backup (B-NHEJ). There is evidence that this pathway utilizes the DNA Ligase III/XRCC1/PARP-1 module.
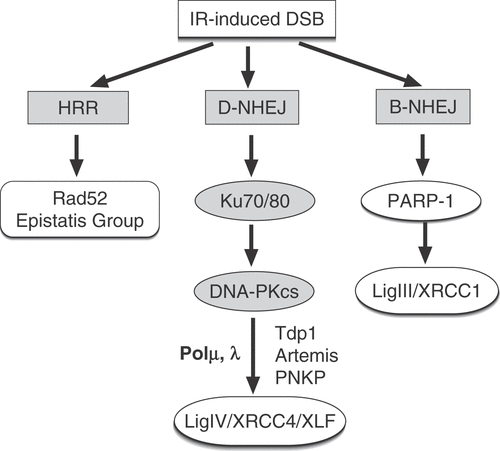
Figure 3. The contribution of HRR and D-NHEJ to heat radiosensitization. (A) Survival curves of plateau-phase CHO cells irradiated and tested immediately after exposure to heat for the indicated times and temperatures. Note the significant heat radiosensitization achieved Citation[18]. (B) Similar data for the Ku80 deficient mutant xrs-5. Note the markedly reduced heat radiosensitization observed Citation[18]. (C) shows the effect of heat shock (45.5°C, 10 min) on the LIG4 deficient 180BRM, as well as in the repair-proficient MRC5SV1 tested during the exponential phase of growth. Note the extensive radiosensitization observed in the LIG4 deficient cells (see text for further discussion) (results obtained from Citation[25]). (D) shows the effect of heat exposure (43°C, 30 min) of the XRCC2 deficient mutant irs-1 and its wild type counterpart V79. Similar heat radiosensitization is observed in the parental cell line and in the HRR deficient mutant (see text for further discussion) (results obtained from Citation[25].
![Figure 3. The contribution of HRR and D-NHEJ to heat radiosensitization. (A) Survival curves of plateau-phase CHO cells irradiated and tested immediately after exposure to heat for the indicated times and temperatures. Note the significant heat radiosensitization achieved Citation[18]. (B) Similar data for the Ku80 deficient mutant xrs-5. Note the markedly reduced heat radiosensitization observed Citation[18]. (C) shows the effect of heat shock (45.5°C, 10 min) on the LIG4 deficient 180BRM, as well as in the repair-proficient MRC5SV1 tested during the exponential phase of growth. Note the extensive radiosensitization observed in the LIG4 deficient cells (see text for further discussion) (results obtained from Citation[25]). (D) shows the effect of heat exposure (43°C, 30 min) of the XRCC2 deficient mutant irs-1 and its wild type counterpart V79. Similar heat radiosensitization is observed in the parental cell line and in the HRR deficient mutant (see text for further discussion) (results obtained from Citation[25].](/cms/asset/2bf76481-0aef-4397-b2dc-09abb5a26d76/ihyt_a_278504_f0003_b.gif)
Figure 4. Theoretical analysis of heat radiosensitization under the assumption that DSBs are the relevant lesions and that different pathways are involved in their repair. (A) outlines a first scenario in which DSBs are repaired by a heat sensitive and a heat resistant pathway. Survival curve A corresponds to the condition where all induced DSBs remain unrepaired and transformed to lethal lesions. This condition is approximated in yeast by several HRR mutants, but remains theoretical for cells of higher eukaryotes. Repair by the heat resistant repair pathway RP1 increases survival to the levels indicated by curve B, whereas repair by the heat sensitive repair pathway RP2 generates survival curve C. Exposure of wild type (WT) cells to heat will compromise RP2 and at the theoretical maximum of the effect will lead to cell survival according to curve B. This is equivalent to extensive radiosensitization. An RP2 mutant will show in the absence of heat the intrinsic radiosensitivity defined by survival curve B. Following exposure to heat the RP2 mutant will sustain no radiosensitization because RP1 is heat resistant. This scenario is implicit in the interpretation of results obtained with cells deficient in either D-NHEJ or HRR. (B) summarizes an alternative scenario in which DSBs are repaired by two heat-sensitive repair pathways; other details as in the first scenario. Exposure of wild type cells to heat will inhibit at the theoretical maximum of effect RP1 and RP2 and will result in survival curve A. This is equivalent to extensive radiosensitization. An RP2 mutant will have in the absence of heat the radiosensitivity defined by survival curve B and exposure to heat will cause significant radiosensitization and will generate at the theoretical maximum of the effect survival curve A. Thus, heat radiosensitization is observed as a result of the heat sensitivity of RP1 and will have a magnitude proportional to the contribution of RP1 to survival. It will be inaccurate to conclude here that RP2 is not involved in heat radiosensitization because the RP2 mutant can be radiosensitized by heat.
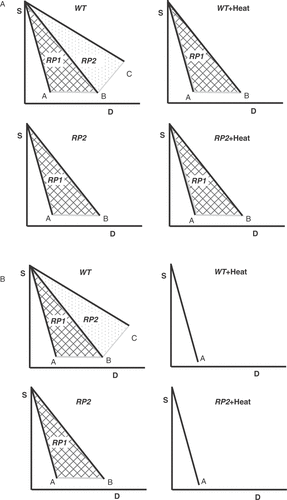
Figure 5. Heat shock inhibits all pathways of DSB repair. (A) Repair kinetics of IR-induced DSBs in plateau-phase CHO cells exposed to 15 Gy X-rays after heating at 45.5°C for the indicated times. Repair of DSBs was measured by neutral filter elution. Note the complete inhibition of DSB rejoining that requires heating for about 30 min. Results are from Citation[19]. (B) Similar results obtained with the Ku80 deficient mutant xrs-5 Citation[19]. Note that in these cells, residual DSB repair, presumably carried out by repair pathways other than D-NHEJ, is already completely inhibited after 8 min of heating suggesting higher heat sensitivity. (C) Repair of DSBs in wild type, exponentially growing MEFs continuously incubated immediately after irradiation (20 Gy) at the indicated temperatures. Note the extensive inhibition of DSB rejoining observed under these conditions as well. Rejoining of DSBs was assayed here by pulsed-field gel electrophoresis. (D) Results similar to those of panel C obtained with LIG4−/− MEFs. Note that here again heat shock has a stronger effect on residual rejoining of DSBs as compared to wt cells.
![Figure 5. Heat shock inhibits all pathways of DSB repair. (A) Repair kinetics of IR-induced DSBs in plateau-phase CHO cells exposed to 15 Gy X-rays after heating at 45.5°C for the indicated times. Repair of DSBs was measured by neutral filter elution. Note the complete inhibition of DSB rejoining that requires heating for about 30 min. Results are from Citation[19]. (B) Similar results obtained with the Ku80 deficient mutant xrs-5 Citation[19]. Note that in these cells, residual DSB repair, presumably carried out by repair pathways other than D-NHEJ, is already completely inhibited after 8 min of heating suggesting higher heat sensitivity. (C) Repair of DSBs in wild type, exponentially growing MEFs continuously incubated immediately after irradiation (20 Gy) at the indicated temperatures. Note the extensive inhibition of DSB rejoining observed under these conditions as well. Rejoining of DSBs was assayed here by pulsed-field gel electrophoresis. (D) Results similar to those of panel C obtained with LIG4−/− MEFs. Note that here again heat shock has a stronger effect on residual rejoining of DSBs as compared to wt cells.](/cms/asset/ace5ad20-b803-4e7c-a0ae-33961d1dee93/ihyt_a_278504_f0005_b.gif)
Figure 6. Rejoining of DSBs in exponentially growing (2d) and plateau phase (4d) MEFs obtained either from wild type or LIG4−/− animals. Cells were exposed to 20 Gy and analysed for DSB repair by pulsed-field gel electrophoresis Citation[30]. Note the reduction in repair capacity of LIG4−/− MEFs as they enter the plateau phase of growth.
![Figure 6. Rejoining of DSBs in exponentially growing (2d) and plateau phase (4d) MEFs obtained either from wild type or LIG4−/− animals. Cells were exposed to 20 Gy and analysed for DSB repair by pulsed-field gel electrophoresis Citation[30]. Note the reduction in repair capacity of LIG4−/− MEFs as they enter the plateau phase of growth.](/cms/asset/fa92b4bf-2f44-42ba-aa93-8eca606c709d/ihyt_a_278504_f0006_b.gif)
Figure 7. Heat radiosensitization has an upper limit. (A) D1, the radiation dose required to reduce survival to 1%, in plateau phase CHO and xrs-5 cells as a function of the resulting cell survival in cells exposed to heat alone. Results obtained from Citation[18]. (B) Survival of exponentially growing CHO cells exposed either to 4 MeV X-rays, or to accelerated 12C ions. Irradiation was carried out after incubation for 1h either at 37°C or at 43°C. Note the absence of heat radiosensitization after exposure to the high LET carbon ions Citation[47].
![Figure 7. Heat radiosensitization has an upper limit. (A) D1, the radiation dose required to reduce survival to 1%, in plateau phase CHO and xrs-5 cells as a function of the resulting cell survival in cells exposed to heat alone. Results obtained from Citation[18]. (B) Survival of exponentially growing CHO cells exposed either to 4 MeV X-rays, or to accelerated 12C ions. Irradiation was carried out after incubation for 1h either at 37°C or at 43°C. Note the absence of heat radiosensitization after exposure to the high LET carbon ions Citation[47].](/cms/asset/7130d557-1b4f-4003-8904-dc875279ba52/ihyt_a_278504_f0007_b.gif)