Figures & data
Figure 1. Schematic diagrams of the ADP‐ribose and phosphate moieties transferred from NAD and ATP onto amino acid side chains during protein‐ADP‐ribosylation and protein‐phosphorylation. ADP‐ribosyltransferases (ARTs) transfer the ADP‐ribose moiety from NAD onto specific amino acid side chains in target proteins (e.g. arginine, asparagine, cysteine, diphthamide or glutamate) while nicotinamide is released. Protein kinases transfer the terminal phosphate of ATP onto specific amino acid side chains (e.g. tyrosine, serine, threonine) in target proteins, while ADP is released. Note that ADP‐ribosylation results in the covalent attachment of a much bulkier group to the target protein than phosphorylation. (ADP = adenosine diphosphate; ATP = adenosine triphosphate; NAD = nicotinamide adenine dinucleotide)
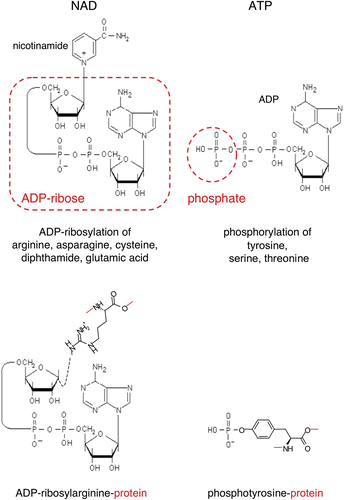
Figure 2. ADP‐ribosylation by bacterial and mammalian ADP‐ribosyltransferases. A: Cholera toxin is a secreted AB5 toxin. The pentameric B subunit binds to the host cell receptor–raft‐associated ganglioside GM1. Following endocytosis, the catalytic A subunit is translocated retrograde through the endoplasmic reticulum (ER) membrane to the cytosol where it ADP‐ribosylates the alpha subunit of heterotrimeric G proteins on an arginine residue. B: Mammalian extracellular mono‐ADP‐ribosyltransferases (ARTs 1–5) most closely resemble the C3/VIP2 family of bacterial toxins. The preferential site of expression is indicated above each ART. ART1 and ART2 ADP‐ribosylate other membrane proteins (mp) or secretory proteins (sp) on arginine residues. Mammalian intracellular poly‐ADP‐ribosyltransferases (pARTs 1–17) bear closer resemblance to diphtheria toxin (DT) and pseudomonas exotoxin A (ETA) than to other bacterial or vertebrate mono‐ADP‐ribosyltransferases. PARP‐1, for example, ADP‐ribosylates nuclear proteins (np) on glutamic acid residues and also catalyzes the extension and branching of poly‐ADP‐ribose polymers on the target protein. (ADP = adenosine diphosphate; NAD = nicotinamide adenine dinucleotide)
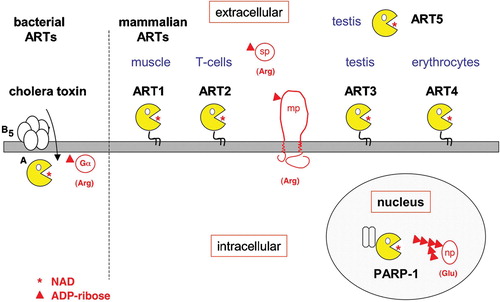
Figure 3. NAD‐induced cell death (NICD). A: Exposure of murine T cells to the ART substrate NAD induces exposure of phosphatidylserine (PS) on the outer leaflet of the cell membrane, which can be detected with a fluorochrome‐conjugate of the PS‐binding protein annexin‐V. NAD‐treatment induces a cascade of reactions culminating in cell death, as evidenced by irreversible staining of cells by the DNA staining dye propidium iodide (PI). NAD‐induced cell death (NICD) has features of both apoptosis and necrosis, including caspase activation, breakdown of the mitochondrial membrane potential, DNA fragmentation, and loss of plasma membrane integrity. B: Fluorescence‐activated cell sorter (FACS) profiles of murine T cells from wild type (ART2+/+) and ART2‐deficient mice (ART2 ‐/‐) stained with fluorochrome‐conjugated annexin‐V and propidium iodide following a 60‐minute incubation in the absence (control) or presence of ecto‐NAD. ART2‐/‐ T cells are resistant to NAD‐induced cell death. Moreover, treatment of ART2‐expressing cells with ART2‐specific antisera blocks cell surface protein ADP‐ribosylation and NICD (not shown), confirming the center stage role of ART2‐catalyzed ADP‐ribosylation of cell membrane proteins in NICD. ‘Spontaneous’ annexin‐V and PI staining of wild type T cells in the control sample is probably caused by NAD released from cells before or during T cell preparation. (NAD = nicotinamide adenine dinucleotide; ART = ADP‐ribosyltransferase; ADP = adenosine diphosphate)
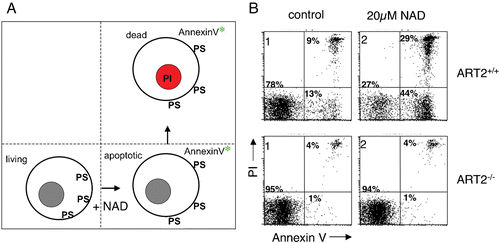
Figure 4. GPI‐anchored ART2 ADP‐ribosylates the cytolytic P2X7 purinoceptor and several other key players of signal transduction on the murine T cell surface. Exposing murine T cells to the ART substrate NAD results in ADP‐ribosylation of several cell surface proteins, including the cytolytic P2X7 purinoceptor, both chains of the integrin LFA‐1, and a 40‐kd protein that is associated with the lck‐tyrosine kinase. ADP‐ribosylation activates P2X7 to from a nonselective cation channel causing influx of calcium and efflux of potassium ions. Its GPI‐anchor ensures that ART2 is associated with specialized, glycosphingolipid enriched membrane microdomains called rafts (indicated by the shaded bar). Raft association focuses the promiscuous enzyme activity of ART2 onto resident raft proteins and proteins that transiently associate with rafts. NAD is much more abundant in the cytosolic than in the extracellular compartment. (GPI = glycosylphosphatidylinositol; ART = ADP‐ribosyltransferase; ADP = adenosine diphosphate)
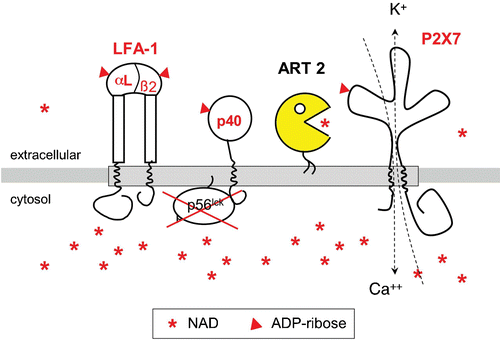
Figure 5. The signaling function of extracellular NAD is relayed by ART2 into ADP‐ribosylated cell surface proteins and is terminated by the hydrolysis of NAD through CD38. NAD concentrations within a cell lie in the mM range (indicated by dark shading), but are 100–10,000 times lower in extracellular tissue fluids. NAD can be released from cells by lytic and nonlytic mechanisms. The extracellular signaling functions of NAD are terminated by its hydrolysis through the CD38 NAD‐glycohydrolase to ADP‐ribose (ADPR) and nicotinamide. The local levels of extracellular NAD are high in the vicinity of damaged cells (light shading) and low in the vicinity of CD38 expressing cells, e.g. B cells. Extracellular NAD levels are relayed by ART2 into ADP‐ribosylated membrane and/or secretory proteins. Naive T cells and regulatory T cells (Tregs) are particularly sensitive to NICD, whereas activated effector T cells shed ART2, resulting in a strongly reduced responsiveness of activated T cells to NAD. (NAD = nicotinamide adenine dinucleotide; ART = ADP‐ribosyltransferase; ADP = adenosine diphosphate; NICD = NAD‐induced cell death)
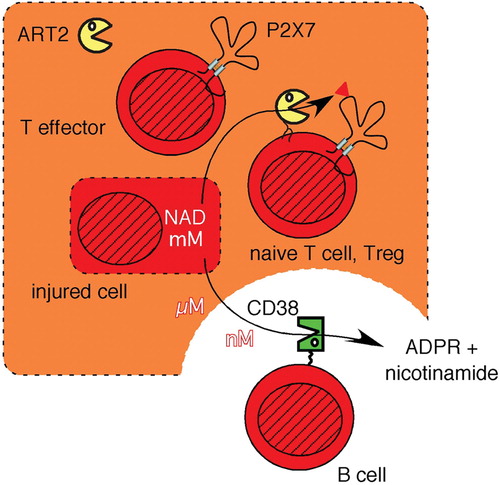