Figures & data
Figure 1. Sub-micron metal rod decorated surfaces; i.e., nanolawn. (A) Production of nano-lawn on isoporous track-etched polymer template. Polymer membranes are coated by gold-sputtering. This layer is enhanced in a platinum or gold plating bath. Polymer pores get filled by cathodic deposition. Polymer template gets removed. Depending on metal plating conditions, multicrystalline pillars with different grain size can be produced. (B) Platinum nano-lawn. (C) Gold nano-lawn. Scale bars of SEM images in B and C represent 1 µm.
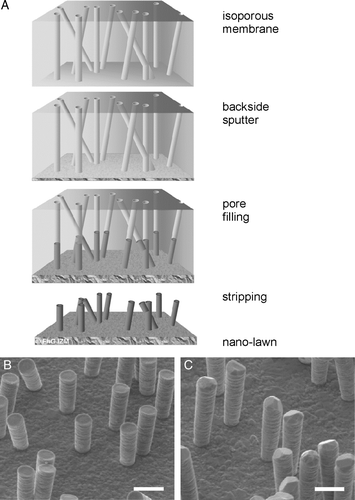
Figure 2. Primary murine astrocytes after 72 h on poly-L-lysin coated glass slides (A, B), platinum (C, D) or gold (E, F) nanolawns. Astrocytes were stained intracellularly with CFSE (A, C and E) or propidium iodide (B, D and F) revealing uncompromised growth and negligible cell death on nano-lawns. Scale bars in A, C and E represent 50 µm; scale bars in B, D and F represent 100 µm. This Figure is reproduced in color in Molecular Membrane Biology online.
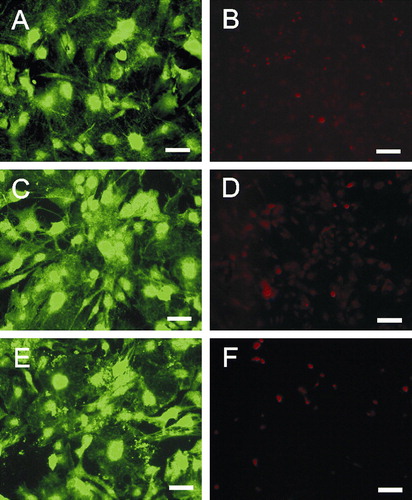
Figure 3. Astrocytes growing on nano-lawns (SEM images). Astrocytes make contact to metal pillars via nanotubular membrane protrusions within 10 min (A, B). They extend nanotubes to distant metal pillars via neighboring pillars while maintaining contact to pillars that were reached before (C). Nanotubes appear straightened in between two attachment points on metal pillars while their correspondent structures grown on poly-L-lysin coated glass surfaces are randomly coiled (D). Nanotubes may extend over several µm at a diameter of about 100 nm (E) but may develop into very long tubes (>100 µm) at a higher diameter which is still <1 µm (F). Astrocytes grow to confluency by making contact to cells further away (G). It can be assumed that astrocytes first bridge the gaps between cells by nanotubular protrusions before closing them with more cellular material (H). Apparently, the material necessary to prolong these nanotubes was transported along the nanotubes in gondola-like structures (H–J) which have been observed as early as 10 min after start of culture (B). Scale bars in A, B, C, D, E, F, G, H and I represent 2, 0.8, 10 (insert: 1), 200, 8, 20, 20, 2, 1 and 1 µm, respectively. Nanostructures were gold (A, B, C, E) or platinum (F, G, H, I, J).
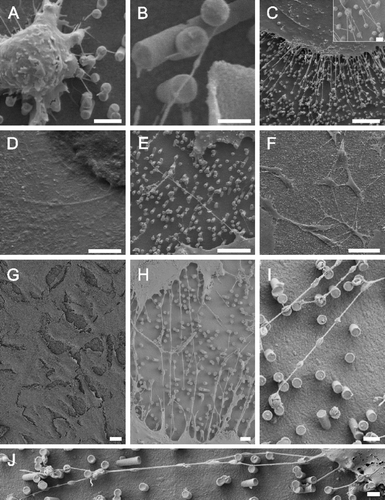
Figure 4. TEM images show that cell bodies and nuclei (marked by ‘N’) were found in between metal pillars (A) while nanotubular protrusions as well as peripheral membrane parts seem to be restricted to the upper parts of the pillars as revealed by SEM images taken at an angle of 45° (B) or 60° (C). The flipped-over cell monolayer in C revealed that it rested on tips of pillars (note imprints of pillars). Scale bars in A, B and C represent 2, 10 and 2 µm, respectively. Nanostructures were gold.
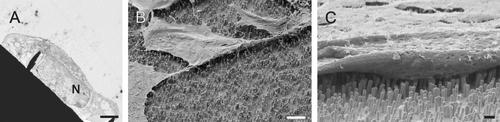
Figure 5. TEM images show that astrocytes make very close contact to the pillars with a distance in the nm range (A and B). SEM image (insert in B) shows that nanotubes end on pillars in a bulb-like contact. Scale bars in A and B represent 200 nm and 1 µm (insert: 2 µm), respectively. Nanostructures were gold.
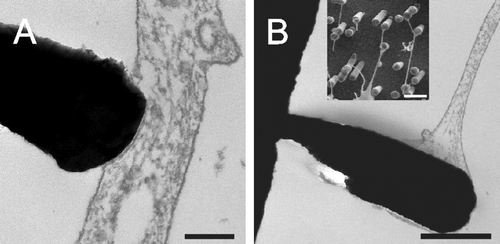
Figure 6. The formation and stability of nanotubes is not affected by latrunculin B. Astrocytes were cultured in the presence of latrunculin B for 1 h (A, B) or 24 h (C, D): 5 µM (A, C); 10 µM (B, D). To test the stability of nanotubes in the absence of actin, astrocytes were seeded onto nano-lawns for 1 h before addition of 5 (E) and 10 µM (F) latrunculin B for another hour. Scale bars in A–F represent 5, 10, 5, 10, 4, and 3 µm, respectively. Nanostructures were gold.
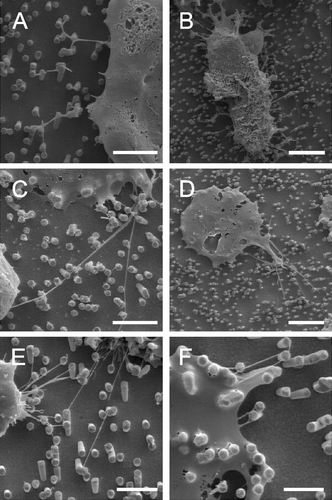
Figure 7. Branched nanotubes forming nets of tubes expanding between cells at different magnifications (A and B). In the same culture, globular, contracted cell bodies covered with a net-like structure (B) were found suggesting that branched nanotubes might not occur when cells colonize nanostructures but rather reflect leftovers of cells having retreated from nanostructures before undergoing apoptosis. Scale bars in A, B and C represent 10, 1 and 2 µm, respectively. Nanostructures were gold.
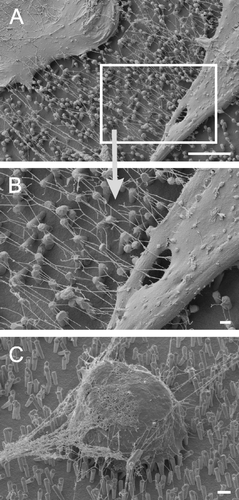
Figure 8. Schematic figure of the favorable shapes of flexible membrane raft elements with different intrinsic (spontaneous) principal curvatures C1m and C2m. (A) isotropic shape for C1m=C2m=0; (B) anisotropic shape for C1m>0 and C2m=0; and (C) anisotropic shape for C1m>0 and C2m<0.
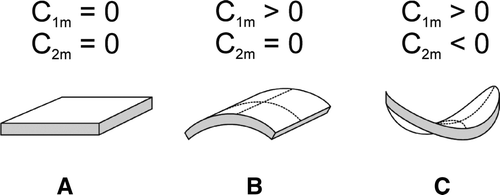
Figure 9. Schematic figure of different orientation of an anisotropic raft element (ARE) with intrinsic principal curvatures C1m>0 and C2m=0 in a barrel-section shaped membrane section with principal curvatures C1>0 and C2=0. Left: energetically favorable orientation. Right: a flexible ARE will be bent in the curvature field of the membrane – unfavorable orientation.
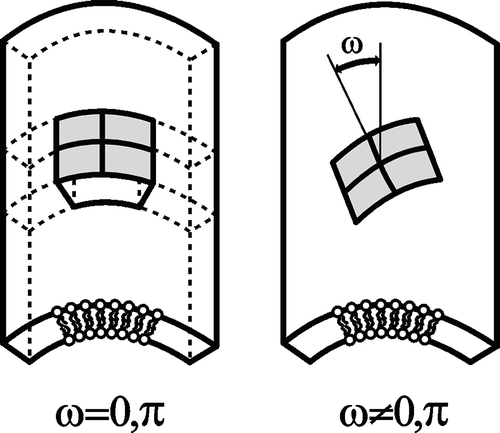