Figures & data
Figure 1 Extrapolation to human exposure when the dose-response curve cannot be assumed to exhibit a threshold. The data in the observable range (typically a response of 10–100% in a cancer bioassay) are used to determine a point of departure (POD) (e.g. NOAEL, BMDL10). The POD is used to extrapolate linearly to a virtually safe dose, that is usually associated with a risk of 1 in 105 or 1 in 106, the choice of value being a risk management decision. Note that the axes are plotted on logarithmic scales, because of the range over which extrapolation is necessary.
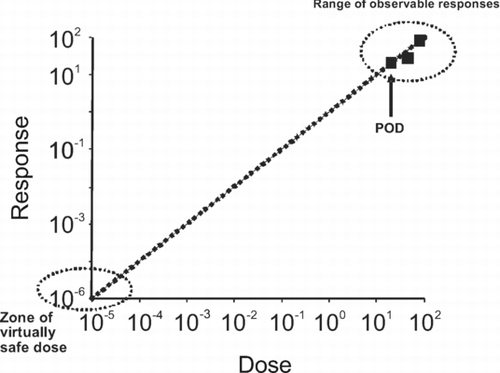
Figure 2 Extrapolation to human exposure when the dose response-curve can be assumed to exhibit a threshold. The data in the observable range are used to determine a point of departure (POD) (e.g. NOAEL, BMDL10). The POD is used to determine the equivalent dose in a sensitive human by dividing it by an uncertainty factor, to allow for possible inter-species and inter-individual differences. The default values are normally 10-fold. The inter-species factor is used to determine the POD in an average human (leftward shifted sigmoidal curve). Within the population there will be a range of sensitivities, reflected by a distribution of PODs (Gaussian curve). The factor for inter-individual sensitivity is used to determine the POD in a sensitive subject, which will lie towards the far left of the distribution curve. This POD has been termed a reference value (RV), for example the tolerable daily intake (TDI).
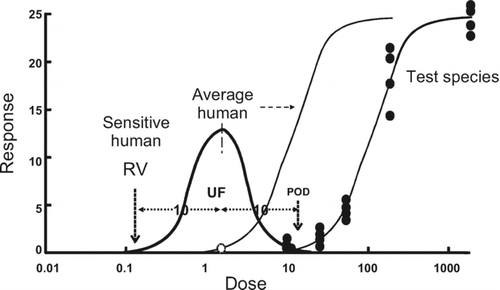
Figure 3 A generic representation of a mode of action for a toxicological effect as a series of key events. Note that often the early (upper) key events will be primarily toxicokinetic while the later (lower) ones will be largely toxicodynamic. Each key event is necessary but not sufficient to produce the toxicological effect. Each key event will exhibit its own dose-response curve, and possibly a threshold. The presence of a threshold, determined by the factors illustrated to the right hand side of the figure, will dictate whether there is progression to the next key event at a given dose. The toxicological effect will be manifest only if all key events operate.
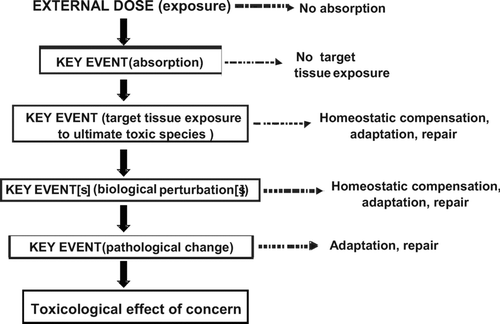
Figure 4 Postulated mode of action and key events for the hepatic toxicity and hepatocarcinogenicity of chloroform. Some of the factors that can influence the dose-response relationships of the key events, and whether they are likely to exhibit a threshold, are illustrated. The early (upper) key events are largely toxicokinetic while the later (lower) key events are largely toxicodynamic.
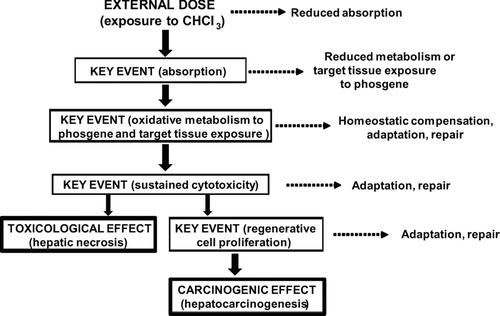
Figure 5 Substrate concentration-reaction velocity curve for an enzyme reaction exhibiting Michaelis-Menten kinetics. The enzyme saturates at high concentrations, reaching a maximum velocity (Vmax). The concentration at which the enzyme velocity is 50% of its maximum is a measure of the enzyme affinity, K m . At low substrate concentrations (up to 50% saturation), the reaction is essentially linear with substrate concentration.
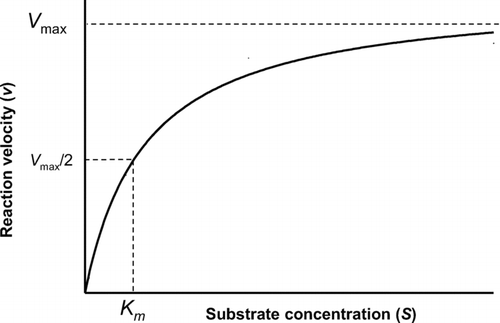
Figure 6 Metabolic activation of chloroform to phosgene and subsequent detoxication by glutathione. Chloroform is oxidized by CYP2E1 to an unstable hydroxylated intermediate, which spontaneously rearranges to the chemically reactive phosgene, the putative toxic species. Phosgene can react spontaneously with glutathione (GSH), the product of which is non-toxic. The glutathione conjugate is further metabolised via γ-glutamyltranspeptidase and cysteinylglycinase to yield 2-oxo-thiazolidine-4-carboxylic acid (OTZ), an unreactive product.
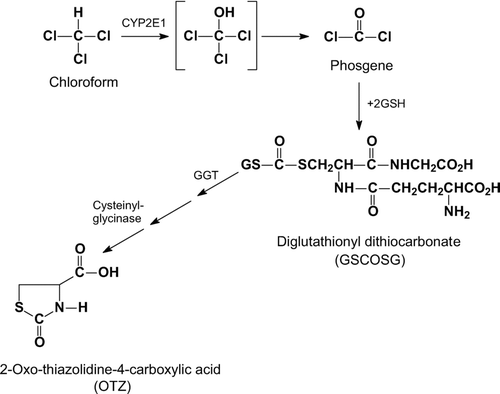
Figure 7 Interindividual variability in human hepatic CYP2E1 content. The illustration is of Western blots of equal protein loading from 30 microsomal samples of human liver, each from a different renal transplant donor, with a highly specific anti-CYP2E1 antibody. Donors had no evidence of liver pathology either histologically or by clinical chemistry. “P” represents a pooled sample used for quality control. (CitationEdwards et al.,1998).
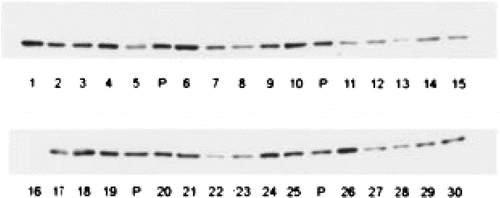
Figure 8 Reversibility of initial damage to hepatocytes. Freshly isolated hamster hepatocytes were exposed to acetaminophen for 90 min (A) and then incubated with the thiol reductant dithiothreitol (DTT) for a further 90 min (B). Despite morphological damage after 90 min, as evidenced by substantial plasma membrane blebbing, hepatocytes could be restored to normal morphology (and function) over the subsequent incubation with DTT, demonstrating that the effects observed at 90 min were fully reversible. If cells were incubated in buffer alone for the second 90 min period there was a marked loss of viability (CitationTee et al., 1986).
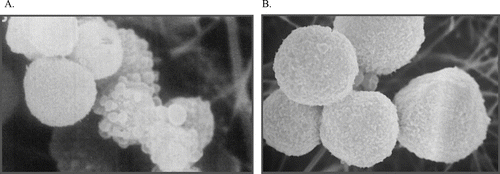