Figures & data
Scheme 1 Inactivation mechanism of a bi-substrate enzyme-catalyzed reaction. The conventional catalytic pathway for an ordered sequential mechanism is shown at the top, with the conversion of EAB to EQ taken to be irreversible. This could occur by release of a product that is then removed by further reaction. Each discrete enzyme species is capable of undergoing conversion to the inactive species F1 to F4, at rates governed by the rate constants j1 to j4, respectively.
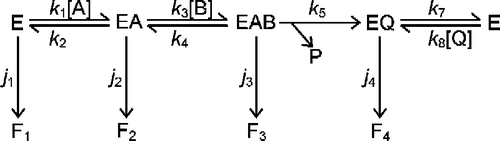
Scheme 2 Simplifications of the mechanism of a bi-substrate enzyme-catalyzed reaction. The conventional catalytic cycle for Scheme 1 is shown in the top panel. Saturation with substrate A effectively removes free enzyme (E) from the cycle (middle panel), while saturation with substrate B results in direct conversion of E to EAB (bottom panel).
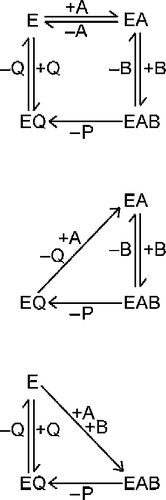
Figure 1 Inactivation of G6P dehydrogenase. Enzyme was incubated at 30°C in 0.1 M Tris-HCl (pH 7.8) containing 0.025% (w/v) SDS. At intervals, samples were removed and assayed for residual activity. The initial activity was 0.22 U/mL.
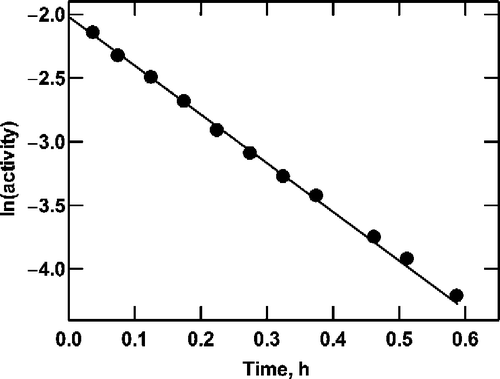
Figure 2 Product formation by G6P dehydrogenase at saturating NAD+. Reactions were as described in the text, containing 10 mM NAD+, G6P as shown (0.5 to 2.0 mM) and initial enzyme concentrations of 70 (•), 56 (○), 42 (▪), 28 (▪) and 14 (▴) mU/mL. A straight line passing through the origin was fitted by linear regression to the data at each enzyme concentration to determine the slope. As shown in the inset, the value of ln(1/slope) is proportional to the enzyme concentration.
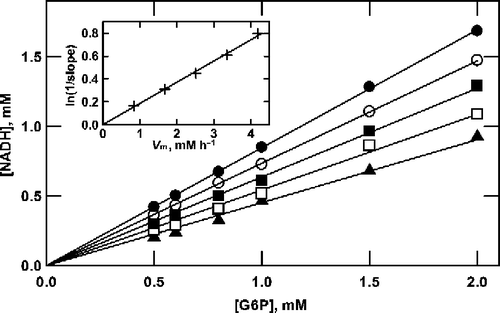
Figure 3 Analysis of product formation by G6P dehydrogenase at saturating NAD+. The data from Figure 2 are plotted according to Equation (11).
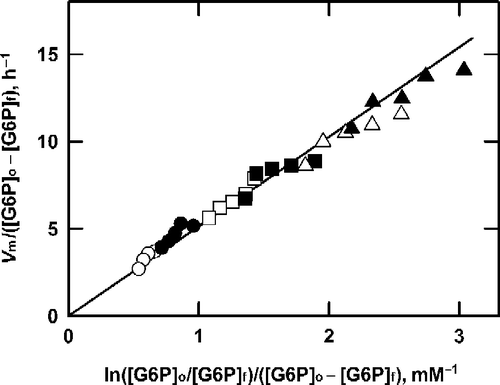
Figure 4 Analysis of product formation by G6P dehydrogenase at saturating G6P. Reactions were as described in the text, containing 10 mM G6P and 150 (•), 100 (○), 70 (▪), 50 (▪) or 30 (▴) μM NADP+. For each concentration of NADP+ the enzyme concentration was varied from 0.69 to 2.76 mU/mL, to obtain the series of data points shown along each line shown. Data are plotted according to Equation (16). As shown in the inset, the slope of the lines in the main graph is proportional to the NADP+ concentration.
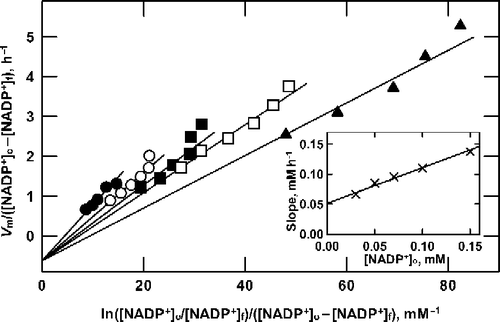
Table I. Analysis of G6P dehydrogenase inactivation during catalysis with saturating G6P. Reactions were as described for Figure 4. Data from experiment 1 were analyzed by nonlinear regression using Equation (16) to obtain best fit values and standard errors for j1, j4 and jb (row 1) The latter was found to be close to zero so the data were reanalyzed with jb=0 (row 2). Experiments 2 (row 3) and 3 (row 4), and the combined results from all three experiments (row 5) were also analyzed with jb=0.