Figures & data
Figure 1. Roles and mechanisms for antigen delivery by immunogenic liposomes following intramuscular injection. liposomes can directly solubilize various adjuvants. Liposome-associated antigens are injected into the muscle and form a depot at the injection site. Antigen-presenting cells (APCs) uptake liposomes and present antigens on the APC surface via major histocompatibility complex (MHC) molecules. Co-delivered adjuvant helps to promote the co-stimulating of T cells. Followed by activated CD4+ and CD8+ T cells to initiate an effective immune response. High-density antigens also lead to B cell receptor crosslinking, followed by co-stimulation between CD4 T cells and B cells, promoting B cell expansion and differentiation.
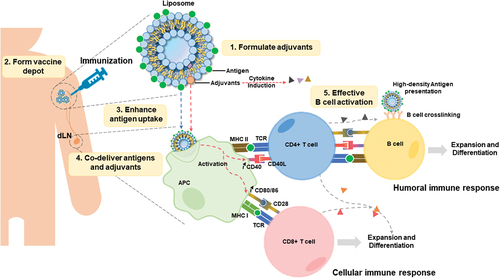
Figure 2. Chemical structure of vaccine adjuvants that localize in lipid bilayers. Representative structures for QS21, MPLA, αGalCer, and 3M–052 are shown.
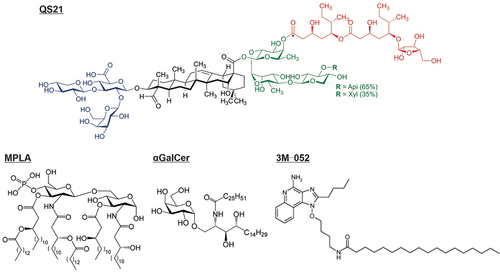
Figure 3. Charged liposomes can capture proteins and mRNA through electrostatic reactions. a) SLP protein spontaneously loads on the cationic liposome surface through electrostatic force [Citation58]. b) mRNA electrostatically adsorbed on lipid-coated PBAE liposome [Citation59]. With permission from International journal of Nanomedicine 2019, originally published by and used with permission from Dove Medical Press Ltd, and American Chemical Society, copyright 2011.
![Figure 3. Charged liposomes can capture proteins and mRNA through electrostatic reactions. a) SLP protein spontaneously loads on the cationic liposome surface through electrostatic force [Citation58]. b) mRNA electrostatically adsorbed on lipid-coated PBAE liposome [Citation59]. With permission from International journal of Nanomedicine 2019, originally published by and used with permission from Dove Medical Press Ltd, and American Chemical Society, copyright 2011.](/cms/asset/960ccafc-e888-4532-8fe7-c1d2971e3fb5/ierv_a_2274479_f0003_oc.jpg)
Figure 4. Modes by which antigens can be captured by liposome, including four types of surface display. Three antigen delivery routes include the admixture without association, encapsulation in an aqueous volume, and displayed on the surface. Under the surface display category, there are four strategies: electrostatic reaction, His-tag-to-metal linkage, lipid headgroup conjugation, and lipid tail anchoring.
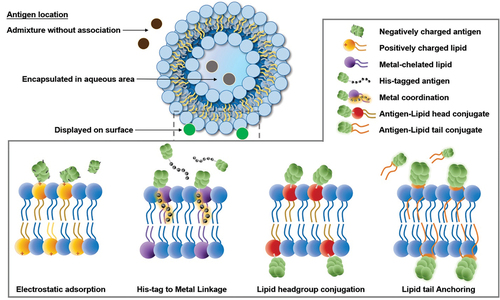
Figure 5. Entrapment of antigens in the aqueous core of liposomes. a) RBD protein antigen encapsulated by DOPC liposome covered with CpG motif DNA [Citation126]. b) OVA protein encapsulated by GM3-αGC liposome [Citation127]. Used with permission from National Academy of Sciences, copyright 2022, and MDPI, copyright 2021.
![Figure 5. Entrapment of antigens in the aqueous core of liposomes. a) RBD protein antigen encapsulated by DOPC liposome covered with CpG motif DNA [Citation126]. b) OVA protein encapsulated by GM3-αGC liposome [Citation127]. Used with permission from National Academy of Sciences, copyright 2022, and MDPI, copyright 2021.](/cms/asset/1e3abbe7-63be-45e7-9688-5a68d7b138ff/ierv_a_2274479_f0005_oc.jpg)
Figure 6. Antigen conjugation with lipid headgroups for antigen display on liposome surfaces. a) an αGalCer-RBD conjugate anchors the lipid bilayer and displays RBD on liposomes [Citation40]. b) Aβ1–16 linked with PEGylated-lysine residues on both C- and N-terminal and anchored with DSPE lipid to display Aβ1–16 antigens [Citation114]. c) fusion peptide anchored on cholesterol (pepE-Bet v 1-CC-CPK-liposome) and displayed peptide on the surface [Citation109]. d) antigen modified with C-terminal cysteine covalently binds with maleimide-based liposome [Citation115]. Used with permission from the American Chemical Society, copyright 2022; National Academy of Sciences, copyright 2007; frontiers, copyright 2023; and American Society for Microbiology, copyright 2017.
![Figure 6. Antigen conjugation with lipid headgroups for antigen display on liposome surfaces. a) an αGalCer-RBD conjugate anchors the lipid bilayer and displays RBD on liposomes [Citation40]. b) Aβ1–16 linked with PEGylated-lysine residues on both C- and N-terminal and anchored with DSPE lipid to display Aβ1–16 antigens [Citation114]. c) fusion peptide anchored on cholesterol (pepE-Bet v 1-CC-CPK-liposome) and displayed peptide on the surface [Citation109]. d) antigen modified with C-terminal cysteine covalently binds with maleimide-based liposome [Citation115]. Used with permission from the American Chemical Society, copyright 2022; National Academy of Sciences, copyright 2007; frontiers, copyright 2023; and American Society for Microbiology, copyright 2017.](/cms/asset/76c4a970-e475-46d3-be30-17ca63664c05/ierv_a_2274479_f0006_oc.jpg)
Figure 7. Surface display of His-tagged antigen with metal chelating liposomes. electron micrographs of a a) plain nanoliposome, b) metal chelated liposome display the N-terminal linked His-tagged proteins (labeled with gold nanoparticles) on the liposome surface [Citation117]. c) His-tagged Env NFL trimer displayed on nickel-bearing liposomes [Citation116]. d) His-tag and cysteine-modified MD39 antigen captured by liposomes containing Ni-NTA and MPB [Citation141]. E) nickle- or cobalt-modified headgroup lipids containing liposome noncovalently capture His-tagged antigens [Citation115]. With permission from PLOS, copyright 2016; cell press, copyright 2019; Nature portfolio, copyright 2018; and American Society for Microbiology, copyright 2017.
![Figure 7. Surface display of His-tagged antigen with metal chelating liposomes. electron micrographs of a a) plain nanoliposome, b) metal chelated liposome display the N-terminal linked His-tagged proteins (labeled with gold nanoparticles) on the liposome surface [Citation117]. c) His-tagged Env NFL trimer displayed on nickel-bearing liposomes [Citation116]. d) His-tag and cysteine-modified MD39 antigen captured by liposomes containing Ni-NTA and MPB [Citation141]. E) nickle- or cobalt-modified headgroup lipids containing liposome noncovalently capture His-tagged antigens [Citation115]. With permission from PLOS, copyright 2016; cell press, copyright 2019; Nature portfolio, copyright 2018; and American Society for Microbiology, copyright 2017.](/cms/asset/5da2273b-e043-4f41-aa46-7512a4652239/ierv_a_2274479_f0007_oc.jpg)
Figure 8. Surface displaying of His-tagged peptide antigen with CoPoP/PHAD/QS21 liposomes as an anti-cancer vaccine. a) His-tagged polypeptides insert into lipid bilayer by binding with CoPoP [Citation104]. b) His-tagged peptide displayed on CoPoP/PHAD/QS21 liposome via coordination with CoPoP lipid [Citation108]. c) Nes2LR captured on liposome CoPoP/PHAD/QS21 induced Nes2LR specific CD8 T cells while Nes2LR adjuvanted with PolyIC is low in immunogenicity [Citation108]. d) schematic of liposomal malaria vaccine immunization based on His-tagged Psf25 protein antigen and CoPoP liposome [Citation107]. Used with permission from Nature, copyright 2015; BMJ, copyright 2021; Springer Nature, copyright 2018.
![Figure 8. Surface displaying of His-tagged peptide antigen with CoPoP/PHAD/QS21 liposomes as an anti-cancer vaccine. a) His-tagged polypeptides insert into lipid bilayer by binding with CoPoP [Citation104]. b) His-tagged peptide displayed on CoPoP/PHAD/QS21 liposome via coordination with CoPoP lipid [Citation108]. c) Nes2LR captured on liposome CoPoP/PHAD/QS21 induced Nes2LR specific CD8 T cells while Nes2LR adjuvanted with PolyIC is low in immunogenicity [Citation108]. d) schematic of liposomal malaria vaccine immunization based on His-tagged Psf25 protein antigen and CoPoP liposome [Citation107]. Used with permission from Nature, copyright 2015; BMJ, copyright 2021; Springer Nature, copyright 2018.](/cms/asset/9cadc986-bab5-454c-a621-e924a490d46d/ierv_a_2274479_f0008_oc.jpg)
Figure 9. Schematic of peptide antigens anchored on liposomes. a) E7 peptide linked with peptide Lys-Ser-Ser which attached with palmitic acid on the Lys residue to form lipopeptide and anchored on lipid bilayer of liposome [Citation111]. b) synthetic CV2 peptide with N-terminal palmitic acid (palm-CV2) anchored CV2 peptide to the lipid bilayer [Citation105]. c) palmitoylated β-amyloid peptide Aβ1–15 displayed on liposome surface [Citation114]. With permission American Chemical Society, copyright 2008; Springer Nature, copyright 2017; and National Academy of Sciences of the U.S.A., copyright 2007.
![Figure 9. Schematic of peptide antigens anchored on liposomes. a) E7 peptide linked with peptide Lys-Ser-Ser which attached with palmitic acid on the Lys residue to form lipopeptide and anchored on lipid bilayer of liposome [Citation111]. b) synthetic CV2 peptide with N-terminal palmitic acid (palm-CV2) anchored CV2 peptide to the lipid bilayer [Citation105]. c) palmitoylated β-amyloid peptide Aβ1–15 displayed on liposome surface [Citation114]. With permission American Chemical Society, copyright 2008; Springer Nature, copyright 2017; and National Academy of Sciences of the U.S.A., copyright 2007.](/cms/asset/8080078d-049e-41a8-8393-64194368b444/ierv_a_2274479_f0009_oc.jpg)