Figures & data
Figure 1. Cartoon illustration of a superinfecting vaccine being introduced into the same population from which the vector came. In actuality, the vaccine is superinfected upon as often as it transmits, but the figure only follows a ‘recipient’ host being repeatedly superinfected upon through time. Colors distinguish vector-infected from vaccine-infected hosts. The bottom row follows one host; the top row depicts its contacts that transmit to it and change its infection state. If, for example, the population is initially 50% wild-type and 50% vaccine, half of encounters are each type, and the host spends 50% of its time in each state. This is an extreme case of superinfection dominating prior infections, but it is especially suited to illustrating how population neutrality applies despite the superinfection.
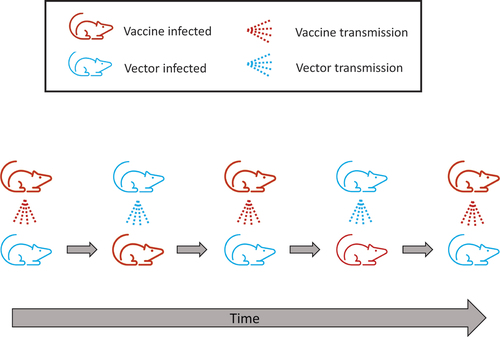
Figure 2. The footprint of vaccine latency, hence immunity, can vary dramatically with changes in host longevity. With superinfection, hosts with latent vaccine contribute to population immunity but are not part of the active vaccine infections and thus can far exceed the population neutrality limit on active infections. This latency ‘footprint’ can be a major benefit to use of a superinfecting vector for a transmissible vaccine. However, superinfection alone does not ensure a large latency footprint – the magnitude depends on infection and host parameters. Two trials of Supplement S2 model (S2.3) are shown, differing in background host death rate (δ); in this model, ‘latent’ infections are considered to be those in which a vaccine infection has been superceded by infection with the wild-type vector. In both trials, vaccine infections at time 300 are introduced at 1/20 the level of active vector infections, and they remain at this level throughout – this is the population neutrality effect whereby the vaccine cannot expand. In contrast, the latency footprint is not determined by just the active vaccine infections but depends – profoundly – on host longevity (the inverse of host death rate). In the top panel, the latent infections (dashed blue) comprise only 0.4% of the total population (measured at time 2000), whereas in the lower panel with reduced host death, latent infections comprise 43% of the total population. Other parameters in the trials are b = 10, β = 1.5 x 10−5. The death rate parameter (δ) also affects the overall level of infections, so the numbers of vaccinated individuals introduced differs between the two trials to achieve 1/20 the level of active vector infections.
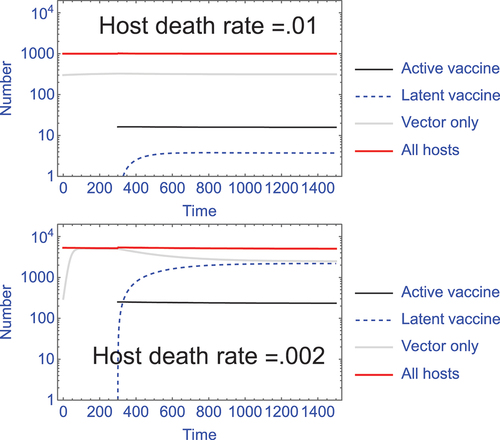
Figure 3. Different possible transmission consequences for a superinfecting transmissible vaccine. The top part of the figure is a key, the lower part depicts a host that started with a vaccine infection (red state) and then became superinfected with wild-type vector (state changed to blue). Qualitatively, there are four cases of possible outcomes from this host regarding the rate it transmits vaccine and vector to new hosts. In the top three cases, the total transmission rate is the same as if it had been infected only once, but the cases differ in whether the transmission is shared between the vaccine and vector or whether the most recent infection dominates the transmission. Those three cases obey population neutrality. At the bottom, the case of independence violates population neutrality because each new superinfection transmits the same regardless of the number of prior infections. These possibilities can be measured experimentally to determine whether a superinfecting transmissible vaccine may escape the expectation of population neutrality.
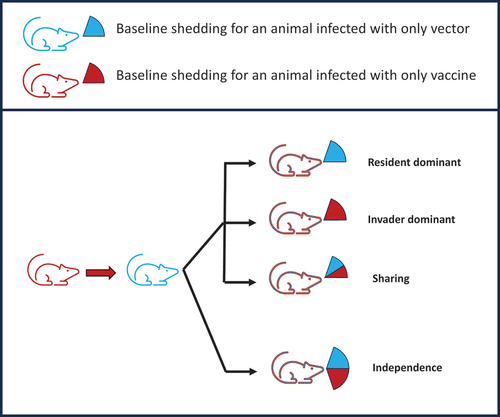
Supplemental Material
Download Zip (779.8 KB)Data availability statement
All data in this study are numbers generated by iteration of equations given in the supplements and displayed in the figures. Initial conditions for these numerical trials are given in the figures. As such, the data are fully reproducible from the information provided.