Figures & data
Figure 1. The schematic representation of the steel production process in an oxygen converter and an electric arc furnace with delineated boundaries of the investigated system.
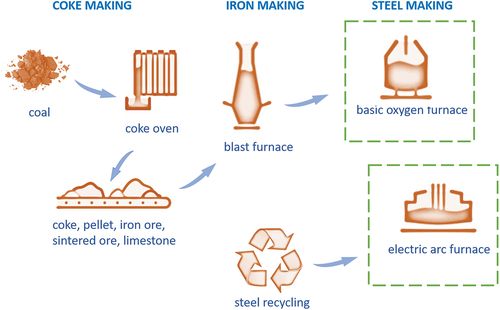
Figure 2. Process scheme for the production of low-alloy steel in an oxygen converter, created in the software LCA for experts.
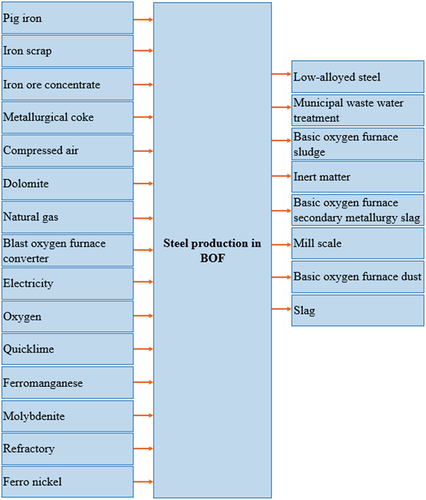
Figure 3. Process scheme for the production of low-alloy steel in an electric arc furnace, created in the software LCA for experts.
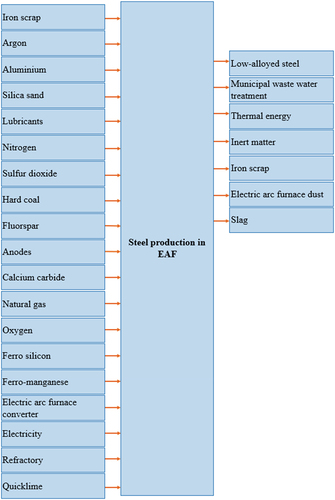
Table 1. Тhe impact on the human body by producing 1 kg of low-alloy steel in BOF and EAF.
Figure 4. Comparison of the impact of the investigated processes on the human body.
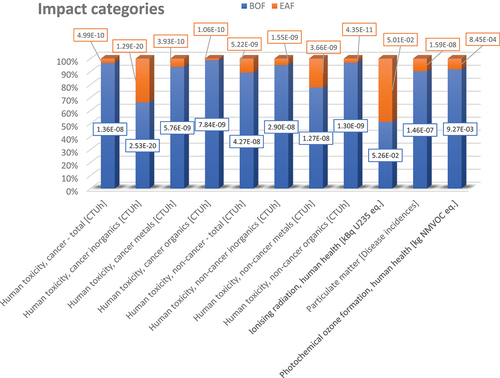
Figure 5. The distribution of the impact on the human body by the process of producing 1 kg of steel in oxygen converters is presented in each of the researched impact categories.
(a) The category of Human toxicity, cancer – total [CTUh]. The production of cast iron and additives such as ferromanganese and ferronickel plays a key role in this category.
(b) The category of Human toxicity, cancer inorganics [CTUh]. Oxygen and additives such as ferronickel play a key role in this category.
(c) The category of Human toxicity, cancer metals [CTUh]. Alloying additives such as ferromanganese and ferronickel play a key role in this category.
(d) The category of Human toxicity, cancer organics [CTUh]. All negative impact in this category is attributed to the process of cast iron production.
(e) The category of Human toxicity, non-cancer – total [CTUh]. The majority of harmful impact in this category is caused by cast iron production, but in addition, ferronickel and refractories exert a noticeable influence.
(f) The category of Human toxicity, non-cancer inorganics [CTUh]. The main portion of hazardous emissions is caused by the process of cast iron production.
(g) The category of Human toxicity, non-cancer metals [CTUh]. Half of the hazardous impact in this category is caused by the steel production process, while the other portion is attributed to refractories and alloying additives.
(h) The category of Human toxicity, non-cancer organics [CTUh]. The majority of the harmful impact in this category is caused by molybdenite, while another portion is attributed to cast iron production.
(i) The category of Ionizing radiation, human health [kBq U235 eq.]. Half of the harmful impact in this category is caused by the process of cast iron production. Additionally, noticeable influence here is attributed to oxygen and alloying additives.
(j) Category Particulate matter [Disease incidences]. In this category, more than half of the hazardous impact is caused by the cast iron production process.
(k) Category Photochemical ozone formation, human health [kg NMVOC eq.]. In this category, the main portion of the hazardous impact is caused by the cast iron production process. Additionally, alloying additives have a noticeable influence on this category.
![Figure 5. The distribution of the impact on the human body by the process of producing 1 kg of steel in oxygen converters is presented in each of the researched impact categories.(a) The category of Human toxicity, cancer – total [CTUh]. The production of cast iron and additives such as ferromanganese and ferronickel plays a key role in this category.(b) The category of Human toxicity, cancer inorganics [CTUh]. Oxygen and additives such as ferronickel play a key role in this category.(c) The category of Human toxicity, cancer metals [CTUh]. Alloying additives such as ferromanganese and ferronickel play a key role in this category.(d) The category of Human toxicity, cancer organics [CTUh]. All negative impact in this category is attributed to the process of cast iron production.(e) The category of Human toxicity, non-cancer – total [CTUh]. The majority of harmful impact in this category is caused by cast iron production, but in addition, ferronickel and refractories exert a noticeable influence.(f) The category of Human toxicity, non-cancer inorganics [CTUh]. The main portion of hazardous emissions is caused by the process of cast iron production.(g) The category of Human toxicity, non-cancer metals [CTUh]. Half of the hazardous impact in this category is caused by the steel production process, while the other portion is attributed to refractories and alloying additives.(h) The category of Human toxicity, non-cancer organics [CTUh]. The majority of the harmful impact in this category is caused by molybdenite, while another portion is attributed to cast iron production.(i) The category of Ionizing radiation, human health [kBq U235 eq.]. Half of the harmful impact in this category is caused by the process of cast iron production. Additionally, noticeable influence here is attributed to oxygen and alloying additives.(j) Category Particulate matter [Disease incidences]. In this category, more than half of the hazardous impact is caused by the cast iron production process.(k) Category Photochemical ozone formation, human health [kg NMVOC eq.]. In this category, the main portion of the hazardous impact is caused by the cast iron production process. Additionally, alloying additives have a noticeable influence on this category.](/cms/asset/d36deb0d-4efe-4c5f-8832-c198f0105d73/tsue_a_2349873_f0005a_oc.jpg)
Figure 6. The distribution of the impact on the human body by the process of producing 1 kg of steel in an electric arc furnace is presented in each of the impact categories.
(a) The category of Human toxicity, cancer - total [CTUh]. The highest impact in this category is caused by dust from electric arc furnaces.
(b) The category of Human toxicity, cancer inorganics [CTUh]. Most of the hazardous effects are due to oxygen.
(c) The category of Human toxicity, cancer metals [CTUh]. In this category, the main exposure is to electric arc dust.
(d) The category of Human toxicity, cancer organics [CTUh]. In this category, the hazardous impact is caused by quicklime and the electricity required for this process.
(e) The category of Human toxicity, non-cancer - total [CTUh]. In this category, electricity and arc dust have a significant impact.
(f) The category of Human toxicity, non-cancer inorganics [CTUh]. More than half of the hazardous impacts are caused by the electricity used.
(g) The category of Human toxicity, non-cancer metals [CTUh]. In this category, the hazardous effects are attributed to arc furnace dust, electrical energy, and refractories.
(h) The category of Human toxicity, non-cancer organics [CTUh]. The largest impact in this category is from the electricity used to run the process.
(i) The category of Ionizing radiation, human health [kBq U235 eq.]. The main impact in this category is caused by electricity.
(j) Category Particulate matter [Disease incidences]. This category is mainly influenced by electricity and alloying additives.
(k) Category Photochemical ozone formation, human health [kg NMVOC eq.]. In this category, the hazardous impact is caused by the electricity used and ferromanganese.
![Figure 6. The distribution of the impact on the human body by the process of producing 1 kg of steel in an electric arc furnace is presented in each of the impact categories.(a) The category of Human toxicity, cancer - total [CTUh]. The highest impact in this category is caused by dust from electric arc furnaces.(b) The category of Human toxicity, cancer inorganics [CTUh]. Most of the hazardous effects are due to oxygen.(c) The category of Human toxicity, cancer metals [CTUh]. In this category, the main exposure is to electric arc dust.(d) The category of Human toxicity, cancer organics [CTUh]. In this category, the hazardous impact is caused by quicklime and the electricity required for this process.(e) The category of Human toxicity, non-cancer - total [CTUh]. In this category, electricity and arc dust have a significant impact.(f) The category of Human toxicity, non-cancer inorganics [CTUh]. More than half of the hazardous impacts are caused by the electricity used.(g) The category of Human toxicity, non-cancer metals [CTUh]. In this category, the hazardous effects are attributed to arc furnace dust, electrical energy, and refractories.(h) The category of Human toxicity, non-cancer organics [CTUh]. The largest impact in this category is from the electricity used to run the process.(i) The category of Ionizing radiation, human health [kBq U235 eq.]. The main impact in this category is caused by electricity.(j) Category Particulate matter [Disease incidences]. This category is mainly influenced by electricity and alloying additives.(k) Category Photochemical ozone formation, human health [kg NMVOC eq.]. In this category, the hazardous impact is caused by the electricity used and ferromanganese.](/cms/asset/0fd8b20d-cfb1-4c02-9abf-7fc700a1a6ee/tsue_a_2349873_f0006a_oc.jpg)
Figure 7. Impact on the human organism of the steel production process in an electric arc furnace using various types of electrical energy.
(a) The category of Human toxicity, cancer – total [CTUh]. In this category, the scenario involving the use of electrical energy generated from wind power will have the highest negative impact, while the safest option will be the use of electrical energy from nuclear power.
(b) The category of Human toxicity, cancer inorganics [CTUh]. In order to minimize the hazardous impact in this category, it is necessary to use electrical energy from hydroelectric power.
(c) The category of Human toxicity, cancer metals [CTUh]. In this case, the use of electrical energy generated from natural gas will have the highest hazardous impact.
(d) The category of Human toxicity, cancer organics [CTUh]. The lowest level of toxic organic substances causing cancer that we can achieve for the investigated process is by using electrical energy from nuclear power. The most hazardous option in this case is the use of electrical energy from biogas.
(e) The category of Human toxicity, non-cancer – total [CTUh]. In this category, the most hazardous is the use of electrical energy obtained from natural gas.
(f) The category of Human toxicity, non-cancer inorganics [CTUh]. In this case, the highest level of danger is associated with the use of electrical energy generated from biogas. The safest option is hydroelectric power usage.
(g) The category of Human toxicity, non-cancer metals [CTUh]. The highest level of danger is posed by the use of electrical energy generated from natural gas.
(h) The category of Human toxicity, non-cancer organics [CTUh]. In this category, the safest option is the use of electrical energy from hydroelectric power.
(i) The category of Ionizing radiation, human health [kBq U235 eq.]. The use of electrical energy from nuclear power provokes the highest level of ionizing radiation emissions.
(j) Category Particulate matter [Disease incidences]. The use of electrical energy from biogas will have the highest emissions level in this category for the investigated process.
(k) Category Photochemical ozone formation, human health [kg NMVOC eq.]. For the investigated process in this category, the use of electrical energy from biogas yields the highest hazardous outcome.
![Figure 7. Impact on the human organism of the steel production process in an electric arc furnace using various types of electrical energy.(a) The category of Human toxicity, cancer – total [CTUh]. In this category, the scenario involving the use of electrical energy generated from wind power will have the highest negative impact, while the safest option will be the use of electrical energy from nuclear power.(b) The category of Human toxicity, cancer inorganics [CTUh]. In order to minimize the hazardous impact in this category, it is necessary to use electrical energy from hydroelectric power.(c) The category of Human toxicity, cancer metals [CTUh]. In this case, the use of electrical energy generated from natural gas will have the highest hazardous impact.(d) The category of Human toxicity, cancer organics [CTUh]. The lowest level of toxic organic substances causing cancer that we can achieve for the investigated process is by using electrical energy from nuclear power. The most hazardous option in this case is the use of electrical energy from biogas.(e) The category of Human toxicity, non-cancer – total [CTUh]. In this category, the most hazardous is the use of electrical energy obtained from natural gas.(f) The category of Human toxicity, non-cancer inorganics [CTUh]. In this case, the highest level of danger is associated with the use of electrical energy generated from biogas. The safest option is hydroelectric power usage.(g) The category of Human toxicity, non-cancer metals [CTUh]. The highest level of danger is posed by the use of electrical energy generated from natural gas.(h) The category of Human toxicity, non-cancer organics [CTUh]. In this category, the safest option is the use of electrical energy from hydroelectric power.(i) The category of Ionizing radiation, human health [kBq U235 eq.]. The use of electrical energy from nuclear power provokes the highest level of ionizing radiation emissions.(j) Category Particulate matter [Disease incidences]. The use of electrical energy from biogas will have the highest emissions level in this category for the investigated process.(k) Category Photochemical ozone formation, human health [kg NMVOC eq.]. For the investigated process in this category, the use of electrical energy from biogas yields the highest hazardous outcome.](/cms/asset/7f2206a1-dfff-41ce-995e-46a592e4b4f5/tsue_a_2349873_f0007a_oc.jpg)
Table 2. Uncertainty analysis of investigated processes.
Table 3. Sensitivity Analysis of Variables for the studied steel production processes.
Data availability statement
The data that support the findings of this study are available from the corresponding author, I. Harasymchuk, upon reasonable request.