Figures & data
Figure 1. Schematic representation of H. pylori pathogenesis and virulence factors. Upon entering the stomach, H. pylori begins to produce urease, which promotes the hydrolysis of urea to synthesize gastric acid. In this way, bacteria can resist gastric acidity and survive in the stomach. With the help of its helical shape and flagellum movement, H. pylori can easily penetrate the mucus layer and reach the gastric epithelium. After reaching the gastric epithelium, H. pylori colonizes gastric epithelial cells under the synergistic action of a variety of adhesins and outer membrane proteins (e.g., BabA, SabA, LabA, OipA, AlpA/AlpB, HopQ, and HopZ). Toxins (e.g., Cag A) are then injected into the host cells through the T4SS, leading to an inflammatory response. In addition, H. pylori can form biofilms composed of bacteria and a self-secreted extracellular matrix that adheres to inert and living surfaces; growth in biofilms facilitates the development of antibiotic resistance in H. pylori.
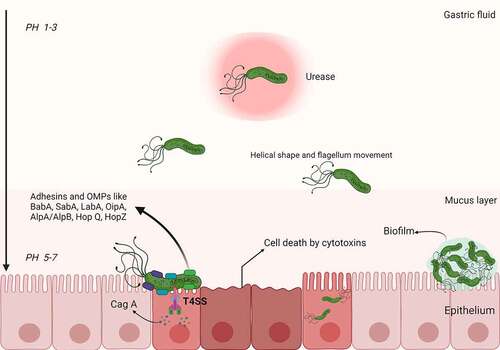
Table 1. The current regimens for H. pylori treatment.
Figure 2. Biological attributes of resistance in H. pylori. The figure shows the biological attributes that drive antibiotic resistance in the H. pylori species (red stars denote the possibility of resistance mutation). It comprises mainly structural changes in genetic sequences that disrupt the cellular activity of antibiotics by altering the drug target (1–3), inhibiting drug activation within the cells (4), biofilm formation (consisting of a matrix of polysaccharides that constitute a multifactorial barrier to drug penetration and activity) (5), reduced drug uptake and increased drug efflux (6), or coccoid formation that generates ultrastructural and metabolic changes that prevent cellular penetration and activity of antibiotic molecules (7). All these attributes are not mutually exclusive but possibly coexist in different strains, jointly leading to three patterns of resistance: single drug resistance (SDR), multidrug resistance (MDR), and heteroresistance (HR). Reproduced with permission from ref,Citation15 © Tshibangu-Kabamba E (2021).
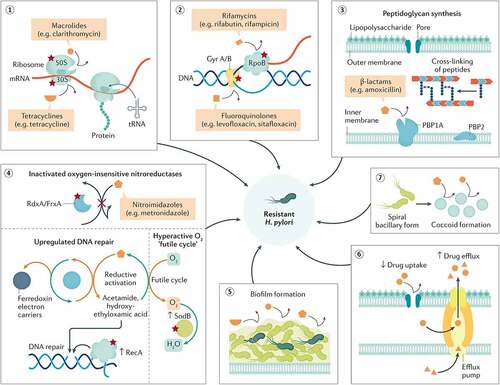
Figure 3. Lipid-based nanoparticles used for H. pylori therapy. a) The schematic drawing shows the molecular structure of LipoLLA, which is composed of phospholipids, cholesterol, and LLA. b) Morphologies of H. pylori SS1 bacteria in the spiral and coccoid forms exposed to different treatments. c) The strategy for lipid polymer nanoparticles to eradicate bacterial biofilms and the eradication of H. pylori plankton in biofilms by nanoparticles. Blank: the blank group with only bacteria; RHL-PC-LPN: amoxicillin-loaded lipid polymer nanoparticles; LPN: using rhamnolipid and phospholipids as mixed lipids; PC-LPN: amoxicillin-loaded LPN: only using phospholipids as the lipid component; PECS + AMX: a mixture of sulfated pectin and amoxicillin; AMX: amoxicillin only; d) The in vitro and in vivo release of NE-P22; a. In vitro release profile of NE-P22; b. In vivo fluorescence imaging of FITC-labeled P22 in mice; c. Quantification of fluorescence intensity; e) Nanostructured lipid carriers kill H. pylori by changing cell-membrane permeability at low concentrations, even without any loaded drug; the eradication does not affect other gut microbiota (such as L. casei and E. coli). (a) and (b) Reproduced with permission from ref,Citation24 © 2012, American Chemical Society; (c) Reproduced with permission from ref,Citation78 © 2015 Elsevier B.V. (d) Reproduced with permission from ref,Citation79 © 2019, The Author(s); (e) Reproduced with permission from ref,Citation80 © 2018 Elsevier B.V.
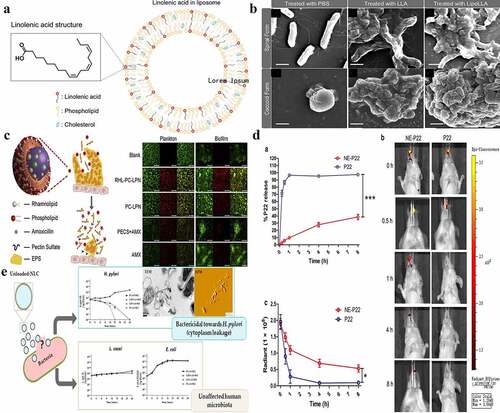
Figure 4. a) A representation of amoxicillin-loaded genipin-FCS/Hep NPs, the strategy and observations for H. pylori eradication using them, and the effects of amoxicillin solution alone and with/without amoxicillin-loaded genipin-FCS/Hep NPs in an H. pylori-induced gastric infection mouse model; b) Schematic illustration of SPIO/AMO@PAA/CHI nanoparticles in the gastric acid of gastric lumen; they adhere to and penetrate the mucus layer, become unstable, and release amoxicillin at the site of H. pylori infection. The distribution of the prepared FITC-SPIO/AMO@PAA/CHI in the mucin layer with/without exposure to a magnetic field for 5 and 10 min. c) Schematic illustration of the structure and bacterial biofilm eradication process of lipid polymer nanoparticles. (a) Reproduced with permission from ref,Citation96 © 2013, Elsevier Ltd. (b) Reproduced with permission from ref,Citation95 © 2020, American Chemical Society (c) Reproduced with permission from ref,Citation98 © 2019, Elsevier B.V.
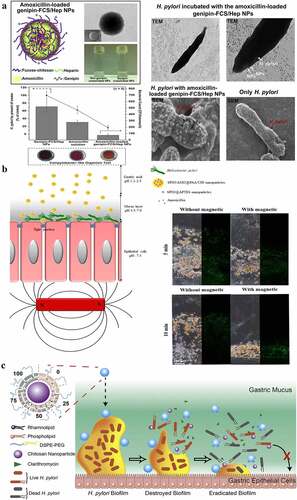
Figure 5. a) Schematic preparation of pH-sensitive GNS@Ab and their application in targeted imaging and photothermal therapy of H. pylori in vivo with antibiotic resistance; b) (a) Schematic diagram of Pd(h)@ZIF-8 and Pd(H)@ZIF-8@AP synthesis and (b) the targeting (i), degradation (II, III), sterilization (IV), regulation of immunity (v), and restoration of gastric mucosa (VI) in the stomach of H. pylori-infected mice, and the influence of intestinal flora homeostasis; c) Schematic illustration of the fabrication and antibacterial process of Janus Ga/Zn micromotors. (a) Reproduced with permission from ref,Citation25 © 2019 Elsevier Inc. (b) Reproduced with permission from ref,Citation23 © 2021 Wiley‐VCH GmbH. (c) Reproduced with permission from ref,Citation115 © 2021 Wiley‐VCH GmbH.
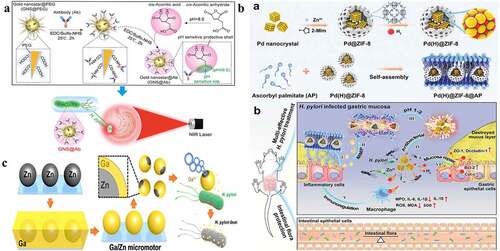
Table 2. The current anti-H. pylori mechanism of biomaterials.
Figure 6. Schematic representation of the main mechanism of H. pylori eradication by biomaterials. (a) Acting as a physical barrier against stomach acids and enzymes, biomaterials provide better stability to the contained drugs. Furthermore, biomaterials delay drug release and target lesions, increasing the contact between H. pylori and the drug, thereby improving drug utilization. (b) Biomaterials directly destroy biofilms (one of the causative factors for H. pylori antibiotic resistance), eradicating H. pylori from biofilms. (c) Biomaterials damaging H. pylori membranes. (d) Biomaterials (such as inorganic metal materials) themselves exhibit antibacterial effects that kill H. pylori directly.
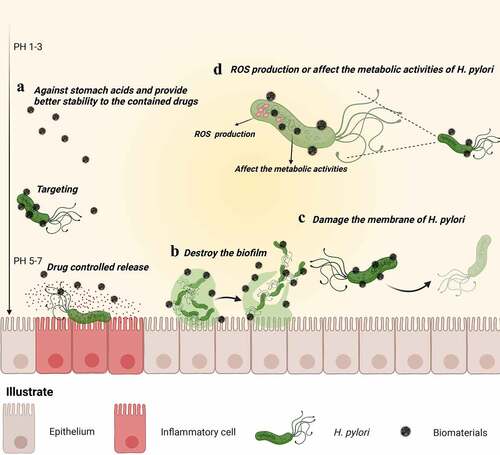