Figures & data
Figure 1 Schematic diagram of skin structure (A), physical trauma (B), chemical trauma (C) and pathological trauma (D).
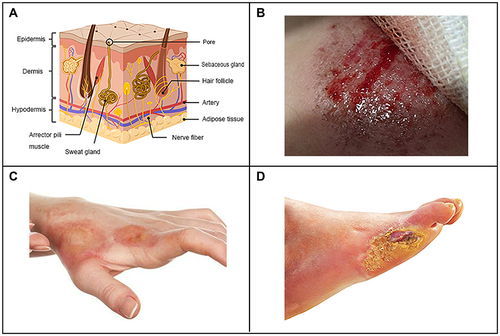
Figure 2 The different stages of normal wound healing. Normal wound healing is a complex biological process that can be divided into three stages: the hemostasis and inflammatory stage, the proliferation stage and the tissue remodeling stage. The inflammatory stage occurs shortly after injury and is characterized by the influx of inflammatory factors. In response to inflammatory signals, neutrophils, macrophages and mast cells are migrate to the wound. As the inflammatory stage subsides and the proliferative phase of tissue repair begins, the dermis and epidermal cells migrate and proliferate excessively in the wound bed. Epithelialization, collagen deposition, angiogenesis, and formation of granulation tissue occur during this stage. The beginning of the stage of tissue remodeling is characterized by matrix remodeling and decreased cell density. At this stage, the wound undergoes contraction to form a scar.
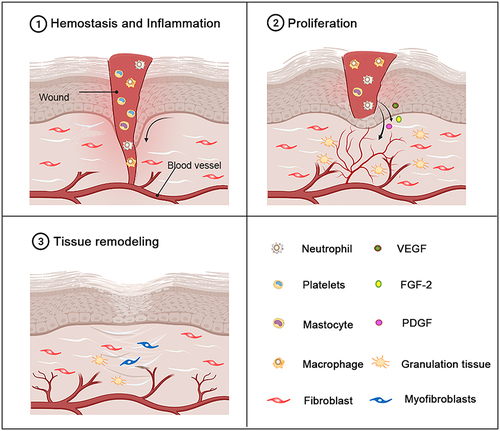
Figure 3 (A) Schematic diagram of the antibacterial mechanism and wound healing mechanism of silver nanomaterials. Exposure to silver nanomaterials (AgNPs) prevents bacterial colonization and inflammation in the wound, thereby promoting wound closure. Adapted with permission from: Lee SH, Jun BH. Silver nanoparticles: synthesis and application for nanomedicine. Int J Mol Sci. 2019;20(4):E865. doi:10.3390/ijms20040865.Citation97 © 2019 by the authors. Licensee MDPI, Basel, Switzerland (http://creativecommons.org/licenses/by/4.0/). And from: Nqakala ZB, Sibuyi NRS, Fadaka AO, Meyer M, Onani MO, Madiehe AM. Advances in nanotechnology towards development of silver nanoparticle-based wound-healing agents. Int J Mol Sci. 2021;22(20):11272. doi:10.3390/ijms222011272.Citation125 Copyright © 2021 by the authors. Licensee MDPI, Basel, Switzerland. Creative Commons Attribution (CC BY) license (https://creativecommons.org/licenses/by/4.0/). (B) Establishment of a mesoporous CeO2 hollow sphere/enzyme nanoreactor and schematic diagram of cascade catalytic antibacterial therapy. Reproduced with permission from: Qin J, Feng Y, Cheng D, et al. Construction of a mesoporous ceria hollow sphere/enzyme nanoreactor for enhanced cascade catalytic antibacterial therapy. ACS Appl Mater Interfaces. 2021;13(34):40302–40314. doi:10.1021/acsami.1c10821.Citation124 Copyright © 2021, American Chemical Society.
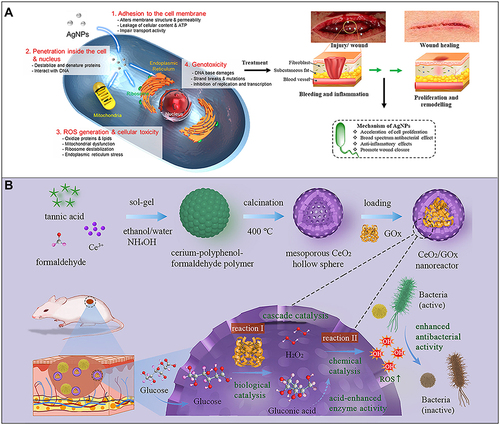
Figure 4 (A) Carboxymethyl chitosan (CMC) combined with dialdehyde-modified cellulose nanocrystal (DACNC) nanocomposite hydrogel for irregular and deep burn wounds. Reproduced with permission from: Huang W, Wang Y, Huang Z, et al. On-demand dissolvable self-healing hydrogel based on carboxymethyl chitosan and cellulose nanocrystal for deep partial thickness burn wound healing. ACS Appl Mater Interfaces. 2018;10(48):41076–41088. doi:10.1021/acsami.8b14526.Citation142 Copyright © 2018, American Chemical Society. (B) Synthesis of bioactive silica-based nanocomposite (PABC) hydrogel scaffold and its potential application and hypothetical mechanism in diabetic wound healing. Reproduced with permission from: Li Y, Xu T, Tu Z, et al. Bioactive antibacterial silica-based nanocomposites hydrogel scaffolds with high angiogenesis for promoting diabetic wound healing and skin repair. Theranostics. 2020;10(11):4929–4943. doi:10.7150/thno.41839.Citation146 Copyright © The author(s). Creative commons attribution license (https://creativecommons.org/licenses/by/4.0/).
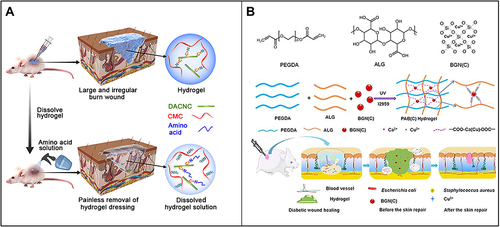
Figure 5 (A) Fe3O4 nanoparticles loaded with basic fibroblast growth factor (bFGF) can greatly promote wound healing by polarizing M2 macrophages and promoting cell proliferation. Reproduced with permission from: Wu J, Zhu J, Wu Q, et al. Mussel-inspired surface immobilization of heparin on magnetic nanoparticles for enhanced wound repair via sustained release of a Growth Factor and M2 Macrophage Polarization. ACS Appl Mater Interfaces. 2021;13(2):2230–2244. doi:10.1021/acsami.0c18388.Citation160 Copyright © 2021, American Chemical Society. (B) A schematic diagram of the use of miRNA-21 mimic nanocarriers in the treatment of skin wounds and the role of miRNA-21 mimics in wound repair by activating cell proliferation and migration. Reproduced with permission from: Wang SY, Kim H, Kwak G, et al. Development of microRNA-21 mimic nanocarriers for the treatment of cutaneous wounds. Theranostics. 2020;10(7):3240–3253. doi:10.7150/thno.39870.Citation166 Copyright © The author(s). Creative Commons Attribution License (https://creativecommons.org/licenses/by/4.0/). (C) Schematic diagram of S-nitrosoglutathione-conjugated poly(lactic acid-glycolic acid) nanoparticles (GPNPs) synthesis and treatment of methicillin-resistant Staphylococcus aureus (MRSA)-infected skin wounds. Reproduced with permission from: Lee J, Kwak D, Kim H, et al. Nitric Oxide-Releasing S-Nitrosoglutathione-Conjugated Poly(Lactic-Co-Glycolic Acid) Nanoparticles for the Treatment of MRSA-Infected Cutaneous Wounds. Pharmaceutics. 2020;12(7):E618. doi:/10.3390/pharmaceutics12070618.Citation183 Copyright © 2020 by the authors. Licensee MDPI, Basel, Switzerland. Creative Commons Attribution (CC BY) license (http://creativecommons.org/licenses/by/4.0/). (D) Preparation of CIP-loaded and ceria-decorated polymer vesicles (CIP-Ceria-PVs) and combination of antioxidants-antibiotics in the treatment of diabetic infected wounds. Reproduced with permission from: Wang T, Li Y, Cornel EJ, Li C, Du J. Combined Antioxidant-Antibiotic Treatment for Effectively Healing Infected Diabetic Wounds Based on Polymer Vesicles. ACS Nano. 2021;15(5):9027–9038. doi:10.1021/acsnano.1c02102.Citation195 Copyright © 2021, American Chemical Society.
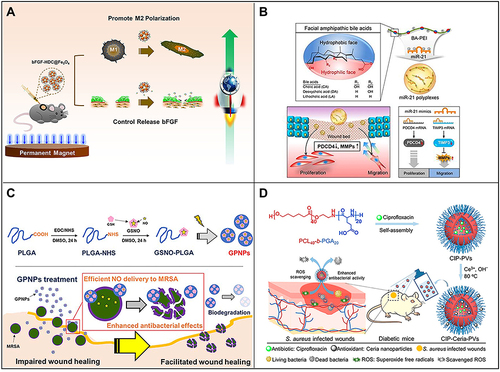
Figure 6 (A) Preparation of silver/copper/graphene oxide nanocomposites and their antibacterial film effect and promotion of wound healing after Pseudomonas aeruginosa infection. Reproduced with permission from: Jang J, Lee JM, Oh SB, Choi Y, Jung HS, Choi J. Development of Antibiofilm Nanocomposites: Ag/Cu Bimetallic Nanoparticles Synthesized on the Surface of Graphene Oxide Nanosheets. ACS Appl Mater Interfaces. 2020;12(32):35826–35834. doi: 10.1021/acsami.0c06054.Citation203 Copyright © 2020, American Chemical Society. (B) Schematic diagram of the wound healing mechanism of arginine-loaded and detachable ceria-graphene nanocomposites (ACG NCS). Reproduced with permission from: Cheng Y, Chang Y, Feng Y, et al. Hierarchical acceleration of wound healing through intelligent nanosystem to promote multiple stages. ACS Appl Mater Interfaces. 2019;11(37):33725–33733. doi:10.1021/acsami.9b13267.Citation204 Copyright © 2019, American Chemical Society.
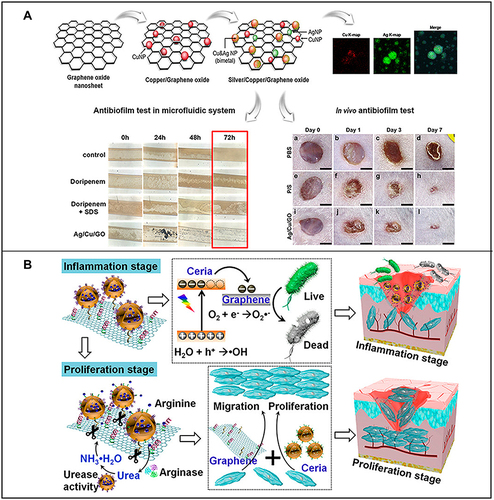