Figures & data
Figure 1. Iron balance in the body. Only 1–2 mg of iron is absorbed from the iron consumed in meals. Iron is required for erythropoiesis, the immune system, brain requirements and for transfer across the placenta in pregnancy. Iron in excess of requirements may be stored in the bone marrow and liver. Iron required for erythropoiesis comes predominantly from the breakdown of red blood cells (RBC) by macrophages; however about 5 % of iron required for RBC formation comes from the newly absorbed iron. The variable component of iron status is that lost in blood such as menstrual loss, blood donation, nose bleeds and gastrointestinal bleeding.
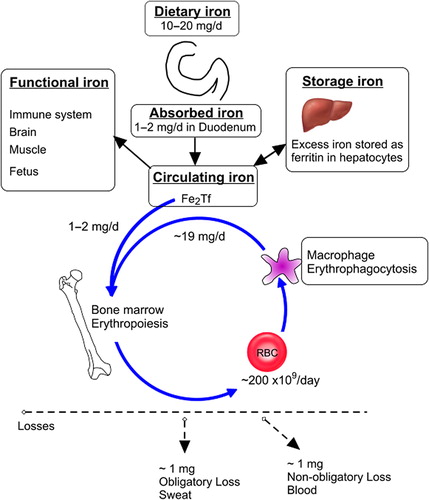
Figure 2. (1) Normal with good iron stores; (2) mild deficiency where the mobilizable iron stores in the bone marrow become depleted but there is normal production of iron-dependent proteins; (3) marginal deficiency or iron deficient erythropoiesis which affects iron-dependent protein production but hemoglobin production and erythropoiesis are preserved; and (4) iron deficiency anemia (IDA) where the production of hemoglobin is compromised and red blood cell synthesis abrogated because there is insufficient iron for incorporation into erythroid precursors.
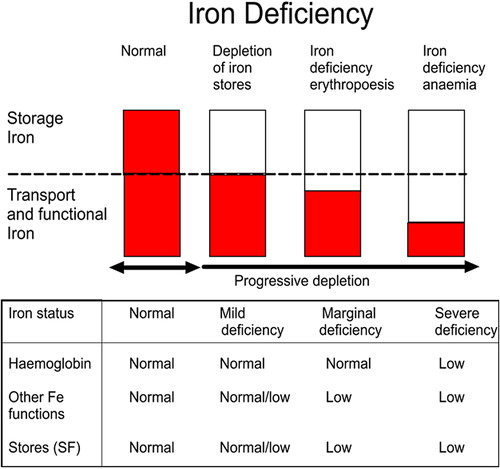
Figure 3. Dietary iron is absorbed across the gut wall as heme iron (from animal food sources) and non-heme iron (from animal and plant food sources). Furthermore, ferritin proteins may be absorbed intact, contributing to the acquisition of non-heme iron. (DMT1 – Divalent metal transporter 1; DCytB – Duodenal cytochrome B; Hox1 – heme oxygenase-1; HCP1 – Heme carrier protein 1).
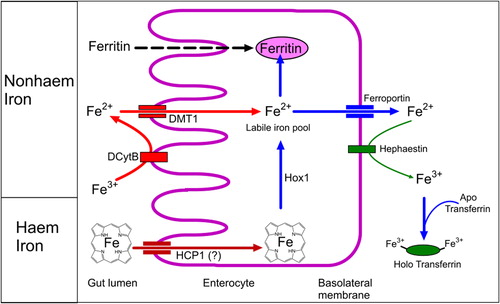
Figure 4. The principal regulator of iron status is hepcidin. Infection and inflammatory conditions increase hepatic hepcidin production via proinflammatory cytokines. Hepcidin binds to ferroportin on enterocytes, macrophages and hepatocytes resulting in its internalization and sequestration of iron in intracellular stores. This decreases iron availability for pathogens but also compromises iron availability for erythropoiesis which can cause functional anemia which is refractory to oral iron therapy.
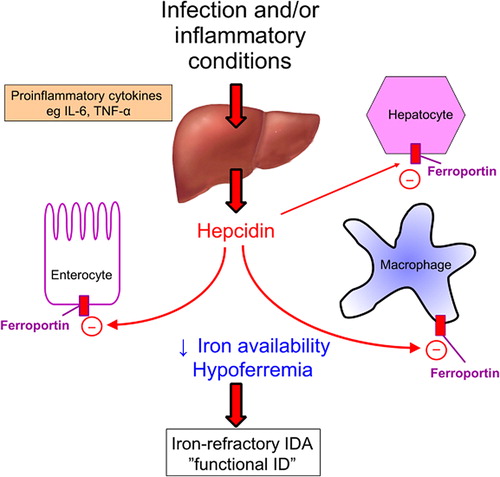
Figure 5. Regulation of iron absorption and uptake: (a) Iron is transported into the enterocytes by the divalent metal transporter (DMT1) and either stored in intracellular ferritin stores or exported out of the enterocyte by ferroportin. (b) When iron status is low, there is upregulation of gut iron transport primarily by an increased expression of DMT1. Also, under these conditions, release of hepcidin from the liver is reduced and more absorbed iron exported from the enterocytes and released from macrophages into the circulation. (c) In iron sufficiency, increased hepcidin production results in the internalization and degradation of ferroportin reducing export of iron from the enterocyte. The increase in intracellular iron results in internalization of DMT1 reducing the absorption of iron.
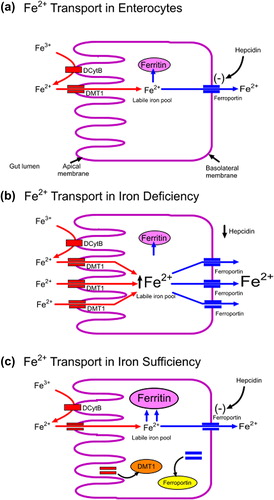
Figure 6. Contributors to iron deficiency and anemia. Blood loss can exceed the ability of the gut to upregulate iron absorption. In women of reproductive age, high menstrual blood loss is the most significant contributor to iron deficiency. In men and older women, blood loss from the gastrointestinal tract is the most common cause of iron deficiency. Diets of low iron bioavailability can reduce iron absorption. Factors, including obesity, that cause raised hepcidin concentrations compromise iron export and limit iron available for erythropoiesis.
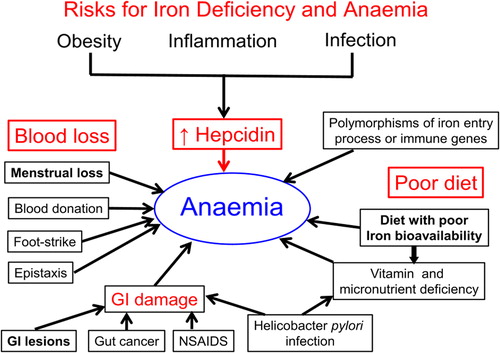