Figures & data
Figure 1. The experimental apparatus used to deliver a focused homogeneous electromagnetic field to growth chambers containing living cells and nanoferrite nanoparticles while monitoring temperature in the cell growth media. A 10 kW AMF power supply was connected to a copper solenoid coil (A1) and used to expose living cells, in the presence or absence of nanoferrite nanoparticles, to an alternating magnetic field. The solenoid coil diameter was designed to accommodate two stacked eight-chamber Lab-Tek devices to bring the cell growth areas into a uniform aspect of magnetic field. The power source was operated at a 150 kHz frequency for 20 min during irradiations. Two temperature probes were inserted through pipette catheters into two growth chamber wells (A2) for all treatments, and temperature of the media in two wells were monitored continuously (B) during and following AMF exposures. Shown are readings for SK-BR-3 cells with Her-2 nanoparticles (black) versus non-directed nanoparticles (grey) collected in parallel under the same AMF exposure. The chiller was set to 33°C and after 20 min of AMF exposure, the media above cells only rose 3°C. This rise in temperature is due to induction coil contact and transference of heat to the Lab-Tek slides. It is observed in wells with medium alone without cells that never received nanoparticles. No cell death is observed using either HMEC or SK-BR-3 cells in control wells without nanoparticles exposed to AMF in parallel and experiencing these temperature ranges during AMF.
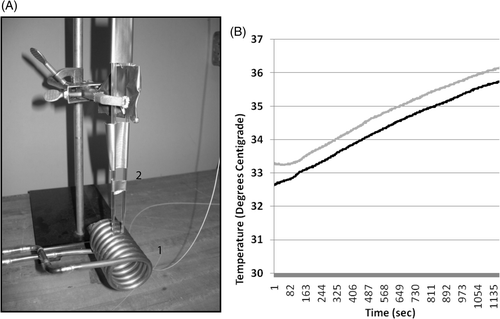
Figure 2. Detection of Herceptin, nuclear morphology and iron in cultures of live SK-BR-3 and HMEC cells. Live SK-BR-3 cells were incubated in medium alone (A–C) (control) or were incubated with Herceptin direct nanoparticles for 1 h (D–F) in parallel with live HMEC cells (G–I). Cells were then washed and fixed and examined using light microscopy for the detection of iron (C, F, I); or subjected to indirect immunostaining to detect retention of Herceptin antibody associated with the cells (A, D, G) or stained with DAPI to reveal nuclear morphology (B, E, H). Only SK-BR-3 cells (D) retained Herceptin-directed nanoparticles, whereas normal mammary cells did not (G). Iron was only found in association with SK-BR-3 cells incubated with Herceptin directed nanoparticles (F). All cells exhibited normal nuclear morphology with no indication of apoptotic bodies occurring in these cultures (B, E, H).
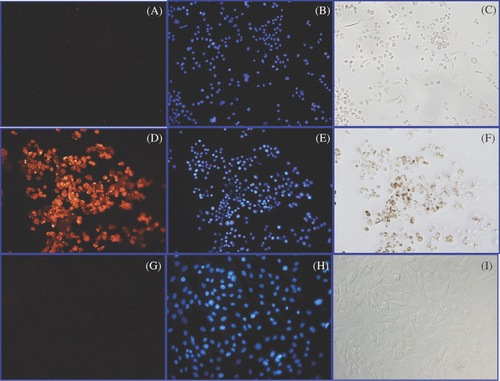
Figure 3. Prussian blue localisation of iron in cultures of HMECs or SK-BR-3 cells treated with Herceptin-directed nanoparticles as a function of time. Live HMEC cells (A–D) and SK-BR-3 cells (E–H) were incubated for either 0 (A, E), 1 (B, F) 2 (C, G) or 3 (D, H) h with Herceptin-directed iron oxide crystal-containing nanoparticles increase. Cells were then fixed and stained to localise iron using Prussian blue. No iron was retained by HMEC cells over the time course, but increasing amounts of iron could be visualised in association with Her-2 positive malignant mammary cancer cells in parallel.
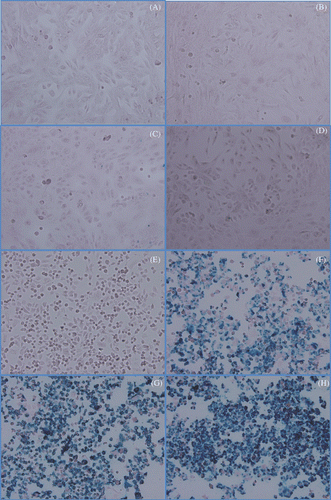
Figure 4. Microscopic study of binding and internalisation of iron oxide crystal containing nanoparticles with or without Herceptin antibody delivery to SK-BR-3 cells. Live SK-BR-3 cells were incubated for 2 h with either non-antibody-directed nanoparticles or the same amount of nanoparticles with Herceptin conjugated to the surface. After washing with PBS and without any fixation or staining, the highly malignant human breast cancer cells could be observed capping (small arrows) and internalising (large arrows) nanomaterial if directed by Herceptin (B), but retained no observable nanomaterial without the benefit of the HER-2 specific antibody (A).
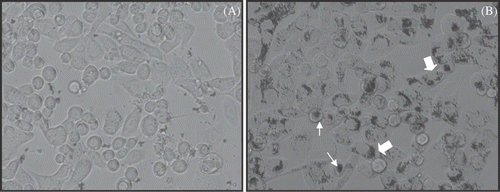
Figure 5. Quantification of non-antibody versus antibody-directed nanoparticle retention and binding to normal versus malignant human mammary cells over time. HMEC cells (A) or SK-BR-3 cells (B) were incubated for 1, 2 or 3 h with either non-antibody directed nanoparticles (grey bars) or Herceptin-directed nanoparticles (black bars) and then washed and stained with Prussian blue. Spectroscopy was used to analyse the amount of iron retained by the cells over time compared to a starting sample of nanoparticles not used with cells processed in parallel to represent 100% binding. These studies reveal a time-dependent increase in binding of Herceptin-directed nanoparticles only to HER-2 positive human cancer cells (lower panel, black bars). Minimal binding of directed or non-directed nanoparticles occurs in response to normal mammary epithelial cells (upper panel) or when non-directed nanoparticles are added to the same Her-2 positive human breast cancer cells (lower panel, grey bars).
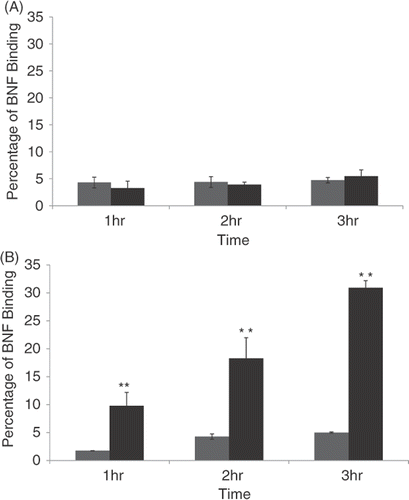
Figure 6. Quantification of HMEC killing before and after AMF exposure following incubation with non-antibody versus antibody-directed nanoparticles using the Live/Dead assay. Live HMECs were incubated in media alone, or with non-antibody-directed nanoparticles (BNF-control) or with Herceptin-directed nanoparticles (BNF-Herceptin) for 2 h. The cells were then washed and treated with a 163 kHz frequency AMF using a steady amplitude of 35,828 A/M for 20 min. Temperature monitoring of the media showed no rise in temperature above 37°C in wells that had been treated with the two types of nanoparticles. Cells were fixed and stained at 6 h using the Live/Dead assay and photographed (upper panels: 40×; calcein (green live) 1/100 s; EthD-1 (red dead) 1/200 s) and cells counted to determine the live/dead cell numbers (lower graphs). Data were pooled for at least duplicate wells and student t-tests used to test the null hypothesis. No killing of normal mammary epithelial cells was seen and cell viability remained at over 90% in all treatment groups. Statistical differences of p < 0.05* or p < 0.01** were not observed.
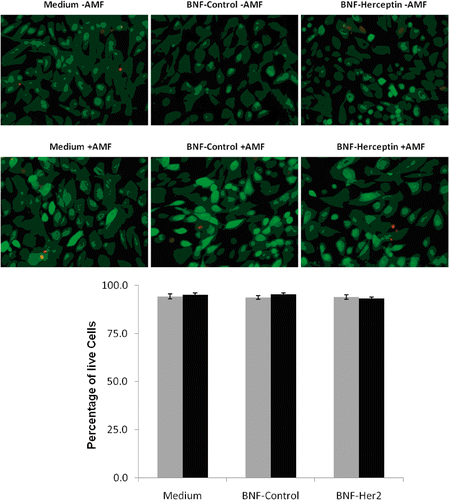
Figure 7. Quantification of SK-BR-3 cell killing before and after AMF exposure following incubation with non-antibody- versus antibody-directed nanoparticles using the Live/Dead assay. Live SK-BR-3 cells were incubated in medium alone, or with non-antibody-directed nanoparticles (BNF-control) or with Herceptin-directed nanoparticles (BNF-Herceptin) for 2 h. The cells were then washed and treated with a 163 kHz frequency AMF using a steady amplitude of 450 Orsteds for 20 min. Temperature monitoring of the media showed no rise in temperature above 37°C in wells that had been treated with the two types of nanoparticles. Cells were fixed and stained at 6 h using the Live/Dead assay and photographed (upper panels: 40×; calcein (green live)1/200 s; EthD-1 (red dead) 1/250 s) and cells were counted to determine the live/dead cell numbers (lower graphs). Data were pooled for at least duplicate wells and student t-tests used to test the null hypothesis. Only the SK-BR-3 cells that had been incubated with Herceptin-directed nanoparticles and exposed to AMF exhibited a statistically increased incidence of cell toxicity in the Live/Dead assay (p < 0.01**).
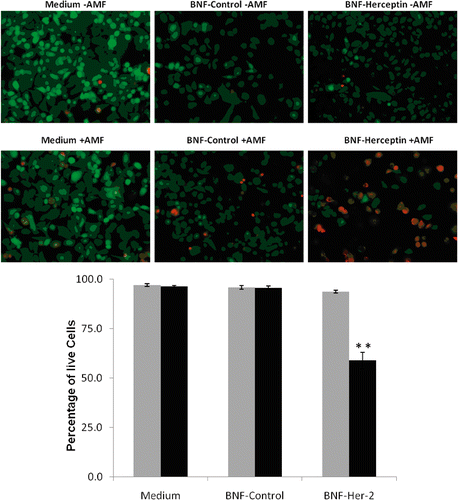
Figure 8. Quantification of lactate dehydrogenase (LDH) release into the media of HMECs or SK-BR-3 cells after incubation with media alone, non-antibody-directed nanoparticles or Herceptin-directed nanoparticles followed by sham or AMF exposures. Lactate dehydrogenase (LDH) was measured in the growth media of HMECs incubated with medium alone, BNF-control and BNF-Herceptin after 24 h (A) and compared to the LDH release by SK-BR-3 cells under parallel conditions at 6 (B) or 24 h (C) after sham (grey) or AMF irradiations (black bars). Total cell lysis standard curves had to be generated in parallel with known numbers of cells. Values are mean ± SE. Statistically significant differences from control are indicated with **; p < 0.01.
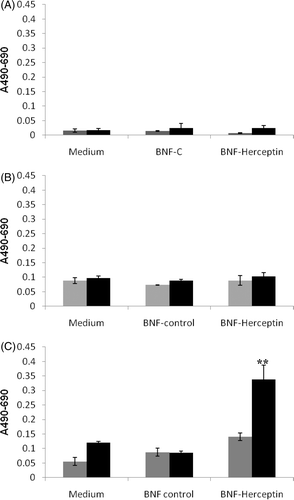
Figure 9. Detection of apoptosis using nuclear morphology in SK-BR-3 cells incubated with Herceptin-directed nanoparticles before and after AMF irradiation. Live SK-BR-3 were incubated with Herceptin-directed nanoparticles for 3 h and then washed and sham treated (A) or AMF treated (B) as above. Cells were fixed and stained 24, 48 (not shown) and 72 h later, using DAPI to stain DNA. Less than 1% of apoptotic cells were seen in sham exposed cultures, but cells that were AMF irradiated had significant apoptosis noted at 72 h.
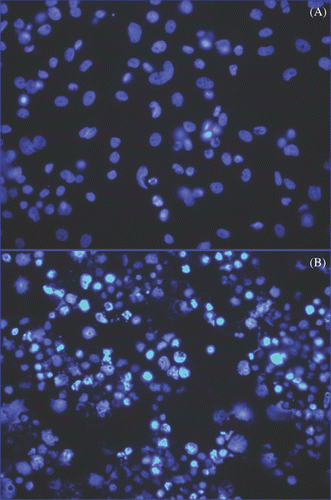