Figures & data
Figure 1. Crystal structures of Type I ABC importers. The TMD subunits are colored pink and deep purple, the NBD subunits are colored light cyan and teal, SBPs orange, RDs dark and light green, the regulation factor EIIA is pale yellow, the substrates are blue spheres and nucleotides are red spheres. From left to right on the top row, the following structures are represented in nucleotide-free conformations: ModBC of A. fulgidus [AfModB2C2-A], ModBC of M. acetivorans [MaModB2C2], and methionine transporter MetN2I2 from E. coli. MaModB2C2 is shown in the inhibited state with molybdate bound to the RDs. The bottom row shows structures of the maltose transporter from E. coli, MalFGK2-E in the following conformations (from left to right): inward-facing; pre-translocation state; outward-facing, nucleotide bound; and inhibited, EIIAglu-bound.
![Figure 1. Crystal structures of Type I ABC importers. The TMD subunits are colored pink and deep purple, the NBD subunits are colored light cyan and teal, SBPs orange, RDs dark and light green, the regulation factor EIIA is pale yellow, the substrates are blue spheres and nucleotides are red spheres. From left to right on the top row, the following structures are represented in nucleotide-free conformations: ModBC of A. fulgidus [AfModB2C2-A], ModBC of M. acetivorans [MaModB2C2], and methionine transporter MetN2I2 from E. coli. MaModB2C2 is shown in the inhibited state with molybdate bound to the RDs. The bottom row shows structures of the maltose transporter from E. coli, MalFGK2-E in the following conformations (from left to right): inward-facing; pre-translocation state; outward-facing, nucleotide bound; and inhibited, EIIAglu-bound.](/cms/asset/3a3ad00d-5bb4-4a10-aec7-85c832951828/ibmg_a_953626_f0001_b.jpg)
Figure 2. Mechanism of Type I ABC importers. Two TMD subunits are colored purple and pink, and two NBD subunits are colored as cyan and magenta. The periplasmic binding proteins are colored orange and the substrate is represented by a blue sphere. ATP and ADP are shown as a red and yellow sphere, respectively. The substrate-loaded SBP binds to inward-facing conformation of transporter (State 1), and induces a partial closure of two NBD subunits (State 2). Binding of ATP triggers closure of the two NBDs, reorientation of TMD subunits, and opening of the SBP, resulting in release of substrate to the TMD subunits (State 3). The dissociation of ADP molecules resets the transporter back to an inward resting state (State 4). The inhibited state (State 5) is shown in brackets; when cytoplasmic substrate levels are high enough, substrate binds the RDs preventing conformation change and ATP hydrolysis of the transporter.
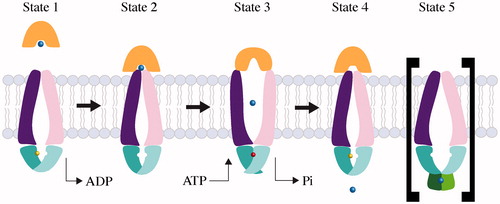
Figure 3. Conformational diversity among Type II importer crystal structures with MolAxis prediction of the translocation pathways. The TMD subunits of each transporter are shown as dark purple and pink tubes, the NBD subunits are teal and cyan, the SBPs are orange, and substrates are light blue. The MolAxis program was used to find and analyze the translocation pathway by fitting the largest possible spheres in along the central cavity (Yaffe et al., Citation2008). Spheres with a diameter <1.4 Å are colored red, 1.4–4 Å green and >4 Å blue. (A) Type II core importers MolB2C2 from H. influenza, HmuU2V2 from Y. pestis and BtuC2D2 from E. coli each solved in the absence of nucleotide. Cognate SBPs (MolA, HmuT and BtuF, respectively) are shown poised above the periplasmic gates. (B) Type II importers solved in complex with SBP. The BtuCD-F structure on the right was solved with AMP-PNP bound (nucleotide not shown).
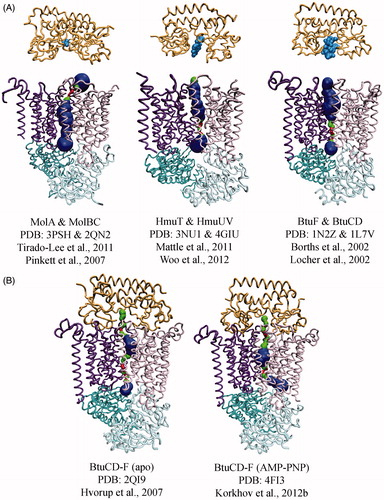
Figure 4. Mechanisms of large and small substrate Type II transporters. The TMD subunits are colored dark purple and pink, the NBD subunits are teal and cyan, the SBP is orange and substrates are light blue. The colors of TMD, NBD, SBP and substrate are the same as in . (A) Transport of a large substrate is initiated when substrate-loaded binding protein docks to the periplasmic surface of the transporter. In State 1, the transporter is shown with ADP (yellow) bound, but the nucleotide-free state is conformationally identical. In State 2, we show SBP docking before ATP due to the higher affinity of transporter for loaded SBP in the absence of nucleotide (Lewinson et al., Citation2010) and the slightly more open conformation of the BtuCD-F periplasmic gate in the absence of nucleotide (Joseph et al., Citation2011). State 3 shows that the binding of ATP (red) traps substrate (blue) in a translocation chamber. Post-hydrolysis, the NBDs open allowing the departure of phosphate and the concurrent collapse of the translocation chamber. Substrate is depicted as it is being squeezed into cytoplasm by the transporter progressing toward an occluded complex (State 4). The transport cycle restarts when the occluded complex disassembles. (B) Transport of a small substrate is initiated when loaded SBP binds to the transporter (State 1). Though small substrate has yet to be trapped in a Type II complex, we suspect that substrate cannot be transferred until ATP binds and the periplasmic gate opens (State 2). After transfer, small substrate may be held in an internal cavity comparable to large substrates (State 3). The possibility of reversion from State 3 to State 2 cannot be ruled out, and may be distinct from the sealed cavity of large substrate Type II transporters. After nucleotide is hydrolyzed, the small substrate transporter returns to an apo-like inward-facing state, from which substrate can enter the cytoplasm (State 4).
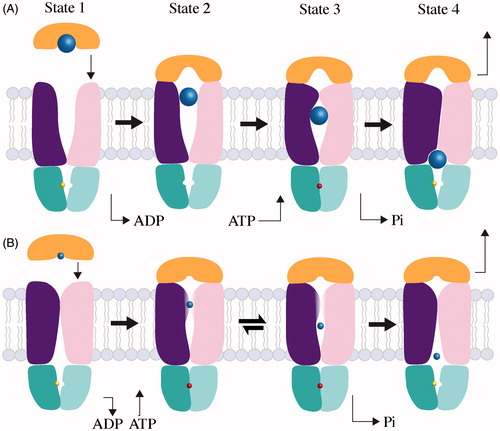
Figure 5. Domain architecture and assembly of Group I and II ECF transporters. ECF transporters contain an energy module (A and A′, colored in teal and cyan respectively), transmembrane component (T, colored in purple) and a substrate-binding module (S, colored in light purple, light blue and light pink). In Group I ECF transporters, S associates with dedicated T, A, A′ units, while Group II ECF transporters utilize the same T, A and A′ components for several different S components.
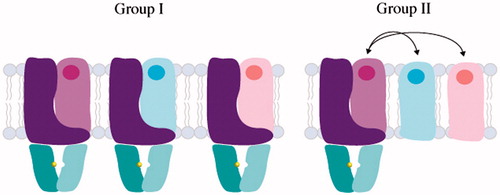
Figure 6. Crystal structures of Type III ECF importers. (A) Cartoon diagrams of S component crystal structures RibU from S. aureus, ThiT and BioY from L. lactis and NikM2 from T. tengcongensis. Each S component is bound to substrate shown in blue stick representation. (B) Crystal structures of full-length, substrate-free folate and HMP transporters from L. brevis. For both full-length structures, the energizing component (shown in purple) predominantly interacts with the substrate bound component in pink. The EcfA and EcfA′ are shown in cyan and teal.
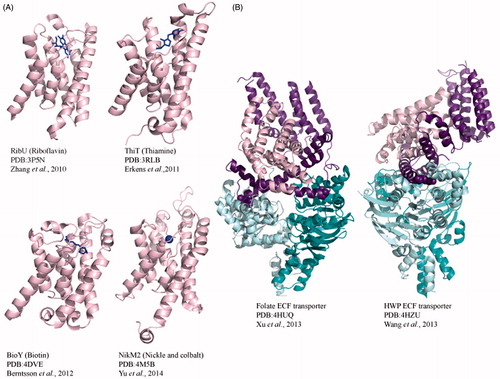