Figures & data
Figure 1. Major steps in the Maillard reaction of reducing sugars following the well-known Hodge scheme.
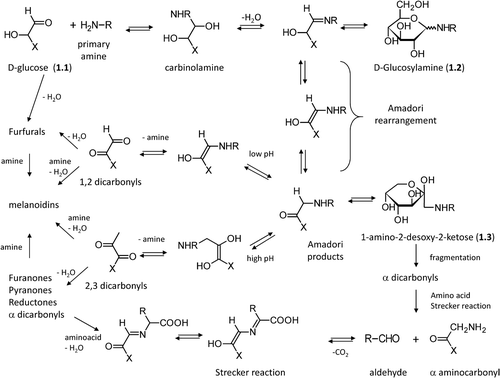
Figure 2. Relevant molecules involved in Maillard reaction. The first row includes α-dicarbolyls, the second row collects cyclization products, and the third row shows carbonyl derivatives generated by sugar fragmentation.
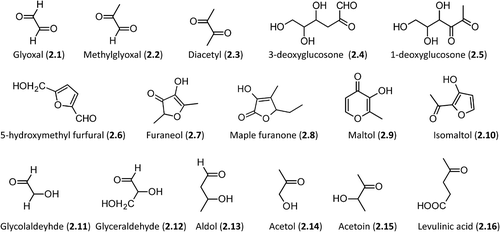
Figure 4. Schematized pathways for MGO formation and catabolism (dotted lines indicate nonenzymatic MGO formation). Adapted from [Citation105].
![Figure 4. Schematized pathways for MGO formation and catabolism (dotted lines indicate nonenzymatic MGO formation). Adapted from [Citation105].](/cms/asset/b0e83dea-b99e-417f-892a-0d9d6206504c/ifra_a_815348_f0004_b.gif)
Figure 8. Major pathways for the formation in vivo of the AGEs deriving from direct rearrangement of the Amadori products.
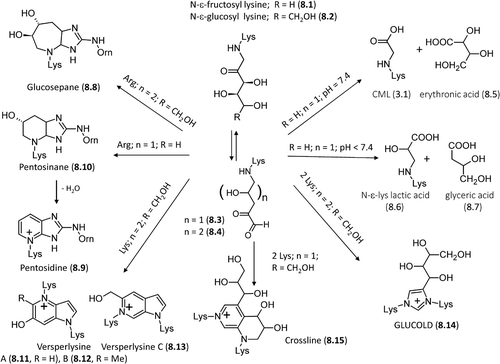
Figure 9. Structures of the most reactive carbonyl species arising from lipidoxidation break-down and prostaglandin metabolism.
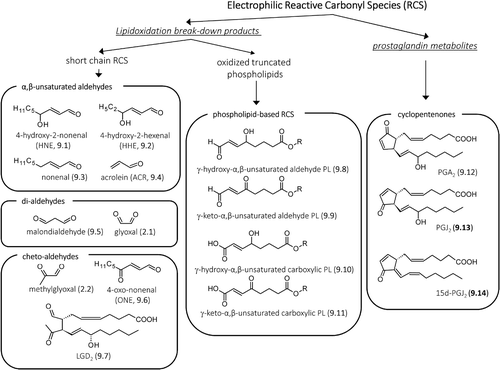
Figure 10. General reaction mechanisms of ALEs formation for hydroxylated and nonhydroxylated α,β-unsaturated aldehydes with specific focus to HNE-derived ALEs.
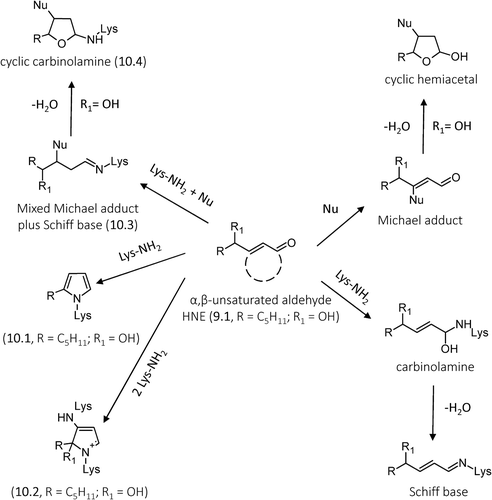
Figure 11. Reaction mechanisms of formation for the ONE-based adducts with nucleophilic sites. Adapted from [Citation22].
![Figure 11. Reaction mechanisms of formation for the ONE-based adducts with nucleophilic sites. Adapted from [Citation22].](/cms/asset/520d43be-3dca-4126-8eab-3c082ae28b30/ifra_a_815348_f0011_b.gif)