Figures & data
Figure 1. Inhibition of the 107 KDa protein band phosphorylation (on tyrosine residues) by different concentrations of prostasomes. Western blot analysis indicates that incubation of spermatozoa with increasing concentrations of prostasomes induces a dose-dependent inhibition of tyrosine phosphorylation of the 107 KDa protein band (Fig. 1a). The lane NI SPZ corresponds to non-incubated spermatozoa therefore non-capacitated spermatozoa. MW stands for molecular weigh markers and SPZ for spermatozoa. The 107 KDa protein band was quantified by densitometry (Fig. 1b) and the results expressed as % of control (spermatozoa incubated without prostasomes). A significant effect was observed with 300 μM and 450 μM of prostasomes. In figure 1b, the values are means ± SEM, n = 5. *significantly different from control (SPZ + 0 μM prostasomes); p < 0.05.
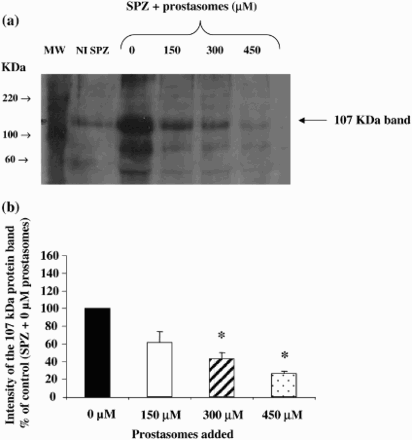
Figure 2. Effect of different concentrations of prostasomes on sperm motility. The sperm motility of spermatozoa incubated in the absence or presence of prostasomes (150, 300 and 450 μM) was analyzed by CASA. The results indicate that addition of prostasomes induced a significant decline of non-progressive motile spermatozoa which was accompanied by an increase of the proportion of immotile spermatozoa. The values are means ± SEM, n = 11. * significantly different (p < 0.05) compared with control (SPZ + 0 μM prostasomes). † significantly different (p < 0.05) compared with 150 μM of prostasomes.
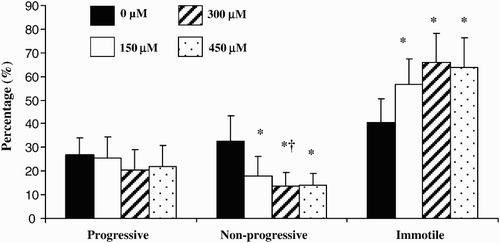
Figure 3. Effect of different concentrations of prostasomes on sperm motion parameters. The addition of prostasomes to spermatozoa resulted in an increase of VAP and VCL. For VAP, only 150 and 300 μM of prostasomes had a significant effect whereas all 3 three concentrations analyzed had a promoting effect on VCL. The table indicates the mean values measured for each parameter considered. The values are means ± SEM, n = 10. * significantly different (p < 0.05) compared with control (SPZ + 0 μM prostasomes). A table with raw data (means ± SEM) for VAP, VSL and VCL (expressed in μm/s) obtained from the CASA analysis is presented.
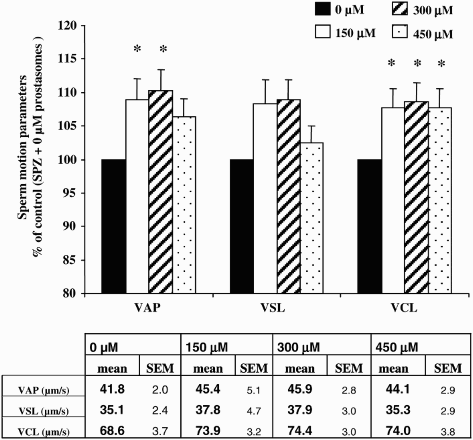
Table 1. Different amounts of prostasomes incubated alone for 3 hours and the recovery of cholesterol in the pellet after centrifugation. The amount of cholesterol recovered before incubation and the percent of cholesterol recovered in the pellets after centrifugation is given.
Figure 4. Pellets lipid composition after incubation of spermatozoa with increasing concentrations of prostasomes. Incubation of spermatozoa with increasing concentrations of prostasomes resulted in a significant enrichment of the pellets in Chol, PE, PC and SM (Fig. 4a). The values are means ± SEM, n = 7 (for Chol, PE and SM) and n = 4 (for PC). * significantly different (p < 0.05) compared with control (SPZ + 0 μM prostasomes), † significantly different (p < 0.05) compared with 150 μM. A picture of the resulting HPTLC plate of the analysis of lipids extracted from spermatozoa alone (control; SPZ + 0 μM prostasomes, lane 1) or spermatozoa incubated with different concentrations of prostasomes (150, 300, 450 μM, lanes 2-3-4 respectively) is shown (Fig. 4b). The amounts of standards spotted on the plate are for PC, PE, SM and Chol: 1, 2, 4, 6 and 8 μg and for PI and PS: 0.5, 1, 2, 3, 4 μg.
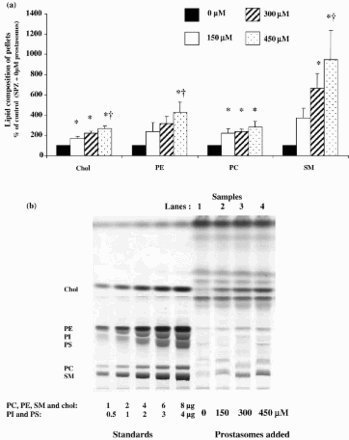
Supplemental Figure 1. Lipid analysis of the two fractions (supernatant and pellets: spermatozoa) obtained after centrifuging spermatozoa incubated with increasing amounts of prostasomes. This HPTLC plate allows better identification of the different sub-classes of lipids analyzed (SM = Sphingomyelin; PC = Phosphatidylcholin; PS = Phosphatidylserin; PI = Phosphatidylinositol; PE = Phosphatidylethanolamin; Chol = Cholesterol). In this new plate, the lines corresponding to PC and SM are more distinguishable. The SM band appears as a doublet and its migration depends on its fatty acid composition which is different from the fatty acid composition of the standards.
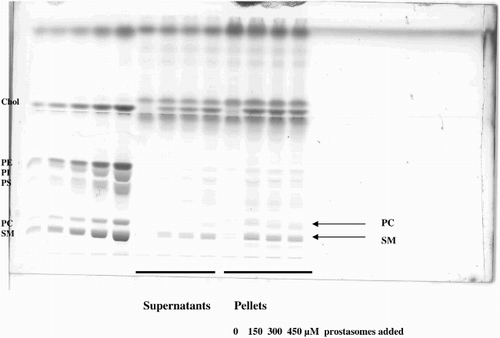
Supplemental Figure 2. Identification of lipid subclasses by bi-dimensional HPTLC. The identification of each spot corresponding to the different sub-classes of lipids was confirmed by bi-dimensional analysis.
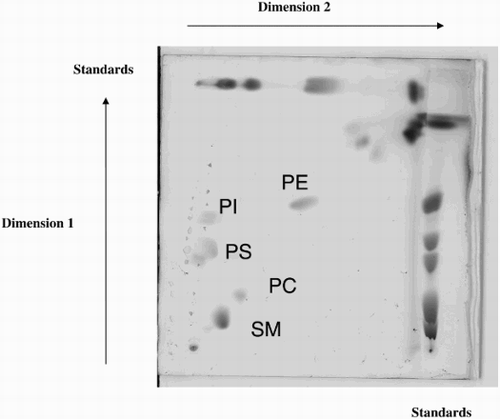
Supplemental Figure 3. Lipid analysis of different fractions in the absence or presence of lipid standards. A new HPTLC plate on which different samples were spotted, is presented in order to explain the non alignment of the lipid classes of the samples with the standards in Figure 4b which is due to the fact that the standards used in our experiments were from various sources. The fatty acid composition (molecular species) of the subclasses (PC = Phosphatidylcholin; PE = Phosphatidylethanolamin; SM = Sphingomyelin; PI = Phosphatidylinositol; PS = Phosphatidylserin) of phospholipids of the standards was different from the composition of the subclasses of phospholipids extracted from human spermatozoa which explains the shift observed for some of the bands. Lanes 2, 4, 6, and 8 correspond to supernatant 100,000g; seminal plasma 10,000g; spermatozoa, and prostasomes. Lane 1 corresponds to the standards alone. Lanes 3, 5, 7, and 9 correspond to the same samples as the ones plated as lanes 2, 4, 6, and 8 with the difference that in each of them standards were added. When comparing lane 2 with lane 3, lane 4 with lane 5, lane 6 with lane 7, and lane 8 with lane 9, we can see that the bands corresponding to SM and PC are not necessarily at the same level and that a shift could be observed for some of the samples. This discrepancy is due to the difference of molecular species of phospholipids subclasses from standards versus samples.
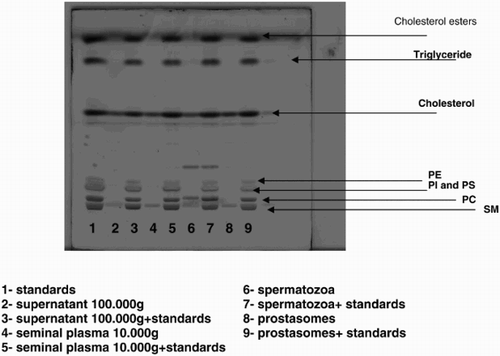