Figures & data
Figure 1. Core domain structure of DEAD-box proteins. The top panel shows a stereoview of Mss116 bound to AMP-PNP and ssRNA (U10).Citation31 Motifs that interact with ATP are orange and motifs that interact with ssRNA are green. Motif III, which does not contact ATP or ssRNA but is involved with communication between the two domains and with substrates, is olive. DI is dark gray, DII is silver, the linker between them is dark blue and the CTE, present in Mss116 and CYT-19, is light blue. Below the structure is a cylinder representation showing the domains and motifs in the same colors. Also shown are the N-terminal extension (NTE) and the C-tail of Mss116, which were not present or not resolved in the crystal structures (refs. Citation31 and Citation41).
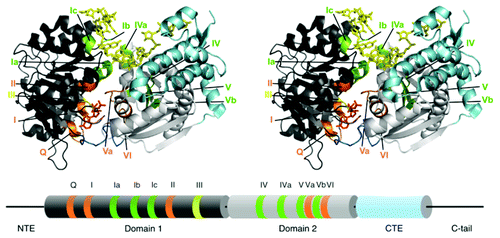
Figure 2. RNA affinity changes resulting from changes in cooperativity during the ATPase cycle. In the absence of nucleotide (No nt), cooperativity is absent by definition. Cooperativity is typically observed in the ATP-bound state and is maximal in the ADP-Pi state, as shown by the thickening blue stripe. Anti-cooperativity in the ADP-bound state is shown by the gray stripe. Specific values of cooperativity for Mss116 are indicated for each state.Citation56 The colors for the nucleotide states match those in .
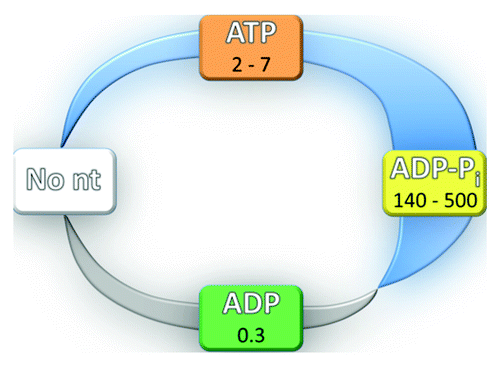
Figure 3. ATPase kinetics of DEAD box proteins. Specific rate and equilibrium constants are shown for Mss116 (data from ref. Citation56) The domain structures shown are based on SAXS studies of the open and closed complexes.Citation57 Nucleotides are abbreviated by T (ATP), D-Pi (ADP and Pi) and D (ADP). The ADP-Pi state is highlighted in red to indicate that it is populated to the greatest extent in the steady-state with saturating ATP and RNA.
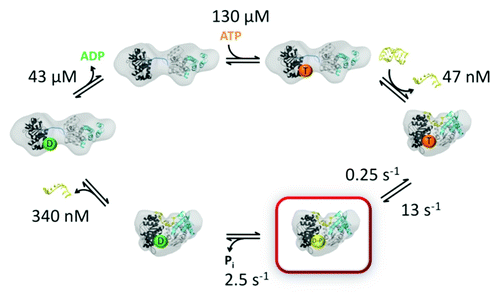
Figure 4. RNA interactions with regions of DEAD-box proteins outside the helicase core. (A) The C-tails of Mss116 and CYT-19 are unstructured in solution and are flexible, as revealed by SAXS experiments,Citation57 and are able to move relative to the core across a wide region of space. (B) Interactions of Mss116 and CYT-19 with structured RNA. The high flexibility of the C-tail is suggested to allow it to contact structured RNA and to tether the helicase core in proximity to structured RNA, while allowing the core to move to sample different regions of the RNA. The figure shows a model of the Tetrahymena group I intronCitation150 and a model from the solution and crystal structures of Mss116.Citation57 (C) A tethering interaction is formed by the C-terminal domain of DbpA/YxiN with a specific hairpin structure of a 23S rRNA precursor. Panels A and B are reprinted from ref. Citation57 and panel C is reprinted from ref. Citation44 (Copyright 2010), with permission from Elsevier.
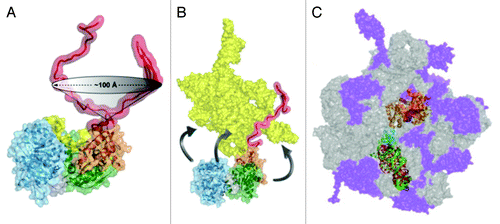
Figure 5. Model for general chaperone activity of DEAD-box proteins. The model is based on refolding of the Tetrahymena group I intron ribozyme by CYT-19. ATP-dependent unfolding of misfolded conformers allows the RNA additional chances to fold to the native state (green). Although the unfolding process mediated by CYT-19 is inherently non-specific, the native state of the RNA can be unfolded with much lower efficiency than misfolded states if it is more stable. The native and misfolded structures of the Tetrahymena ribozyme are shown as identical to emphasize the extensive native structure in the misfolded form of this RNA and because the specific structural difference is not known. A plausible model for the unfolding process is shown, in which CYT-19 first binds to the ribozyme non-specifically by interacting with the C-tail, and then the helicase core engages and unwinds a duplex in an ATP-dependent reaction, which also requires or results in disruption of a tertiary contact. The sequestration and local unwinding of this helix then fosters additional losses of structure, which may propagate along the unwound helix or through space, as shown, by the loss of tertiary structure. Although this model is consistent with the known properties of CYT-19 and the refolding process of this RNA, the specific pathways and mechanisms of unfolding are not known.
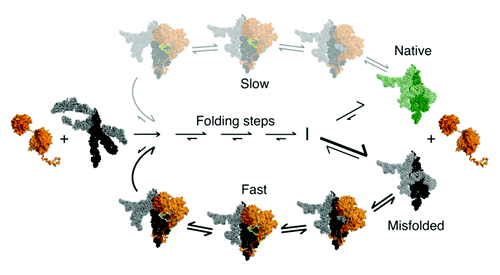