Abstract
Aspartame is a non-nutritive sweetener that is used predominantly in various ‘diet’ and ‘low-calorie’ products, such as beverages, instant breakfasts, desserts, breath mints, sugar-free chewing gum, vitamins, and pharmaceuticals, consumed by millions of people who are attempting weight loss, young adults and diabetic persons. On a weight basis, the metabolism of aspartame generates approximately 50% phenylalanine, 40% aspartic acid and 10% methanol. The detailed mechanisms of the effects of aspartame on the electrophysiological response are still unclear; therefore, this study was designed to clarify whether longer-term aspartame consumption has any effect on the electrophysiological response in Wistar albino rats. The oral administration of aspartame in a safe dose of 40 mg/kg bodyweight/day (as recommended by EFSA, 2012) was tested in Wistar albino rats for a longer period (90 days). Electrophysiological responses, including heart rate variability (HRV) and electroencephalogram (EEG) pattern, were assessed in a folate-deficient animal model along with control animals using BIOPAC and EEG equipment (model RMS EEG–24 brain new-plus: RMS – Recorder and Medicare systems). In this study, the folate-deficient animal model was used to mimic human methanol metabolism in rats. After 90 days of aspartame treatment, a significant alteration was observable in the time domain [Mean RR (ms) SDNN (ms) RMSSD (ms) PNN50 (%)] and the frequency domain [LF, HF, and LF/HF ratio] with significantly impaired frequency and amplitude of the fronto-parietal and occipital EEG waves at p ≤ 0.05. The results of this study clearly indicate that the oral consumption of aspartame reduced HRV, with sympathetic dominance and loss of vagal tone, and altered sympathovagal activity along with impairment of learning and memory, showing an additional effect on health within this study duration. The aspartame metabolites methanol and formaldehyde may be the causative factors behind the change observed.
Keywords:
1 Introduction
Cardiovascular diseases remain the principal cause of death in both developed and developing countries, accounting for approximately 20% of all deaths worldwide annually. Aspartame (anon-nutritive sweetener) is added to a variety of foodstuffs, offering an alternative sweetening choice to diabetics, dieters, and others who must limit sugar intake. It is consumed by millions of people in numerous products such as beverages, instant breakfasts, desserts, breath mints, sugar-free chewing gum, vitamins, and pharmaceuticals [Citation1]. Aspartame is completely metabolized in the gut and absorbed as the two amino acids aspartic acid (40%) and phenylalanine (50%) along with methanol (10%) [Citation2]. After aspartame consumption, the concentrations of its metabolites are increased in the blood [Citation3]. Aspartame is unstable under conditions of prolonged heating and is inappropriate for use in cooking and baking [Citation4]. Aspartame also decomposes in liquids during prolonged storage. It is known that the rat constitutes an important model for cardio-vascular physiologic research, and ECG-based studies have long been conducted in this animal model. Cardiac activation is an electrical propagation that results in a measurable change in potential difference on the surface of the body of the subject. The resulting amplified and filtered electrical signal is the electrocardiogram and is widely used to measure the heart rate and heart rate variability [Citation5]. The baseline variability of the heart rate (beat to beat interval) is determined on many scales; on the shortest scale, the time between each heartbeat is irregular. These short-term oscillations reflect changes in the sympathovagal balance [Citation5]. Our hypothesis was that methanol, a metabolite of aspartame, might cause oxidative stress in the cardiovascular centres in the brain stem and/or sympathetic centres in the hypothalamus [Citation6]. These mechanisms may affect cardiac function in experimental animals. The experimental and epidemiological data currently available to evaluate the above toxigenic risks of aspartame are insufficient and often unreliable due to the inadequate planning and conduct of previous experiments. Recently, based on previous studies, the ability of aspartame and its metabolites to alter the oxidative status of the cells via reactive oxygen species (ROS) generation and the modulation of intracellular antioxidant enzyme levels were investigated. Oral aspartame consumption at 75 mg/(kg bw/day) has been found to cause oxidative stress in the brain and liver [Citation6,Citation7], and oral aspartame consumption at 40 mg/(kg bw/day) has been found to cause oxidative stress in the brain [Citation8], liver and kidney [Citation9], and immune organs [Citation10] and to alter immune response [Citation11]. The inadequacy of past studies, combined with the general limited knowledge about the safety/potential toxigenic effects of substances widely present in the industrialized diet, motivated the design of this experiment.
Heart rate variability (HRV) [Citation12] is considered to be a non-invasive measure of autonomic nervous system activity. Despite the importance of HRV as a reproducible marker of sympathetic–parasympathetic balance, the studies related to HRV in aspartame-treated animal are very few. Little is known about the effects of aspartame on electrophysiological response (HRV and EEG). The detailed mechanisms of the effects of aspartame remain unclear; the purpose of the current study is to clarify whether longer-term aspartame consumption has any effect on the electrophysiological response of Wistar albino rats.
2 Material and method
2.1 Chemicals
Pure aspartame powder and methotrexate was purchased from Sigma–Aldrich chemical, St. Louis, USA, and all other chemicals used were of analytical grade and obtained from Sisco Research Laboratory, Mumbai, India.
2.2 Animal model
Animal experiments were conducted after obtaining clearance from the Institutional Animal Ethical Committee (IAEC No: 01/21/2014) and the Committee for the Purpose of Control and Supervision of Experiments on Animals (CPCSEA). The animal studies were approved by the appropriate ethics committee and have therefore been performed in accordance with the ethical standards laid down in the 1964 Declaration of Helsinki and its later amendments. It should also be stated clearly in the text that all persons gave their informed consent prior to their inclusion in the study. The experimental animals were healthy, inbred adult male Wistar albino rats, weighing approximately 200–220 g. The animals were maintained under standard laboratory conditions and were allowed food and water ad libitum (standard rat feed pellets supplied by M/s. Hindustan Lever Ltd., India). Animals of the aspartame-treated groups were daily administered aspartame (40 mg/kg bw/day) [Citation13,Citation14] dissolved in normal saline orally (by means of gavage needle) for 90 days. All of the rats were housed under conditions of controlled temperature (26 ± 2 °C) with 12-h light and 12-h dark exposure.
2.3 Folate-deficient model and treatment
Animals were randomly divided into four groups, each consisting of six animals. Group 1 included the control animals, which were administered normal saline orally (by means of gavage needle) throughout the experimental protocol. Group 3 consisted of control animals treated with aspartame orally for 90 days (40 mg/kg bw/day). In this study, animals fed a folate-deficient diet were used to mimic the human methanol metabolism, as human beings have very low hepatic folate content [Citation15]. In the methanol metabolism, the conversion of formate to carbon dioxide is folate dependent. Hence, in the case of a deficiency of folic acid, the methanol metabolism can take an alternate pathway (the microsomal pathway) [Citation16]. To simulate this process, rats were made folate deficient by feeding them a special dietary regime for 37 days, after which methotrexate (MTX) in sterile saline was administered every other day for two weeks [Citation17] before euthanasia. MTX folate deficiency was confirmed by estimating the urinary excretion of formaminoglutamic acid (FIGLU) [Citation18] prior to the experiment. Rats on a folate-deficient diet excreted an average of 90–100 mg FIGLU/kg body weight/day (range 25–125), while animals on the control diet excreted an average of 0.29 mg/kg body weight/day (range 0.15–0.55). These folate-deficient animals showed a significant increase in FIGLU excretion compared to the control animals (P < 0.05). The folate-deficient animals were further divided into two groups. Group 2 consisted of animals fed a folate-deficient, while Group 4 consisted of animals fed a folate-deficient diet and treated with aspartame orally for 90 days (40 mg/kg bw/day).
2.4 Experimental Groups
Group 1 Control animals.
Group 2 Folate-deficient diet fed animals.
Group 3 Control animals treated with aspartame (40 mg/kg bw) orally for 90 days.
Group 4 Folate-deficient diet fed animals treated with aspartame (40 mg/kg bw) orally for 90 days.
2.5 Experimental procedure
2.5.1 Measurement of heart rate variability (HRV)
Different approaches based on spectral and time domain analysis were used to evaluate the cardiac rhythm regulatory response to aspartame. HRV monitoring using the radio-telemetric system has been described previously [Citation19,Citation20]. The time intervals between adjusted R waves in the electrocardiography channel (RR) were obtained on a beat-to-beat basis using the Dataquest A.R.T. analysis software (version 2.20; Data Sciences International, USA). Errors resulting from the R-wave detector were eliminated using the following criteria to obtain normal-to-normal (NN) intervals. The most commonly used approaches to the analysis of heart rate variability are time domain (TD) and frequency domain (FD) analyses [Citation21]. In time domain analysis, the intervals between normal N-N waves are measured over the period of the recording. The NN intervals are used to determine the SD of the NN intervals (SDNN) and the root mean square of successive differences of adjacent NN intervals (RMSSD). Simple descriptive statistics are used to obtain measures of HRV in this method. Frequency domain methods are expressed in the form of a spectral density function that depicts spectral power as a function of frequency [Citation22]. High frequency is solely mediated by changing levels of vagal activity [Citation23,Citation24], whereas low frequency is mediated by both sympathetic and vagal activity.
2.5.2 Electroencephalogram (EEG) measurement
Electrodes were implanted in all of the animals after the last session of aspartame treatment. In brief, animals were anesthetized with ketamine/xylazine (90/15 mg/kg, i.p.). The toe pinch method was used once every 5 min to check the responsiveness after the administration of anaesthetic agent, after which the animal was fixed in a stereotaxic frame. After aseptic cleaning procedures, a small incision was made in the skin and a recording electrode fixed on the surface of the scalp. The electrode was placed on the scalp and glued with dental acrylic resin, and the incision was closed and sutured, exposing the electrode. Post-operative care was performed according to ethical guidelines with the administration of saline and to prevent fluid loss during incision and of antibiotic to prevent sepsis. After recovery, the electrodes were connected to the EEG equipment (using model RMS EEG–24 brain new-plus: RMS – Recorder and Medicare systems). The EEG activity was recorded for approximately 10 min in anesthetized rats. Signals were filtered between 1 and 70 Hz. Recordings were analyzed by RMS EEG–24 Super sec version 1.1 and expressed in terms of amplitude (μv) and frequency (Hz). All experiments were performed at the same time of day (10:00–12:00 am) to eliminate variation due to circadian rhythms.
2.6 Statistical analysis
Data are expressed as the mean ± standard deviation (SD). All data were analyzed using the SPSS for Windows statistical package (version 20.0, SPSS Institute Inc., Cary, North Carolina. The statistical significance among the four different groups was analyzed using the one-way ANOVA test followed by Tukey's multiple comparison tests, and the significance level was fixed at p < 0.05.
3 Results
3.1 Effect of aspartame on mean RR interval
The results are summarized in () as the mean ± SD. The RR interval was not significantly altered in folate-deficient animals compared to control animals. However, both control and folate-deficient animals treated with aspartame for 90 days showed a significant increase in RR interval compared to control and folate-deficient animals not treated with aspartame.
Table 1 Aspartame on heart rate variability (HRV).
3.2 Effect of aspartame on frequency domain analysis
The data are summarized in () as the mean ±SD. The total spectral power, lower frequency, higher frequency and LF/HF ratio of folate-deficient animals were similar to the control animals. However, both the control and the folate-deficient animals treated with aspartame for 90 days showed significant decreases in total spectral power and higher frequency and significant increases in lower frequency and LF/HF ratio compared to controls and folate-deficient animals not treated with aspartame.
3.3 Effect of aspartame on time domain analysis
The results are summarized in () as the mean ± SD. The standard deviation of the NN intervals (SDNN), the square root of the mean squared differences of successive NN intervals (RMSSD), and the proportion derived by dividing NN50 by the total number of NN intervals (PNN50) of folate-deficient animals were similar to the corresponding values for the control animals. However, both control and folate-deficient animals treated with aspartame for 90 days showed significant decreases in SDNN, RMSSD, and PNN50 compared to control and folate-deficient animals not treated with aspartame.
3.4 Effect of aspartame on EEG analysis parameters that reflect learning and memory
The results are summarized in (a–f) as the mean ± SD. The amplitude and frequency of EEG waves in the fronto-temporal and occipital lobes of folate-deficient animals were similar to the control animals. However, both control and folate-deficient animals treated with aspartame for 90 days showed a significant increase in amplitude and decrease in frequency compared to control and folate-deficient animals not treated with aspartame.
Fig. 1 Effect of aspartame on EEG recording. (a)–(f) EEG waves (amplitude and frequency) of both the fronto-parietal and occipital regions. Each value represents mean ± SD. Significance at *p < 0.05, *a – compared with control animals, *b – compared with folate-deficient (FD) animals. FD: folate deficient, ASP: aspartame.
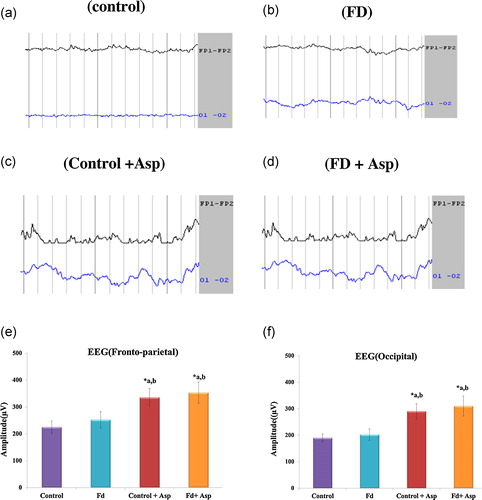
4 Discussion
Laboratory animals have been used in research on an ongoing basis, and non-invasive methods are being increasingly implemented in cardiac autonomic regulation, including heart rate variability analysis (HRV). Experimental studies on human cardiovascular function are obviously limited for safety reasons. In most animal studies, non-parametric methods (time and frequency domain) are used normally. In this study, we showed the HRV and EEG response to aspartame metabolites in aspartame-fed rats thoroughly habituated to the laboratory conditions. We developed a folate-deficient model to mimic the human methanol metabolism in rats. The time interval between heartbeats is not constant, and the variability that occurs is due to a variety of physiological processes that affect the heart rate at different frequencies. The measured tachogram of HRV is a reflection of all of the different interacting phenomena. The PSA of HRV divides the overall variability into separate frequencies and thereby enables the identification of the activity of the various affecting components [Citation25]. This non-invasive technique has attracted considerable interest in both cardiology [Citation26] and neurology [Citation27]. Time- and frequency-domain HRV parameters were obtained and analyzed simultaneously. SDNN and RMSSD are time-domain indices of HRV, reflecting parasympathetic modulation of the RR interval. In this study, these indices were reduced in the aspartame-treated animals compared to the untreated animals. The reduction of SDNN, RMSSD and PNN50 in aspartame-treated animals clearly shows that cardiac parasympathetic modulation is reduced in these animals. Heart rate variability (HRV) has attracted considerable interest as a non-invasive index of frequency-specific modulation of the SA node by the autonomic nervous system. LF power is contributed by changes in sympathetic and parasympathetic activity to the heart [Citation26]. LF quantifies the baroreflex modulation of RR intervals, and HF quantifies baroreflex-mediated changes in RR intervals at the respiratory frequency [Citation24]. This study showed that LF was significantly increased, HF was reduced, and the LF/HF ratio was significantly decreased in aspartame-treated animals. Total power (TP), an index of overall HRV, was also reduced in our aspartame-treated animals. An increase in sympathetic activity can also lead to the formation of oxidative by-products [Citation28]. Oxidative stress results from an oxidant–antioxidant imbalance [Citation29] and plays a role in the pathogenesis of many diseases [Citation30]. Reactive oxygen species (ROS) play a role in elevating central and peripheral sympathetic activity by increasing nitric oxide production [Citation31]. Our previous study showed that aspartame acts as a chemical stressor, alters the oxidative–antioxidative balance [Citation10,Citation11], increases oxidative stress, and depresses HRV [Citation32]. When a person is exposed to a stressor, the autonomic nervous (ANS) system is triggered: the parasympathetic nervous system is suppressed, and the sympathetic nervous system is activated [Citation24]. This process results in the secretion of the hormones epinephrine and norepinephrine into the bloodstream and changes in heart rate (HR) and heart rate variability (HRV) [Citation33]. An increase in HR, generally considered to result from a shift of the sympathovagal balance towards the sympathetic nervous system, was associated with a modest increase in the LF/HF ratio, which, on the contrary, suggests sympathetic gain. Tight links seem to exist between time- and frequency-domain HRV measures, as suggested by the high association between RMSSD and HF-spectral power or SDNN and TP, indicating the consistency of the obtained data. In rats, sympathetic efferents are dominant regulatory components that control the heart rate [Citation34]. Their HRV resembles its human counterpart, with two principal components: the LF and HF [Citation35]. Reduced HRV is an independent indicator of adverse prognosis in cardiac disorder [Citation36,Citation37].
Normal thinking and decision making with higher cognitive abilities are mainly associated with the prefrontal cortex [Citation38] and involve the activation and transmission of signals in the neurons of the prefrontal cortex. They also have connections with other regions of the brain that regulate cognitive, emotion and memory functions. In addition to the prefrontal cortex, the parietal and occipital cortices also play a role by transferring the highly processed visuospatial information and oculomotor attention guidance to the dorsolateral prefrontal cortex [Citation39]. Furthermore, in addition to visual functions, the occipital cortex is involved in non-visual functions such as spatial recognition memory [Citation40]. In particular, the medial extrastriate visual cortex is involved in mediating the memory of visual object information [Citation41]. Hence, all of the information and signals are conducted to the frontal cortex for further processing and analysis by different pathways and connections. The activity of these brain areas can be recorded cortically by EEG [Citation42]. The occipital cortex (medial extrastriate visual cortex) is involved in visual recognition memory [Citation43]. In this study, EEG waves (amplitude and frequency) were altered in both the fronto-parietal and occipital region in aspartame-treated animals, which might affect learning and memory in rats.
Aspartame has previously been reported to affect the brain through the elevated release of ROS and RNS [Citation6,Citation44]. It is postulated that the consumption of aspartame might potentially perturb the amount of biogenic amines in brain via the aspartame-induced increase in phenylalanine and tyrosine [Citation45]. Na+/K+-ATPase is inhibited by oxygen reactive substances, as demonstrated in the brain [Citation46]. The administration of aspartame alone or, in the presence of mild systemic inflammatory response, the intraperitoneal (i.p.) administration of lipopolysaccharide increases oxidative stress and inflammation in the brain but not in the liver [Citation47]. Yokogoshi and Wurtman [Citation48] reported that brain norepinephrine levels were increased in rats 2 h after gavage with a single dose of aspartame (200 mg/kg bw) compared with saline controls. This current finding on aspartame is also consistent with previous reports.
5 Conclusion
The results showed that heart rate variability was markedly decreased in aspartame-treated rats. Our previous study showed that aspartame acts as a chemical stressor and impairs the oxidative–antioxidative balance, which may be associated with the reduced HRV, sympathetic dominance and loss of vagal tone appearing in this study. The loss of protective vagal tone would explain the increased vulnerability to cardiovascular disease. An increasing number of physical illnesses also appear to be associated with sympathetic dominance, reduced vagal tone and reduced HRV. Aspartame metabolites also showed impairment of learning and memory by alteration of EEG (amplitude and frequency) waves in both the fronto-parietal and occipital regions, which may affect learning and memory in rats. This result has also been documented in this study. The aspartame metabolites methanol and/or formaldehyde might be the causative factors behind the changes observed.
6 Limitations of the study
We were unable to calculate baroreflex sensitivity and blood pressure variability, as we lacked a beat-to-beat BP monitor.
Conflict of interest
The authors declare no potential conflicts of interest with respect to the authorship and/or publication of this article.
Acknowledgements
The authors gratefully acknowledge the University of Madras for their financial support [UGC No.D.1. (C)/TE/2012/1868].
Notes
Peer review under responsibility of Taibah University.
References
- R.AllevaB.BorghiL.SantarelliE.StrafellaD.CarbonariM.BracciM.TomasettiIn vitro effect of aspartame in angiogenesis inductionToxicol. In Vitro252011286293
- R.E.RanneyJ.A.OppermanE.MuldoonF.G.McMahonComparative metabolism of aspartame in experimental animals and humansJ. Toxicol. Environ. Health21976441451
- L.D.SteginkThe aspartame story: a model for the clinical testing of a food additiveAm. J. Clin. Nutr.461987204215
- M.KrogerK.MeisterR.KavaLow-calorie sweeteners and other sugar substitutes: a review of the safety issuesCompr. Rev. Food Sci. Food Saf.520063547
- D.Azuaje FranciscoE.Clifford GariP.McsharryAdvanced Methods and Tools for ECG Data Analysis2007Artech House1385
- I.AshokR.SheeladeviEffect of chronic exposure to aspartame on oxidative stress in the brain of albino ratsJ. Biosci.372012679688
- I.AshokR.SheeladeviD.WankharW.WankharLong-term effect of aspartame on the liver antioxidant status and histopathology in Wistar albino ratsBiomed. Prev. Nut.201310.1016/j.bionut2013.10.002
- M.M.ImanA.N.NaveenAspartame (a widely used artificial sweetener) and oxidative stress in cerebral cortexInt J. Pharm. Biomed. Sci.22011410
- M.M.ImanEffect of aspartame on some oxidative stress parameter in liver and kidney of ratsAfr. J. Pharm. Pharmacol.52011678682
- K.C.ArbindR.SheeladeviImbalance of oxidant–antioxidant status by aspartame in the organs of immune system of Wistar albino ratsAfr. J. Pharm. Pharmacol.7201430193025
- K.C.ArbindR.Sheela DeviL.SundareswaranRole of antioxidant enzymes in oxidative stress and immune response evaluation of aspartame in blood cells of Wistar albino ratsI.F.R.J.21201522632272
- D.R.BrownL.V.BrownA.PatwardhanD.C.RandallSympathetic activity and blood pressure are tightly coupled at 0.4 Hz in conscious ratsAm. J. Physiol.267199413781384
- European Food Safety Authority (EFSA)Opinion of the Scientific Panel on Food Additives, Flavourings, Processing Aids and Materials in contact with Food (AFC) on a request from the Commission related to a new long-term carcinogenicity study on aspartameEFSA J.3562006144
- Food and Drug Administration (FDA)Food additives permitted for direct addition to food for human consumption: aspartameFed. Regist.6119963365433656
- A.B.MakerT.R.TephlyMethanol in poisoning folate deficient ratsNature2611976715716
- T.R.TephlyThe toxicity of methanolLife Sci.48199110311041
- S.M.HwangC.A.MeadowsS.W.ThenenMethotrexate effects on folate status and the deoxyuridine suppression test in ratsNutr. Res.91989431444
- J.C.RabinowitzW.E.PricerFormiminotetrahydrofolic acid and methenyltetrahydrofolic acid as intermediates in the formation of N10-formyltetrahydrofolic acidJ. Am. Chem. Soc.78195657025704
- C.C.ChangJ.S.HwangC.C.ChanP.Y.WangT.H.HuT.J.ChengEffects of concentrated ambient particles on heart rate variability in spontaneously hypertensive ratsJ. Occup. Health472005471480
- C.C.ChangJ.S.HwangC.C.ChanT.J.ChengInteraction effects of ultrafine carbon black with iron and nickel on heart rate variability in spontaneously hypertensive ratsEnviron. Health Perspect.115200710121017
- E.L.FallenHidden rhythms in heart rate record: a primer in neurocardiologyClin. Invest. Med.232000339346
- G.G.BerntsonJ.T.BiggerD.L.EckbergP.GrossmanP.G.KaufmannM.MalikH.N.NagarajaS.W.PorgesJ.P.SaulP.H.StoneM.W.Van Der MolenHeart rate variability: origin, methods andinterpretive caveatsPsychophysiology341997623648
- B.PomeranzR.J.B.MacaulayM.A.CaudillI.KutzD.AdamD.GordonK.M.KilbornA.C.BargerD.C.ShannonR.J.CohenH.BensonAssessment of autonomic function in humans by heart rate spectral analysisAm. J. Physiol. Heart. Circ. Physiol.2481985151153
- S.AkselrodD.GordonF.A.UbelD.C.ShannonA.C.BargerR.J.CohenPower spectral analysis of heart rate fluctuation: a quantitative probe of beat-to-beat cardiovascular controlScience2131981220222
- M.MalikHeart rate variability: standards of measurement, physiological interpretation and clinical use, Task Force of the European Society of Cardiology and the North American Society of Pacing and ElectrophysiologyCirculation93199610431065
- M.MalikA.J.CammComponents of heart rate variability: what they really mean and what they really measuresAm. J. Cardiol.721993821822
- J.S.DonchinA.ConstantiniE.A.SzoldS.ByrneW.PorgesCardiac vagal tone predicts outcome in neurosurgical patientsCrit. Care Med.201992942949
- K.F.HarrisK.A.MathewInteractions between autonomic nervous system activity and endothelial function: a model for the development of cardiovascular diseasePsychosom. Med.662004153164
- I.Dalle-DonneR.RossiR.ColomboD.GiustariniA.MilzaniBiomarkers of oxidative damagein human diseaseClin. Chem.522006601623
- H.CaiD.G.HarrisonEndothelial dysfunction in cardiovascular disease: the role of oxidative stressCirc. Res.872000840844
- V.M.CampeseS.YeH.ZhongV.YanamadalaZ.YeJ.ChiuReactive oxygen species stimulatescentral and peripheral sympathetic nervous activityAm. J. Physiol. Heart Circ. Physiol.2862004695703
- N.ChattipakornT.InthachaiS.LekawanvijitS.ChattipakornDipeptidyl peptidase-4 inhibitor attenuates cardiac dysfunction and adverse remodeling after myocardial infarctionJ.A.C.C.65201511821191
- J.TaelmanS.VandeputA.SpaepenS.Van HuffelInfluence of mental stress on heart rate and heart rate variabilityIFMBE22200813661369
- P.MansierJ.ClairambaultN.CharlotteC.MédigueC.VermeirenG.LePapeLinear and non-linear analyses of heart rate variability: a mini reviewCardiovasc. Res.311996371379
- J.AltimarasUnderstanding autonomic sympathovagal balance from short-term heart rate variations. Are we analyzing noiseComp. Biochem. Physiol. A. Mol. Integr. Physiol.1241999447460
- M.T.La RovereJ.T.BiggerF.I.MarcusJr.A.MortaraP.J.SchwartzBaroreflex sensitivity and heart-rate variability in prediction of total cardiac mortality after myocardial infarctionLancet3511998478484
- J.NolanP.D.BatinR.AndrewsS.J.LindsayP.BrooksbyM.MullenProspective study of heart rate variability and mortality in chronic heart failure: results of the United Kingdom Heart Failure Evaluation and Assessment of Risk TrialCirculation98199815101516
- A.F.ArnstenStress signaling pathways that impair prefrontal cortex structure and functionNat. Rev. Neurosci.102009410422
- H.BarbasConnections underlying the synthesis of cognition, memory, and emotion in primate prefrontal corticesBrain Res. Bull.522000319330
- A.D.AlfonsoOn the Neuroplasticity of the Occipital Cortex in both the Congenitally and Early Blind and Possible Implications for Rehabilitation2010Princeton University
- R.P.KesnerA.RavindranathanP.JacksonR.GilesA.A.ChibaA neural circuit analysis of visual recognition memory: role of perirhinal, medial and lateral entorhinal cortexLearn. Mem.820018795
- M.Hernández-GonzálezM.A.GuevaraM.CervantesG.MoraliM.Corsi-CabreraCharacteristic frequency bands of the cortico-frontal EEG during the sexual interaction of the male rat as a result of factorial analysisJ. Physiol. (Lond.)9219984350
- R.P.KesnerA.RavindranathanP.JacksonR.GilesA.A.ChibaA neural circuit analysis of visual recognition memory: role of perirhinal, medial, and lateral entorhinal cortexLearn. Mem.820018795
- M.V.AbhilashV.Sauganth PaulR.MathewsN.VargheseHarikumaranLong-term consumption of aspartame and brain antioxidant defense statusDrug Chem. Toxicol.36201316
- R.J.WurtmanT.J.MaherEffects of oral aspartame on plasma phenylalanine in humans and experimental rodentsJ. Neural Transm.701987169173
- P.KalpanM.MatejovicovaV.MezesovaIron induced inhibition of Na+/K+ -ATPase and Na+/Ca2+ exchanger in synaptosomes: protection by the ptridoindole stobadineNeurochem. Res.22199715231529
- O.M.EAbdel-SalamN.A.SalemJ.HusseinEffect of aspartame on oxidative stress and monoamine neurotransmitter levels in lipopolysaccharide-treated miceNeurotox. Res.212012245255
- H.YokogoshiR.J.WurtmanAcute effects of oral or parenteral aspartame on catecholamine metabolism in various regions of rat brainJ. Nutr.1161986356364