ABSTRACT
1. Due to the increasing global demand for more sustainably produced animal protein, there is an intensive search for feeds to replace soybeans. Black soldier fly larvae (BSFL) appear to have great potential for replacing soybeans in poultry diets. The main objective of this study was to determine if the nutritional value of BSFL is superior to soybeans when feeding organic broilers, since smaller amounts of BSFL could replace the soybean content in the feed, thus saving even more resources.
2. Eighty Hubbard S757, a slow growing organic broiler type, were fattened for 63 d, spending the last 49 d on one of five diets. Two soybean cake- and soybean oil-based diets (SS, SS-) were compared with three diets based on partially defatted BSFL meal and BSFL fat from two origins (AA-, AB-, BB-). Different from diet SS, diets SS-, AA-, AB-and BB- were designed with approximately 20% less lysine and methionine. Growth (n = 16), metabolisability, body nitrogen retention, carcase and meat quality (n = 8) were evaluated.
3. Broilers of the insect-based feeding groups, AA- and AB-, grew similarly well compared to those of group SS. They also retained more nitrogen in the body than those fed BB- and SS-. Breast meat yield was higher with AA- and AB- than with BB- and SS-, but still lower than with SS. Dietary variations in physicochemical meat quality were of low practical relevance. Diet BB- resulted in a more yellow skin and meat. The fatty acid profile of the breast meat lipids reflected the high lauric acid proportion of the BSFL lipids, resulting in up to 80 times higher proportions than when feeding the soybean-based diets.
4. The results indicate that high-quality BSFL, depending on their origin, may indeed be superior to soybean protein, but that the meat lipids from BSFL-fed broilers can contain significant amounts of lauric acid, which, from a human nutrition perspective, could have a negative impact on meat quality.
Introduction
Poultry meat is a commodity with increasing global importance, and soybeans are a main protein source for the poultry’s consumption. Also, soybean oil is used in poultry diets. Cultivating soybeans primarily takes place in subtropical regions and is co-responsible for ongoing deforestation (Taherzadeh and Caro Citation2019). In addition, the same authors point out that the high amounts of fertiliser and water required for its cultivation lead to further environmental impacts. The search for alternatives that are safe for human consumption and can be produced more ecologically while remaining economically competitive has intensified in recent years. One such solution may be insects. Specifically, the black soldier fly larvae (BSFL, Hermetia illucens L.), a globally prevalent stratiomyidae (Diptera) presents a promising alternative protein source (Smetana et al. Citation2019; van Huis Citation2020). These larvae can convert a wide range of organic materials including animal manure, kitchen waste and agricultural waste into a high-quality protein source (Ewusie et al. Citation2018; Gold et al. Citation2020). Recently, the EU has admitted protein meals from certain insect species, among them BSFL, as feeds for poultry (Commission Regulation (EU) 2021/1372 from 17 August 2021). This EU regulation also prescribes that rearing substrates are restricted to materials that could be used directly as food or feed.
Research related to BSFL in poultry nutrition has steadily increasing (Abd EL-Hack et al. Citation2020; Sypniewski et al. Citation2020). The BSFL meal is rich in N (up to about 100 g/kg dry matter (DM)) and comparably rich in lysine (Lys) and methionine (Met) (Heuel et al. Citation2021a). Moreover, unprocessed BSFL are rich in energy (up to 400 g ether extract/kg DM) as well as Ca and P (Barragan-Fonseca et al. Citation2017). However, the focus was placed on feeding laying hens and on testing defatted BSFL meal. Recently, research has included feeding BSFL to broilers, whether as a full-fat meal (Pieterse et al. Citation2019; Woods et al. Citation2019; de Souza Vilela et al. Citation2021) or as fully or partially defatted BSFL meal (Dabbou et al. Citation2018; Schiavone et al. Citation2019). Additionally, the substitution of soybean oil by pure BSFL fat was tested (Schiavone et al. Citation2018; Cullere et al. Citation2019; Kim et al. Citation2020), as was the use of live BSFL in broiler diets, where an increased activity promoted the birds’ welfare (Ipema et al. Citation2020).
These studies did not find any adverse effects on growth performance and feed conversion rate. However, the diets used in these studies were always designed to fully cover the needs of the used broiler types and usually replaced soybean material one-to-one. This excluded determining whether BSFL-based feeds were even superior to soybean in their nutritional quality. If so, it would be possible to replace the soybean with lower proportions of BSFL. The quality of the BSFL material could also depend on the quality of its production and processing (Heuel et al. Citation2021a). Furthermore, the use of BSFL in poultry nutrition, especially full-fat BSFL, might be limited by the richness of its lipids in the undesired lauric acid (12:0, LAU) (Ulbricht and Southgate Citation1991). However, laying hens were shown to incorporate only 0.7–0.8% of LAU intake in the egg lipids (Heuel et al. Citation2021b). This might not be the case in broiler meat lipids. Schiavone et al. (Citation2019) found a clear increase in LAU, myristic acid (14:0, MYR) and palmitic acid (16:0, PAM) in the meat lipids compared to the soybean-based feeding, even when using defatted BSFL meal.
For these reasons, the present study’s objective was to test the feeding value of BSFL meal and the fat of different production and processing origins in diets designed for organic broilers. Three hypotheses were tested: (1) The nutritional value of defatted BSFL meal is superior to that of soybeans for broilers and, consequently, lower amounts are needed compared to soybeans: (2) replacing soybeans with BSFL material improves the growth performance of organic broilers and does not impair their carcase and meat quality; and (3) the high content of LAU and other medium-chain fatty acids (FAs) of the BSFL lipids leads to only a small increase of these FA in the meat lipids. In order to provoke differences between BSFL and soybean protein, a lower proportion of BSFL in the diet was compared to soybean, with a calculated deficiency in Lys and Met.
Materials and methods
Ethics approval
All experimental procedures were approved by the responsible authority for animal welfare, the Cantonal Veterinary Office of Zurich, Switzerland (licence no. ZH221/17).
Birds and housing conditions
A total of 86 one-day-old organic-type broiler chicks (Hubbard S757) were purchased from Wüthrich Geflügel, Belp, Switzerland, to be fattened for 63 d following the Swiss guidelines for organic poultry production (Bio Suisse Citation2020). Six of these chicks were randomly selected and euthanised 24 h after hatching for comparative body composition analysis and stored at −20°C until analysis. The remaining 80 chicks were housed for the first 2 weeks of life in groups of 28–30 birds in three wooden compartments (1.73 m2 floor size each) and fed a commercial crumbled starter diet (UFA 671, UFA, Herzogenbuchsee, Switzerland) at ad libitum access. The boxes were equipped with a perch, fine sawdust, and a plastic drinking trough. The room temperature was continuously reduced from 33°C during the first 2 d to 28°C from day 8 onwards. Light was provided for 23 h/day in the first 2 d and for 16 h/day afterwards. Humidity was maintained at about 45%.
For the remaining 49 d, the broilers were moved in pairs to their final pens of 0.604 m2 floor size, 0.8 m height and meshed floor with a perch and nest mat. Sixteen broilers each, marked individually by rings, were assigned each of five experimental diets using a randomised block design. It was ensured that the animals in each pair were of similar weight in order to create a balance of strength and prevent rank warfare. Following the common practice for broiler fattening, the birds remained unsexed. The temperature was continuously reduced from 28°C to 22°C from day 14 to day 28 and maintained going forward. Diets (one trough per pen) and water (three nipple drinkers) were provided at ad libitum access. The health status of the broilers was visually monitored daily. One SS bird was euthanised on day 45 due to neurological damage unrelated to the experiment.
Diets and experimental design
The two control diets (SS, SS-) were based on soybean cake and soybean oil, while the other three experimental diets (AA-, AB-, BB-) were based on different combinations of partially defatted BSFL meal and fat from two origins (A, B) that varied in rearing, harvesting and processing of the BSFL material (). Larvae material A was produced at an industrial scale, where the BSFL grew on wheat bran and wheat distillery solubles (InnovaFeed, Paris, France). Larvae material B came from a small-scale experimental facility (FiBL, Frick, Switzerland) to simulate the potential and challenges of BSFL production within a smallholder farming context. For this purpose, the BSFL were grown on a substrate based on side streams and pre-consumer waste (400 g fruit and vegetable raw waste, and 300 g brewer’s grains, 300 g pasta production waste/kg). Further details of the production process and the differences between the two BSFL origins are given in Heuel et al. (Citation2021a). The control diet SS contained a typical amount of soybean-based feeds (250 g soybean cake and 30 soybean oil/kg) and was designed to meet the breeder’s requirements (Hubbard Citation2019) for Hubbard S757. These were at least 31.2 g N (195 g crude protein), 10.2 g Lys, 3.4 g Cys and 4.6 g Met per kg of dietary DM. Different from SS, the negative control diet SS- was formulated to induce a deficiency in Lys and Met (+Cys) that was similar to the three BSFL-based diets.
Table 1. Ingredient composition (g/kg DM) of the 5 different experimental diets.
All components, except for the BSFL meal and fat, the soybean oil and the maize gluten, were of certified organic origin. As the organic regulations demand (Bio Suisse Citation2020), no synthetic amino acids were supplemented. Celite was added as an indigestible marker to determine apparent digestibility and metabolisability. The feed components were mixed in a single-shaft feed mixer (100 kg volume, Gericke, Zurich, Switzerland). Afterwards, the respective proportions of soybean oil or liquefied BSFL fat (by heating up to 25°C) were added and the material was mixed again for 15 min. This material was pressed into crumbly pellets of 3 mm diameter using a pelleting machine (model RP18, Ecokraft, Deggendorf, Germany).
Measurements and sampling
The BW was measured once per week and at the end of the fattening period. Feed and water intake were measured daily per pen (n = 8 observations per diet for the statistical analysis). The excreta were collected in a tray installed below the mesh floor. Over 6 d in week 4, the excreta were removed daily per pen, weighed, frozen and combined into one composite sample per pen per week. Subsequently, these samples were frozen at −20°C and lyophilised for 48 h. Samples of the soybean-based feeds and BSFL materials were taken at the beginning of the feed mixing process, and diet samples were taken after the pelleting process. All feed items and excreta were milled to 0.5 mm (feed) or 0.75 mm (excreta) (centrifugal mill, model ZM1, Retsch, Haan, Germany).
Finally, both birds per pen fasted for 24 h, and were slaughtered by stunning with a blow to the head followed by exsanguination. One bird per pen was plucked, and its organs were removed (n = 8 per diet). The carcases were weighed and the colour of the skin on top of the breast was measured at three defined points with a chromameter (Minolta, Ramsey, NJ, USA) using the L*a*b* colour space. Subsequently, the carcases were covered with a humid cloth and stored overnight at approximately 4°C. The breast muscles were removed and weighed, and the right breast muscle was vacuum-sealed and frozen at −20°C. The left breast muscle’s colour was measured in the same way as skin colour, and pH was measured with a pH-metre (testo 205, Testo Ltd., Alton, Hampshire, UK). Afterwards, the left breast muscle was homogenised with a Moulinette type DP-800 (Ecully, France). Two portions were vacuum sealed and frozen at −20°C for further analyses.
The second bird per pen (n = 8/diet; n = 7 in group SS) was subjected to whole-body analysis. The exuding blood was collected, weighed, and frozen at −20°C. The proportion of feathers to the total body was calculated by the difference in carcase weight before and after plucking. Feather samples were taken from all feather types, frozen at −20°C, thawed and cut to 1 to 2 mm length with a scissor. The carcases were frozen at −20°C overnight and cut to strips of approximately 2 × 5 cm using a band saw (type SCF-16, 213 119, Bosshart, Amriswil, Switzerland). These strips were homogenised in a meat grinder (type HR, No. 4330–9050, Rouviere Slicing, Eclépens, Switzerland). During homogenisation, the birds’ frozen blood was added to obtain a homogeneous mass of meat and blood. Homogenates were lyophilised for 48 h and ground to 0.75 mm with the same mill as specified above. The six 1-day-old chicks were also homogenised in the meat grinder (including feathers). To generate sufficient mass, the materials from three chicks each were pooled.
Laboratory analyses
All analyses were run in duplicate. Feed items were analysed for proximate composition, gross energy (GE), chitin, P, Ca, Mg, Cl and Na, as well as amino acids by standard procedures (AOAC International Citation1997) and methods described in detail in Heuel et al. (Citation2021a). DM and total ash contents were assessed using an automatic thermogravimetric device (model TGA-701; Leco, St. Joseph, MI; AOAC Official Method 942.05). Nitrogen (N) was analysed with a C/N-Analyser (model TruMacCrude CN, Leco, St. Joseph, MI; AOAC Official Method 968.06). Data were not presented as crude protein due to uncertainties in conversion factors for insect and feather proteins. The feed items’ colour characteristics were determined with L*a*b* photometry as described for skin and meat. Excreta, feathers and whole-body homogenates were analysed for DM, N and GE using the same methods as for the feed samples.
The FA composition of the feed items were determined by applying Method 2.301 of the International Union of Pure and Applied Chemistry (IUPAC Citation1991) as Heuel et al. (Citation2021b) described in detail. An accelerated solvent extractor (model ASE 200, Dionex Corp., Sunnyvale, CA, USA) and a Hewlett-Packard gas chromatograph (model HP 6890, Wilmington, PA, USA) equipped with an FID detector, and a CP7421 column (200 m × 0.25 mm × 0.25 μm, Varian, Darmstadt, Germany) were used. Sunflower oil was used to calculate the response factor.
In the homogenised breast muscle samples (n = 8/diet), moisture, N, intramuscular fat (IMF), cholesterol and FA composition were determined. For moisture, N, and FA, the same methods as for feed analyses were used, except that the response factor for FA determination was calculated using pork fat. For IMF, the breast meat samples were first hydrolysed using 4 M HCl (BAG Citation1999). Cholesterol was measured in hydrolysed samples by gas chromatography with an Ultra1 column (25 m × 0.32 mm, 0.52 μm film thickness, Agilent, Palo Alto, CA, USA) and quantified by an internal standard (α-5-cholestane, Sigma-Aldrich, Darmstadt, Deutschland) (method 440.1 of the Swiss Food Book).
Thawing and cooking loss were determined in the right breast muscles (n = 8/diet). The muscle was cooked in a sealed bag to 75°C core temperature in a water bath (WB 22 Pump, Gerber Instruments, Effretikon, Switzerland) and cooled for 10 min under cold running water. The cooked meat was cut into 10 × 10 mm cube-shaped strips (10 per breast) using a double knife in the direction of the fibres in order to measure the maximal shear force with a Volodkevich device mounted on a texture analyser (model ProLine table-top machine Z005, Zwick Roell, Ulm, Germany). In this method, two wedge-shaped rods were pressed into the strips of meat until they separated.
Calculations and statistical analysis
The difference in whole-body contents of broilers and 1-day old chicks was used to calculate body retention of N and energy from week 1 to week 9. Coefficients of the metabolisability of N and GE (Joule) were calculated as Vukić Vranješ, Pfirter, and Wenk (Citation1994) outlined. For statistical data analysis, animal individual data measured per day, weekly, or in duplicate were combined to one value per bird. Data were subjected to ANOVA using the linear mixed effect model (procedure MIXED) of SAS (version 9.4, SAS Institute, Cary, NC, USA), reading
Yij = μ + Di + εij
where Yi is the individual observation of the respective variable, μ is the overall mean, D is the effect of the diet, and ε is the error term. Diet was considered as fixed effect and pen, and individual broilers were treated as experimental units. The visual control of normal distribution and homogeneity of variance were performed. Comparisons among Least Square means were performed using the Tukey-Kramer option, considering P < 0.05 as significant, with the trend set at P < 0.10.
Results
Composition of the larvae materials and the complete diets
The analysed N content of the BSFL meal A was highest among the main protein sources, followed by BSFL meal B and the soybean cake (). The experimental diets SS-, AA-, AB- and BB- contained between 29 and 32 g N/kg DM and SS 34 g N/kg DM. Larvae meal A had the highest Met content, while BSFL meal B and the soybean cake were similar in Met content. The soybean cake had a Cys + Met content like that of BSFL meal A and exceeding that of meal B. The Lys content of the soybean cake was about 6 g/kg DM higher than in BSFL meal B, but 2 g/kg DM lower than in BSFL meal A. In relation to the breeder’s recommendations, Lys was fully covered in diet SS, whereas Met and the sum of Cys + Met were below the requirements by 23% and 9%, respectively. All other diets contained less of these amino acids than recommended. Lys was particularly deficient in diet BB-, and Cys + Met was least deficient in diet AA-. The content of EE was similar across diets, except for BB- (+20 g/kg compared to the other diets). Contents of chitin were quite similar between the two BSFL meals. The BSFL meals were darker (lower L* value) and redder than the soybean cake, while the yellowness was more pronounced in the soybean cake. The differences in lightness and redness were less pronounced in the complete diets, but the soybean-based diets were noticeably more yellow than the BSFL-based diets.
Table 2. Analysed chemical composition of the soybean cake, the two larvae meals and the five experimental diets used (g/kg DM unless stated otherwise).
The proportion of SFA in both BSFL fats was about four times higher compared to the proportion in the soybean oil, with LAU accounting for the largest share here, followed by PAM, MYR and stearic acid (18:0, STA) (). The proportion of the n-6 FA mainly represented by linoleic acid (18:2 n-6, LA) in the soybean oil was 3- and 6-times higher than in the BSFL fats A and B, respectively, with 53% of total FAME. Still, the n-6/n-3 FA ratio in soybean oil was comparable to that in BSFL fat B, but twice as high in BSFL fat A. The complete diets reflected the FA profiles of these main lipid sources. Specifically, there were almost 30 times higher LAU proportions in the lipids of the BSFL-based diets than of the soybean-based diets. Similarly, MYR and PAM (only Fat B) proportions were higher in the lipids of the larvae-based diets. The n-6/n-3 FA ratio in the final BSFL-based diets was comparable between each other, although the ratio in BSFL fat A was twice as high as in fat B.
Table 3. Analysed fatty acid composition (% of total fatty acid methyl esters) of the main energy source and the experimental diets.
Performance and metabolisability
All groups started the experimental feeding period on day 15 with a similar average BW of about 210 g (). After 63 d, SS, AA- and AB- birds were heavier (P < 0.001) than the SS- and BB- birds. These differences were also reflected on the ADG (), which varied in average from 19.9 (BB-) to 27.5 g/day (AA-). Concomitantly, the broilers with the poorest ADG consumed the least amount of feed (P < 0.05) (). Thus, the birds of the BB- and SS- groups ate, on average, about 17% less than the birds of the other groups. The feed conversion ratio was affected by the diet (P = 0.003) and was more favourable with SS than with SS- and BB- by −0.4 to 0.5 g ADFI/g ADG, with AA- and AB- being intermediate. The SS birds consumed the most Lys, followed by the AB- and AA- birds. The SS- and BB- birds consumed significantly less Lys compared to the other groups (P < 0.001). The SS, AA- and AB- birds consumed more Met than the SS- and BB- birds (P < 0.001), with a span of 156 mg/day (SS-) to 231 mg/d (AB-). Cysteine intake was also highest with SS, followed by an equal intake in AA- and AB-, and the intake in BB- was lower by 100 mg/day compared to SS; the intake of SS- was intermediate (P < 0.001). The average daily water intake with diet BB- was lower (P < 0.001) by up to 40 ml/day than with diets SS, AA- and AB-. The SS- birds also drank less (P < 0.001) than the SS birds (−26 ml/day).
Table 4. Effect of the five experimental diets on performance1 (n = 8 pens per treatment with two broilers each except for BW and ADG, where individual data are based on n = 16 per diet (n = 15 in case of SS)) and body retention (n = 8 per diet; n = 7 in case of SS).
Figure 1. Weight development of the five dietary treatment groups (means ± SD) (SS = control; soybean cake + soybean oil; SS- = negative control; soybean cake + soybean oil; AA- = larvae meal A + larvae fat A; AB- = larvae meal A + larvae fat B; BB- = larvae meal B rich in larvae fat B) during experimental week 3 to 9 (n = 16, except n = 15 for group SS).
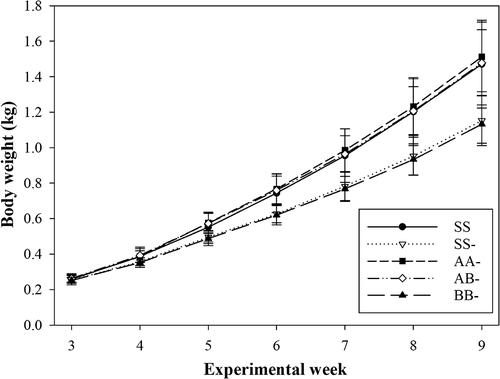
Diet AB- had the lowest, and diet SS the highest N metabolisability (P < 0.001). The dietary differences in energy metabolisability were less pronounced but still present (P < 0.001). It was lower (P < 0.05) with AB- compared to the soybean-based diets and BB-. The measured ME content was higher (P < 0.001) in group BB- than in all other groups that were comparable. The whole-body composition of the broilers did not differ significantly among diets. From week 1 to week 9, with feeding the experimental diets from week 3 through 9, SS, AA- and AB- birds retained more N than those fed BB-, with group SS- being intermediate (P < 0.001). The same order was true for body energy retention. In both variables, AA- birds had the highest retention, N retention was lowest with BB- (28% lower than AA-) and energy retention was lowest with SS- (32% lower than AA-).
Carcase and meat quality
Consistent with the BW at slaughter, carcase weights were higher (P < 0.05) with diets SS, AA- and AB- compared to diets SS- and BB- (). The different treatments did not influence the dressing percentage. Breast meat yield (g/bird) was higher (P < 0.05) with SS, AA- and AB- than with SS- and BB-, but there was a different order in the breast meat proportion of carcase which was higher (P < 0.05) with SS compared to all other diets. There were no diet effects on skin lightness, but on its yellow colouration, as b* was most pronounced with BB- (diet effect, P < 0.05). Consistent with this, diet BB- resulted in the strongest yellow meat colouration compared to the other diets (P < 0.05). There were no differences in lightness and redness of the meat and no diet effects on the breast meat’s pH. The cooking loss of the breast meat of BB- (17.2%) was greater (P = 0.033) than that of the SS meat (14.3%), with all other groups falling in between. Thaw loss tended (P = 0.053) to be higher with BB- than with AA- and SS. Shear force was lower (P = 0.046) with SS- than with BB- and was intermediate in all other groups. The proximate composition of the meat remained unaffected by the diets. The cholesterol content of the meat differed among the diets (P = 0.033), but the multiple comparisons among means did not reveal individual group differences.
Table 5. Effect of the five experimental diets on the characteristics of carcases and breast meat (n = 8/diet).
Fatty acid composition of the intramuscular fat
The greatest diet-mediated variations (P < 0.001) were observed in the intramuscular fat’s composition in the proportions of LAU, MYR, 14:1, PAM, 16:1, oleic acid (C18:1 n-9, OLA), LA, conjugated linoleic acid (9/t11-C18:2, CLA), ALA and arachidonic acid (C20:4 n-6, ADA) (). The proportions of SFA were higher in groups AA-, AB- and BB- compared with SS and SS-, being highest (P < 0.001) in group BB-, where SFA were dominated by PAM, STA and MYR. The LAU proportions were up to 80 times higher (P < 0.001) when feeding the larvae-based diets compared with those of SS and SS-. The breast muscle lipids of groups AA- and AB- contained more (P < 0.05) MUFA than those of SS, with groups SS- and BB- ranging in between. The MUFA were dominated by OLA and 16:1. The PUFA proportions were higher in the IMF of groups SS and SS- compared with that of broilers that were fed BSFL-based diets (P < 0.001). Feeding SS and SS- resulted in greater (P < 0.001) proportions of n-6 and n-3 FA, dominated by LA, ADA and ALA, than the BSFL-based diets. The BB- birds had comparatively high ADA levels. Accordingly, n-6/n-3 FA ratio was lower with SS and SS- compared with the BSFL-based diets (P < 0.001). Diet AA- resulted in the highest (P < 0.05) n-6/n-3 FA ratio.
Table 6. Effect of the five experimental diets on the fatty acid composition of the intramuscular fat (% of total FAME; n = 8/diet).
Discussion
Comparative response of broilers to BSFL-based diets deficient in limiting amino acids
Lysine and Met are considered the most limiting amino acids in broiler diets. Excessive intake of N to cover Lys and Met requirements or an imbalance in the amino acid profile can lead to abundant N excretion, which is harmful to the environment (Kim et al. Citation2006). Therefore, an appropriate supply with limiting amino acids is of high importance. In organic broiler diets, this may be difficult as the use of synthetic amino acids is prohibited. This explains the wide-spread use of the favourably composed soybean protein in this production system. When opposing dietary contents and theoretical requirements of Lys and Met, there was a clear deficiency in the BSFL-based diets (AA-, AB- and BB-) and in the corresponding soybean-based diet (SS-), as intended. Contrary to the calculated composition and different from Lys, Met and the sum of Met + Cys were below the breeder’s recommendations (Hubbard Citation2019), even in the positive soybean-based control diet (SS). The final BW of the SS birds was below the expected final BW of about 2.5 kg that the breeder communicated. However, the ADG of group SS exactly complied with the maximum of 27.5 g/day within 63 d of fattening prescribed for organic broiler production in Switzerland (Bio Suisse Citation2020), which also applies in other European countries, such as France (Ministère de l`agriculture et de l`alimentation Citation2020). This limited the BW at slaughter to about 1.6 kg.
Growth performance revealed differences among diets. Broilers on the two diets containing BSFL meal A (AA- and AB-) exhibited growth superior to those with BSFL meal B (BB-) and soybeans (SS-), and even approached the control birds’ performances. Changes in body N and energy retention over the 9 weeks of growth both largely corresponded to that expected from the birds’ normal growth development. Despite using a slow-growing broiler type, N contents of the whole bodies were comparable to those of conventional broilers of around 2.8 to 3.5% of the BW (Yu et al. Citation1990; Aletor et al. Citation2000).
Several factors might explain the superiority of diets AA- and AB- over BB- and SS-. On a closer look, despite similar ADG, AA- and AB- birds had a numerically higher ADFI, a less favourable feed conversion ratio and exhibited a lower breast meat proportion than SS birds. It remains unclear how the latter was compensated by a greater N deposition somewhere else in the body, as overall body N retention did not differ accordingly. The extra ADFI of the AA- and AB- birds compared to the SS birds ensured a similar Lys and Met supply as with SS, whereas this compensatory measure was not taking place with SS- and BB-. Also, the concomitant increase in body N and energy retention points to an effect of intake rather than of only a particularly high protein value of BSFL meal A. An explanation for the lack of response in BB- could be a slightly lower palatability compared to a soybean-based diet, as found in laying hens (Heuel et al. Citation2021a), whereas diets containing BSFL meal A were similar or even slightly superior in palatability to a diet like SS. For diet BB-, an adverse effect of the particularly high fat content on ADFI, and thus energy concentration (Alqazzaz et al. Citation2019), could not be excluded, but this does not explain the low ADFI with diet SS-.
Another explanation for the different growth responses to the diets could be a difference in nutrient and energy utilisation. However, the differences in N and energy metabolisability were not fully consistent with the growth results. Given the lower growth rate of SS- and BB- birds, an equally lower metabolisability would have been expected, but this was not the case. Also, in laying hens, integrating the same batches of BSFL materials into the diets did not lead to a lower metabolisability compared to a soybean-based diet (Heuel et al. Citation2021a). As with the present study’s broilers, the proportionate differences in N and energy metabolisability were less pronounced than in ADG, and the differences in ADFI seem to have been the overriding factor to the diet’s nutrient and energy concentrations. Still, the latter would affect metabolisability. In this respect, chitin, which has frequently been considered to be widely undigestible and may be found in BSFL at levels up to 87 g/kg DM, may negatively affect even the total diet digestibility of nutrients, especially that of protein and lipids (Kroeckel et al. Citation2012). A newer study (Tabata et al. Citation2017), however, demonstrated that poultry possess an acidic chitinase in the gut that may degrade chitin. Accordingly, the difference in metabolisability between BSFL-based diets and SS- was not very pronounced. Still, it might explain the numerically lower N and energy metabolisability found in the diets with BSFL meal A compared to B since A had a higher chitin content. A deficiency of limiting amino acids can also affect the utilisation of nutrients, especially that of dietary protein (Kim et al. Citation2006). In our case, the greatest total deficiency of limiting amino acids was calculated for diet SS- which, however, did not result in the lowest metabolisability.
Influence of BSFL-based diets on carcase and meat quality
Carcase weight was rather low, especially when compared to other studies with slow-growing broilers where carcase weights were about 1.5 kg (Leiber et al. Citation2017). However, in that study, broilers were fattened for up to 82 d, a period which might have had the same results in the present study. Dressing percentage complied with the target range of up to 68%, a range that is also close to that of conventionally reared broilers (Schiavone et al. Citation2019). The breast meat yield in our study was lower compared to other studies on the performance of broilers fed a BSFL meal or fat. However, in these studies the birds were either fattened longer (Leiber et al. Citation2017) or were growing types (Schiavone et al. Citation2019; de Souza Vilela et al. Citation2021). Nevertheless, an influence of the Met deficit cannot be excluded here in all groups.
Important carcase quality traits, such as carcase weight, dressing percentage, or breast meat yield, were not generally impaired by replacing the soybean cake with BSFL. Consistent with growth performance, birds on the BSFL-based diets AA- and AB- were even quite competitive to SS, though not fully reaching the same breast meat proportion as SS, as discussed earlier. Even the numerically lower breast meat yield of about 30 g per bird compared to SS could be relevant in terms of the profitability of poultry production. Under the same condition of a deficit in limiting amino acids, AA- and AB- birds were superior to SS- by more than 50 g breast meat per bird. In other studies, there were also only limited effects on carcase quality when replacing other protein sources partly or fully with BSFL meal. For example, Leiber et al. (Citation2017) did not record adverse effects on the weight of the main meat cuts in organic broilers when the soybean material was partially replaced by BSFL in combination with alfalfa or pea and compared to a soybean-based control diet. Also, in fast-growing broilers, an increasing proportion of BSFL (50 to 150/200 g/kg), as a partial replacement for soybeans in the diet, had no negative effect on carcase quality (Schiavone et al. Citation2019; de Souza Vilela et al. Citation2021).
Yellowness of skin and meat may have an influence on consumer acceptance. This influence is mostly negative, but in some countries or regions, the meat and skin having an intense colour may lead to an increase in demand (Kennedy et al. Citation2005; Wideman et al. Citation2016). In the present study, the skin and meat of the birds fed diet BB- had a more intense yellow colouration. This is probably due to BSFL meal B and the corresponding rearing substrate of the BSFL (predominantly vegetables, fruits and stuffed pasta), but not of the fat B, as otherwise also AB- birds would have exhibited this yellow colouration. The skin’s and breast meat’s colour are determined by pigments, especially xanthophylls, that accumulate in the epidermis and the muscle. Depending on the content of pigments in the diet, the colour changes in skin and meat are variable (Pérez-Vendrell et al. Citation2001). One feed rich in xanthophylls is maize (Brown et al. Citation2008), known to cause yellow colouration of the skin and meat. However, since diet BB- had the same maize proportion as diets AA- and AB-, and as SS- even included slightly more maize, a maize effect could be excluded. The results of Schiavone et al. (Citation2019) demonstrated that yellowing is not a general property of BSFL meal as, in their study, increasing the BSFL meal proportion resulted in a decreasing yellowness of the meat. By contrast, Cullere at al. (Citation2019) found a numerically higher yellow colouration of leg meat when soybean oil was partially or completely replaced by BSFL fat in broilers’ diets. These publications did not describe the exact rearing method of the BSFL used as feeds. The extent of increase in yellowness when fed BB-, though significant, was hardly perceptible to the naked eye and only in direct comparison. However, if BSFL substrates which are even richer in pigments are used, a limitation of BSFL meal in broiler diets (or vice versa, its promotional use) may be required.
The high cooking loss of the meat from group BB-, in comparison with the other groups, was associated with an elevated shear force (an indicator of a lower meat tenderness). There is a known association between these two traits (Toscas et al. Citation1999). Both point towards an adverse influence of BSFL meal B on meat quality, since such values did not occur in any other group. However, causal relationships of this effect that are driven by only one of the BSFL meal types remain elusive. Reasons associated with high cooking loss (comparatively high PUFA content, low pH, deviating procedure in the freezing process) can be excluded (Mir et al. Citation2017). Also, an effect of the slower meat retention in the body can be excluded because there was no corresponding response with diet SS-. Compared to previously published values for cooking loss of meat from the broilers that were fed BSFL, the results shown in the present study are on the lower end. Leiber et al. (Citation2017) observed a similar level of cooking loss in diets based on BSFL plus alfalfa or peas, whereas de Souza Vilela et al. (Citation2021) found cooking loss as high as 30% when 20% full-fat BSFL was added to the diet of 42-day-old broilers. In both studies, no BSFL effects were reported; however, none of the available studies has ever investigated differently produced BSFL in a single setup with intentionally induced amino acid deficiency in the diet.
Feeding BSFL had no effect on meat gross nutrient composition and cholesterol content. The latter varied between 0.33 and 0.37 g/kg meat, with values being lower than the 0.55 g cholesterol/kg that Dalle Zotte and Szendrő (Citation2011) reported for chicken meat. Previous studies with conventional broilers (Kim et al. Citation2020), quails (Cullere et al. Citation2018) and turkeys (Sypniewski et al. Citation2020) fed BSFL meal or fat agree with our results and could not find increased levels of neither cholesterol concentrations in the meat nor in the blood serum.
Differences in the fatty acid profile of the intramuscular fat
One characteristic of the BSFL fat is its high content of medium-chain FAs, especially LAU and MYR (Heuel et al. Citation2021b). Different from the low transfer from feed to egg reported in laying hens (Heuel et al. Citation2021b), the present results demonstrated that the IMF of broilers who were fed BSFL-based diets was clearly richer in LAU and MYR, and, depending on the origin of the BSFL, higher in MUFA and lower in PUFA compared to IMF of the meat from broilers on soybean-based diets. Similarly, Kim et al. (Citation2020), supplementing a soybean-based broiler diet with BSFL fat replacing maize oil, found a significant increase in proportions of SFA, including LAU, MYR, PAM and MUFA, and a lower PUFA proportion in the abdominal fat of the birds. Cullere et al. (Citation2019) showed that replacing half or all soybean oil in the broilers’ diet with BSFL fat increases the proportion of SFA (mainly caused by increased LAU and MYR proportions), while it reduces the proportion of PUFA.
It therefore seems that different from the patterns observed for egg lipid formation, the FA retention in broiler bodies largely matched the FA intake. The FA profiles of the IMF seem sufficiently indicative of a more intense body deposition of the main medium-chain FA compared with their retention in the egg. Accordingly, in the broilers’ metabolism their relative utilisation for other metabolic pathways, particularly energy utilisation, is comparatively limited. The medium-chain FA are considered dietetically undesirable, because especially LAU and MYR are known for their hypercholesterolemic properties (Ulbricht and Southgate Citation1991). The SFA, including these FA, generally have a lower susceptibility to FA oxidation compared to MUFA and PUFA, which increase the shelf life of the meat (Woods and Feron Citation2009). Additionally, LAU has also been shown to have an antimicrobial effect on intestinal bacteria in broilers and thus may still have some health benefits (Zeitz et al. Citation2015). In the present study, PUFA proportions in the IMF went down with the BSFL-based diets compared to the soybean-based diets, and the n-6/n-3 FA ratio was unfavourably increased (Young Citation2009) (less so with BSFL fat B). Cullere et al. (Citation2019) also reported an increase in the n-6/n-3 FA ratio in the meat with the use of BSFL fat. An improvement would be possible, in this respect, when using material from BSFL reared on substrates containing components like fish offal or seaweed (St-Hilaire et al. Citation2007; Liland et al. Citation2017).
In conclusion, the results of the present study showed that soybean-based feeds can be exchanged with BSFL meals and fats in the broilers’ diet as there was no significant loss in performance, even in organic-type diets where no synthetic amino acids may be supplemented. This suggests that it is possible to fully profit from the environmental advantages of BSFL over soybean without causing problems by higher N losses and a lower N utilisation in these slow-growing birds. Despite a clearly calculated deficiency of limiting amino acids in the experimental diets, one of the BSFL sources showed a certain compensation potential and led to a better performance than when fed a soybean-based diet that was similarly deficient in amino acids. The birds almost reached the same performance of those on a soybean-based diet that was not as deficient in amino acid supply. This was mainly the result of a compensatorily higher feed intake rather than of a higher content of metabolically available, limiting amino acids. The results confirm hypothesis 1 for one of the BSFL batches (A), but not for the other (B). It shows that the quality of the BSFL meal, especially its palatability, plays a crucial role. Superiority of BSFL to soybeans also applied to the carcase (only one of the materials) and meat quality (mostly for both materials), confirming hypothesis 2. Attention must be paid to the level of pigments present in BSFL material as this may lead to yellow skin and meat of the broilers and could influence the carcases’ retailing quality. Disproving hypothesis 3, feeding BSFL, which is rich in LAU and MYR, resulted in a non-negligible increase in these FA in broiler meat lipids, although their original, extreme levels in BSFL materials were not reached. This must be considered in terms of meat quality for human nutrition. Completely replacing soybean-based feeds in the diets of organic broilers with high-quality BSFL can be recommended, but it would be advantageous to use specific larval substrates, thereby causing the larvae to depose more of the favourable FA and to feed high-LAU BSFL to laying hens.
Acknowledgments
The present research was funded by the Mercator Research Program of the World Food System Center at ETH Zurich and the Swiss Federal Office for Agriculture (grant no. 627000824). We would like to especially thank Carmen Kunz, Muna Mergani, Pascal Bucher and Nico Perez from ETH Zurich for their invaluable support in the laboratory throughout the entire study. Furthermore, we thank Bio Partner Schweiz AG, Seon; Brauerei Müller AG, Baden, Pastinella AG, Oberentfelden and Coop-Bananenreiferei, Kaiseraugst for providing BSFL substrates B, and Jens Wohlfahrt, Uwe Krug, and Markus Leubin for managing the insect production at FiBL. We are grateful to Margit Wittman for her valuable advice in determining the whole carcase composition.
Disclosure statement
No potential conflict of interest was reported by the author.
Additional information
Funding
References
- Aletor, V. A., I. I. Hamid, E. Niess, and E. Pfeffer. 2000. “Low‐Protein Amino Acid‐Supplemented Diets in Broiler Chickens: Effects on Performance, Carcass Characteristics, Whole‐Body Composition and Efficiencies of Nutrient Utilisation.” Journal of the Science of Food and Agriculture 80: 547–554. doi:10.1002/(SICI)1097-0010(200004)80:5<547::AID-JSFA531>3.0.CO;2-C.
- Alqazzaz, M., A. A. Samsudin, L. H. Idris, D. Ismail, and H. Akit. 2019. “Effect of Energy to Protein Ratio Using Alternative Feed Ingredients on Growth Performance and Nutrient Digestibility in Broilers.” Indian Journal of Animal Research 53: 1069–1073.
- AOAC. 1997. Association of Official Analytical Chemists In: Official Methods of Analysis. Arlington, VA, USA: Association of Official Analytical Chemists).
- BAG (Swiss Federal Office of Health). 1999. Bestimmung des Gesamtfettgehaltes von Fleisch und Fleischerzeugnissen. Methode 319.1. Berne, Switzerland: Swiss Food Manual.
- Barragan-Fonseca, K. B., M. Dicke, and J. J. A. van Loon. 2017. “Nutritional Value of the Black Soldier Fly (Hermetia Illucens L.) And Its Suitability as Animal Feed – A Review.” Journal of Insects as Food and Feed 3 (2): 105–120. doi:10.3920/JIFF2016.0055.
- Bio Suisse. 2020. “Richtlinien für die Erzeugung, Verarbeitung und Handel von Knospe-Produkten. Teil II: Richtlinien für den Pflanzenbau und die Tierhaltung in der Schweiz.” Accessed May 2021. https://www.bio-suisse.ch/media/VundH/Regelwerk/2019/DE/rl_2019_1.1_d_teil_ii__30.11.2018.pdf
- Brown, S. N., G. R. Nute, A. Baker, S. I. Hughes, and P. D. Warriss. 2008. “Aspects of Meat and Eating Quality of Broiler Chickens Reared under Standard, Maize-Fed, Free-Range or Organic Systems.” British Poultry Science 49 (2): 118–124. doi:10.1080/00071660801938833.
- Cullere, M., A. Schiavone, S. Dabbou, L. Gasco, and A. D. Zotte. 2019. “Meat Quality and Sensory Traits of Finisher Broiler Chickens Fed with Black Soldier Fly (Hermetia Illucens L.) Larvae Fat as Alternative Fat Source.” Animals 9 (4): 140. doi:10.3390/ani9040140.
- Cullere, M., G. Tasoniero, V. Giaccone, G. Acuti, A. Marangon, and A. Dalle Zotte. 2018. “Black Soldier Fly as Dietary Protein Source for Broiler Quails: Meat Proximate Composition, Fatty Acid and Amino Acid Profile, Oxidative Status and Sensory Traits.” Animal 12 (3): 640–647. doi:10.1017/S1751731117001860.
- Dabbou, S., F. Gai, L. Biasato, M. T. Capucchio, E. Biasibetti, D. Dezzutto, M. Meneguz, I. Palcha, L. Gasco, and A. Schiavone. 2018. “Black Soldier Fly Defatted Meal as a Dietary Protein Source for Broiler Chickens: Effects on Growth Performance, Blood Traits, Gut Morphology and Histological Features.” Journal of Animal Science and Biotechnology 9 (1): 49. doi:10.1186/s40104-018-0266-9.
- Dalle Zotte, A., and Z. Szendrő. 2011. “The Role of Rabbit Meat as Functional Food.” Meat Science 88 (3): 319–331. doi:10.1016/j.meatsci.2011.02.017.
- de Souza Vilela, J., T. I. Alvarenga, N. R. Andrew, M. McPhee, M. Kolakshyapati, D. L. Hopkins, and I. Ruhnke. 2021. “Technological Quality, Amino Acid and Fatty Acid Profile of Broiler Meat Enhanced by Dietary Inclusion of Black Soldier Fly Larvae.” Foods 10 (2): 297. doi:10.3390/foods10020297.
- El-Hack, A. B. D., A. E. Mohamed, M. E. Shafi, W. Y. Alghamdi, S. A. Abdelnour, A. M. Shehata, Â. E. Noreldin, et al. 2020. “Black Soldier Fly (Hermetia Illucens) Meal as A Promising Feed Ingredient for Poultry: A Comprehensive Review.” Agriculture 10: 339. doi:10.3390/agriculture10080339.
- Ewusie, E. A., P. K. Kwapong, G. Ofosu-Budu, C. Sandrock, A. Akumah, E. Nartey, C. Teye-Gaga, S. K. Agyarkwah, and N. Adamtey. 2018. “Development of Black Soldier Fly, Hermetia Illucens (Diptera: Stratiomyidae) in Selected Organic Market Waste Fractions in Accra, Ghana.” Asian Journal of Biotechnology and Bioresource Technology 4 (1): 1–16. doi:10.9734/AJB2T/2018/42371.
- Gold, M., C. M. Cassar, C. Zurbrügg, M. Kreuzer, S. Boulos, S. Diener, and A. Mathys. 2020. “Biowaste Treatment with Black Soldier Fly Larvae: Increasing Performance through the Formulation of Biowastes Based on Protein and Carbohydrates”. Waste Management 102: 319–329. doi:10.1016/j.wasman.2019.10.036.
- Heuel, M., C. Sandrock, F. Leiber, A. Mathys, M. Gold, C. Zurbrügg, I. D. M. Gangnat, M. Kreuzer, and M. Terranova. 2021a. “Black Soldier Fly Larvae Meal and Fat Can Completely Replace Soybean Cake and Oil in Diets for Laying Hens.” Poultry Science 100 (4): 101034. doi:10.1016/j.psj.2021.101034.
- Heuel, M., C. Sandrock, F. Leiber, A. Mathys, M. Gold, C. Zurbrügg, I. D. M. Gangnat, M. Kreuzer, and M. Terranova. 2021b. “Transfer of Lauric and Myristic Acid from Black Soldier Fly Larval Lipids to Egg Yolk Lipids of Hens Is Low.” Lipids 56: 423–435. doi:10.1002/lipd.12304.
- Hubbard. 2019. Hubbard Breeders Management Guide Broiler. Accessed April 2021. https://www.winmixsoft.com/files/info/Hubbard%20Broiler%20Management%20Guide.pdf
- Ipema, A. F., W. J. Gerrits, E. A. Bokkers, B. Kemp, and J. E. Bolhuis. 2020. “Provisioning of Live Black Soldier Fly Larvae (Hermetia Illucens) Benefits Broiler Activity and Leg Health in a Frequency- and Dose-Dependent Manner.” Applied Animal Behaviour Science 230: 105082. doi:10.1016/j.applanim.2020.105082.
- IUPAC. 1991. “Preparation of the Fatty Acid Methyl Esters.” In Standard Methods for Analysis of Oils, Fats and Derivates, edited by A. Dieffenbacher and W. D. Pocklington, 123–129. 7th ed. Oxford, England: Blackwell.
- Kennedy, O. B., B. J. Stewart-Knox, P. C. Mitchell, and D. I. Thurnham. 2005. “Flesh Colour Dominates Consumer Preference for Chicken.” Appetite 44: 181–186. doi:10.1016/j.appet.2004.11.002.
- Kim, W. K., C. A. Froelich, P. H. Patterson, and S. C. Ricke. 2006. “The Potential to Reduce Poultry Nitrogen Emissions with Dietary Methionine or Methionine Analogues Supplementation.” Worlds Poultry Science Journal 62 (2): 338–353. doi:10.1079/WPS2005103.
- Kim, Y. B., D. H. Kim, S. B. Jeong, J. W. Lee, T. H. Kim, H. G. Lee, and K. W. Lee. 2020. “Black Soldier Fly Larvae Oil as an Alternative Fat Source in Broiler Nutrition.” Poultry Science 99: 3133–3143. doi:10.1016/j.psj.2020.01.018.
- Kroeckel, S., A. G. Harjes, I. Roth, H. Katz, S. Wuertz, A. Susenbeth, and C. Schulz. 2012. “When a Turbot Catches a Fly: Evaluation of a Pre-Pupae Meal of the Black Soldier Fly (Hermetia Illucens) as Fish Meal Substitute – Growth Performance and Chitin Degradation in Juvenile Turbot (Psetta Maxima).” Aquaculture 364: 345–352. doi:10.1016/j.aquaculture.2012.08.041.
- Leiber, F., T. Gelencsér, A. Stamer, Z. Amsler, J. Wohlfahrt, B. Früh, and V. Maurer. 2017. “Insect and Legume-Based Protein Sources to Replace Soybean Cake in an Organic Broiler Diet: Effects on Growth Performance and Physical Meat Quality.” Renewable Agriculture and Food Systems 32 (1): 21–27. doi:10.1017/S1742170515000496.
- Liland, N. S., I. Biancarosa, P. Araujo, D. Biemans, C. G. Bruckner, R. Waagbø, B. E. Torstensen, and E. J. Lock. 2017. “Modulation of Nutrient Composition of Black Soldier Fly (Hermetia Illucens) Larvae by Feeding Seaweed-Enriched Media.” PloS One 12: e0183188. doi:10.1371/journal.pone.0183188.
- Ministère de l`agriculture et de l`alimentation. 2020. “Guide de Lecture du RCE N° 834/2007 Et du RCE N° 889/2008”. Accessed June 2021 (in French. https://www.inao.gouv.fr/content/download/1352/13877/version/18/file/GUIDE-de-LECTURE-RCE-BIO%202020-01.pdf
- Mir, N. A., A. Rafiq, F. Kumar, V. Singh, and V. Shukla. 2017. “Determinants of Broiler Chicken Meat Quality and Factors Affecting Them: A Review.” Journal of Food Science and Technology 54 (10): 2997–3009. doi:10.1007/s13197-017-2789-z.
- Pérez-Vendrell, A. M., J. M. Hernandez, L. Llaurado, J. Schierle, and J. Brufau. 2001. “Influence of Source and Ratio of Xanthophyll Pigments on Broiler Chicken Pigmentation and Performance.” Poultry Science 80 (3): 320–326. doi:10.1093/ps/80.3.320.
- Pieterse, E., S. W. Erasmus, T. Uushona, and L. C. Hoffman. 2019. “Black Soldier Fly (Hermetia Illucens) Pre‐Pupae Meal as a Dietary Protein Source for Broiler Production Ensures a Tasty Chicken with Standard Meat Quality for Every Pot.” Journal of the Science of Food and Agriculture 99: 893–903. doi:10.1002/jsfa.9261.
- Schiavone, A., S. Dabbou, S. M. De Marco, M. Cullere, I. Biasato, E. Biasibetti, M. T. Capucchio, et al. 2018. “Black Soldier Fly Larval Fat Inclusion in Finisher Broiler Chicken Diet as an Alternative Fat Source.” Animal 12: 2032–2039. doi:10.1017/S1751731117003743.
- Schiavone, A., S. Dabbou, M. Petracci, M. Zampiga, F. Sirri, I. Biasato, F. Gai, and L. Gasco. 2019. “Black Soldier Fly Defatted Meal as a Dietary Protein Source for Broiler Chickens: Effects on Carcass Traits, Breast Meat Quality and Safety.” Animal 13 (10): 2397–2405. doi:10.1017/S1751731119000685.
- Smetana, S., E. Schmitt, and A. Mathys. 2019. “Sustainable Use of Hermetia Illucens Insect Biomass for Feed and Food: Attributional and Consequential Life Cycle Assessment.” Resources, Conservation and Recycling 144: 285–296. doi:10.1016/j.resconrec.2019.01.042.
- St‐Hilaire, S., K. Cranfill, M. A. McGuire, E. E. Mosley, J. K. Tomberlin, L. Newton, W. Sealey, C. Sheppard, and S. Irving. 2007. “Fish Offal Recycling by the Black Soldier Fly Produces a Foodstuff High in Omega‐3 Fatty Acids.” Journal of the World Aquaculture Society 38: 309–313. doi:10.1111/j.1749-7345.2007.00101.x.
- Sypniewski, J., B. Kierończyk, A. Benzertiha, Z. Mikołajczak, E. Pruszyńska-Oszmałek, P. Kołodziejski, M. Sassek, M. Rawski, W. Czekala, and D. Józefiak. 2020. “Replacement of Soybean Oil by Hermetia Illucens Fat in Turkey Nutrition: Effect on Performance, Digestibility, Microbial Community, Immune and Physiological Status and Final Product Quality.” British Poultry Science 61 (3): 294–302. doi:10.1080/00071668.2020.1716302.
- Tabata, E., A. Kashimura, S. Wakita, M. Ohno, M. Sakaguchi, Y. Sugahara, Y. Kino, V. Matoska, P. O. Bauer, and F. Oyama. 2017. “Gastric and Intestinal Proteases Resistance of Chicken Acidic Chitinase Nominates Chitin-Containing Organisms for Alternative Whole Edible Diets for Poultry.” Scientific Reports 7 (1): 6662. doi:10.1038/s41598-017-07146-3.
- Taherzadeh, O., and D. Caro. 2019. “Drivers of Water and Land Use Embodied in International Soybean Trade.” Journal of Cleaner Production 223: 83–93. doi:10.1016/j.jclepro.2019.03.068.
- Toscas, P. J., F. D. Shaw, and S. L. Beilken. 1999. “Partial Least Squares (Pls) Regression for the Analysis of Instrument Measurements and Sensory Meat Quality Data.” Meat Science 52 (2): 173–178. doi:10.1016/S0309-1740(98)00165-X.
- Ulbricht, T. L. V., and D. A. T. Southgate. 1991. “Coronary Heart Disease: Seven Dietary Factors.” The Lancet 338 (8773): 985–992. doi:10.1016/0140-6736(91)91846-M.
- van Huis, A. 2020. “Insects as Food and Feed, A New Emerging Agricultural Sector: A Review.” Journal of Insects as Food and Feed 6 (1): 27–44. doi:10.3920/JIFF2019.0017.
- Vukić Vranješ, M., H. P. Pfirter, and C. Wenk. 1994. “Influence of Processing Treatment and Type of Cereal on the Effect of Dietary Enzymes in Broiler Diets.” Animal Feed Science and Technology 46: 261–270. doi:10.1016/0377-8401(94)90144-9.
- Wideman, N., C. A. O’Bryan, and P. G. Crandall. 2016. “Factors Affecting Poultry Meat Colour and Consumer Preferences – A Review.” World’s Poultry Science Journal 72 (2): 353–366. doi:10.1017/S0043933916000015.
- Woods, M. J., M. Cullere, L. Van Emmenes, S. Vincenzi, E. Pieterse, L. C. Hoffman, and A. D. Zotte. 2019. “Hermetia Illucens Larvae Reared on Different Substrates in Broiler Quail Diets: Effect on Apparent Digestibility, Feed-choice and Growth Performance.” Journal of Insects as Food and Feed 5 (2): 89–98. doi:10.3920/JIFF2018.0027.
- Woods, V. B., and A. M. Fearon. 2009. “Dietary Sources of Unsaturated Fatty Acids for Animals and Their Transfer into Meat, Milk and Eggs: A Review.” Livestocks Science 126 (1–3): 1–20. doi:10.1016/j.livsci.2009.07.002.
- Young, K. 2009. “Omega-6 (N-6) and Omega-3 (N-3) Fatty Acids in Tilapia and Human Health: A Review.” International Journal of Food Sciences and Nutrition 60 (sup5): 203–211. doi:10.1080/09637480903140503.
- Yu, M. W., F. E. Robinson, M. T. Clandinin, and L. Bodnar. 1990. “Growth and Body Composition of Broiler Chickens in Response to Different Regimens of Feed Restriction.” Poultry Science 69 (12): 2074–2081. doi:10.3382/ps.0692074.
- Zeitz, J. O., J. Fennhoff, K. Kluge, G. I. Stangl, and K. Eder. 2015. “Effects of Dietary Fats Rich in Lauric and Myristic Acid on Performance, Intestinal Morphology, Gut Microbes, and Meat Quality in Broilers.” Poultry Science 94: 2404–2413. doi:10.3382/ps/pev191.