ABSTRACT
Cecropia pachystachya is a myrmecophyte which has domatia and nutritional resources used by ants of the genus Azteca. In addition to Müllerian bodies, another type of structure, food bodies (FBs), are present on the leaves of this plant, which is the focus of this study. Leaf samples were collected and prepared for light, and transmission and scanning electron microscopy. Histochemical analysis and thin layer chromatography were also employed to determine the composition of reserves. Food bodies are found in young leaves, distributed on the leaf blades and petioles. On leaf blades, FBs occur predominantly on the abaxial surface and are always associated with veins. Food bodies are emergences produced by anticlinal divisions of protodermic cells and divisions of the underlying ground tissue. Mature FBs are claviform, translucent and show fragile stalks. Similar to the epidermal cells, the inner parenchyma cells have thin walls and cytoplasm with few mitochondria and endoplasmic reticulum segments; their reserves consist of oil droplets dispersed in the cytoplasmic matrix and reducing sugars in the large central vacuole. Because of the liquid reserves that allow them to be consumed by adult ants, these FBs differ from the well-studied Müllerian bodies. Food bodies are collected by ants after stipule rupture and immediately consumed. Our results suggest that these FBs act in a complementary function to the Müllerian bodies, but this hypothesis remains to be tested. Searching for FBs, ants patrol young leaves and this seems to reduce response time to herbivory, thereby reducing leaf damage.
Introduction
According to Webber et al. (Citation2007b), myrmecophily is a term employed to describe any relationship between ants and another organism. This concept includes the mutualistic relationships between plants and ants, which is maintained by plants in two primary ways: plants provide nutrients and are termed myrmecophilous; or plants offer housing, and perhaps also food, and are known as myrmecophytes. In return, ants may provide protection to plants against herbivores (O’Dowd Citation1980; Rocha & Bergallo Citation1992; Gaume et al. Citation1997; Bronstein et al. Citation2006), vines (Janzen Citation1969) and pathogens (Fuente & Marquis Citation1999).
The production of nutritional resources by plants varies in relation to quantity and quality, and depending on abiotic and biotic conditions (Janzen Citation1973; Folgarait & Davidson Citation1994, Citation1995; Heil et al. Citation1998). Among the structures involved in the synthesis and supply of food resources by plants, nectaries, oil glands and food bodies (FBs) stand out.
Food bodies can be uni- or multi-celled trichomes or be characterised as emergences in which parenchyma occupies the central portion (Buono et al. Citation2008). Food bodies may contain proteins, lipids or carbohydrates in different proportions, and serve to maintain mutualistic relationships between the plant and ants.
The presence of FBs is well known in the genus Cecropia, whose interaction with ants of the genus Azteca has been the subject of several, mostly ecological, studies (Janzen Citation1969, Citation1973; Rickson Citation1976; Longino Citation1989; Dejean et al. Citation2012). Azteca colonies are located in domatia found throughout the stem axis of several species of Cecropia. These mutualistic ants find not only a nesting site, but also food in the form of Müllerian bodies that the plant provides at the base of the petiole (Janzen Citation1969). These Müllerian bodies are peculiar because they store glycogen in addition to the usual lipids and proteins (Rickson Citation1976).
Although FBs of species of Cecropia have been the subject of several studies, these works focused on the Müllerian bodies, which occur in the base of the petiole. We call attention to the FBs in other portions of the leaf, since these structures are still poorly known due to absence of ontogenetic and structural studies. Although not based on structural analysis, Rickson (Citation1976) called these FBs ‘pearl glands’, stating that they differ morphologically from Müllerian bodies by being trichomes, whereas Müllerian bodies are emergences. This author also reports the presence of few glycogen plastids and purely lipid reserves in pearl glands, as opposed to the conspicuous accumulation of glycogen in Müllerian bodies. According to Davidson (Citation2005) these pearl glands can provide ants with lipids and amino acids.
The lack of structural and ontogenetic studies of FBs other than Müllerian bodies of species of Cecropia, and the importance of these structures in mediating the mutualistic interactions between ants and plants, have motivated the present study. Here we analyse the structural and ultrastructural organisation and development of FBs produced in young leaves of Cecropia pachystachya Trecul and identify their main reserve substances, with the aim of understanding their possible complementary function as ant attractants in relation to the well-studied Müllerian bodies.
Materials and methods
Light microscopy and food body composition
Samples were collected from six adult Cecropia pachystachya growing on the campus of the Universidade Federal de Minas Gerais, Minas Gerais, Brazil. All sampled specimens were inhabited by colonies of ants of the genus Azteca. The samples consisted of fragments removed from young leaves, immediately prior to stipule rupture, and from younger leaves from the node immediately above.
Samples for light microscopy were fixed in Karnovsky’s fixative (Karnovsky Citation1965) for 24 h, dehydrated in an ethyl alcohol series (Johansen Citation1940) and embedded in synthetic resin (2-hydroxyethyl)-methacrylate (Leica). Serial transverse and longitudinal sections (6 µm) were produced with a rotary microtome, and the slides were stained with 0.05% toluidine blue pH 6.8 (O’Brien et al. Citation1964). Subsequently, the slides were counterstained with 0.05% aqueous ruthenium red solution. The photomicrographs were acquired using an Olympus® BHZ digital image capture system.
Histochemical tests and their respective controls were performed to detect substances of the main metabolic groups, using: Lugol solution to detect starch (Johansen Citation1940); ferric chloride plus a little sodium carbonate for tannins (Johansen Citation1940); and Sudan red B for lipids (Brundett et al. Citation1991), the latter being also used for fresh, non-fixed FBs.
For sugar analysis, FBs were sampled with a needle, macerated, and reserves were extracted with pure methanol. The obtained extract was immediately analysed by thin layer chromatography (TLC) to detect the presence of sugar. We employed 10 × 10 cm silica gel TLC plates prepared with 0.02% sodium acetate. The sample and standard marker solutions for fructose, glucose, sucrose and distilled water (control) were applied to the TLC plates. The plates were run with an n-butyl alcohol, acetone, acetic acid and water (14:14:4:8 v/v) mobile phase. Subsequently, the plates were dried at room temperature and sprayed with an orcinol-sulphuric acid solution, followed by heating to 120 °C for 5 min. The sugars appeared as dark-purple bands against a yellow background (Stahl Citation1988). Assays with glucose strips were employed in macerate of fully developed FBs to confirm glucose presence.
Electron microscopy
For scanning electron microscopy (SEM) leaves were examined under a stereomicroscope to ensure the presence of FBs for sampling. Samples were then fixed in a 2.5% glutaraldehyde solution (0.1 M phosphate buffer, pH 7.2), dehydrated in an ethanol series, dried to the critical point and sputter-coated with 10 nm of gold (Robards Citation1978). The samples were analysed on a Quanta 200 Scanning Electron Microscope (FEI Company) with digital image capture at 12–20 kV.
For transmission electron microscopy (TEM) samples of leaf blades with young and mature FBs were prepared using conventional methods (Roland Citation1978). These samples were fixed in Karnovsky’s solution (Karnovsky Citation1965) for 24 h, post-fixed in 1% osmium tetroxide (0.1 M phosphate buffer, pH 7.2) and dehydrated in a graded acetone series for embedding in Araldite resin. Ultrathin sections (50 nm) were contrasted with a saturated solution of uranyl acetate and lead citrate. The sections were examined using a Tecnai G2–Spirit Transmission Electron Microscope (Philips/FEI Company) at 80 kV.
Results
Distribution and structure
The FBs of C. pachystachya were randomly distributed throughout the leaf blades and petioles of young leaves. On the leaf blade FBs were always associated with the prominent veins, particularly the midrib (A), and predominantly on the abaxial surface. On the abaxial surface, FBs occurred among a dense indumentum formed by simple and arachnoid trichomes (B–D). The fully developed FBs were translucent and club-shaped (B–C). The FBs had fragile stalks (B–C), making them easily detachable (D).
The observation of the collected samples indicated that FBs were collected by ants as soon as stipule rupture occurred, with a consequent exposure of the developing leaf. They were consumed by adult ants, rather than being carried to the nest. After stipule rupture the FBs became scarce and completely dehydrated, and are almost absent in fully expanded leaves.
The FBs had a uniseriate epidermis consisting of juxtaposed tabular cells with a thin cuticle (B, E); stomata were not observed in these structures. At maturity the epithelial cells of the FBs were highly vacuolated and devoid of reserve substances (B). In some FBs we observed, by SEM, a pore in one of the epidermal cells of the apical region (E–F). This pore was clearly outlined and appeared not to be restricted to the cuticle, therefore it created an aperture in the FB.
The cells that comprise the inner tissue of the FBs were parenchymatous and exhibited thin pectocellulosic walls. In mature FBs, these cells showed a large central vacuole forcing the remaining cytoplasm to be restricted to a thin peripheral layer (B). Histochemical tests showed that the parenchyma cells accumulate oil. The presence of starch, rarely detected by histochemical tests, was restricted to a few grains sometimes observable in TEM. Thin layer chromatography analyses showed the presence of soluble sugars (glucose and fructose) within the FBs, probably stored in the vacuole. There were no vascular elements in the FBs (, A–C). The vascular tissue of the midrib did not show ramifications towards FBs.
Figure 1. Food bodies of the leaf of C. pachystachya. A, General aspect of the young leaf before stipule rupture, arrows indicate FBs; B, fully developed FB, note a fragile stalk; C–D, FBs observed under SEM, note the dense indumentum and, in D, the stalk rupture; E–F, detail of the surface of a FB showing thin cuticle and a pore (arrow). Abbreviations: cc, central cells; ep, epidermis; st, stalk.
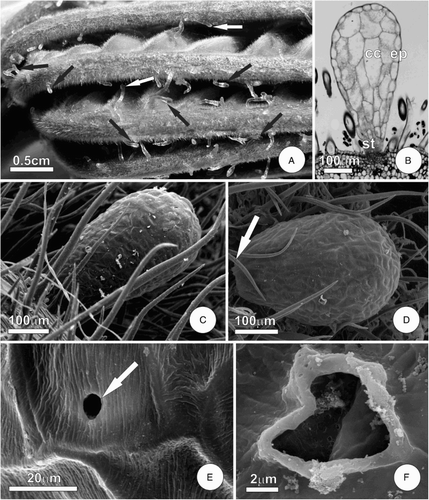
Figure 2. Diagram showing major developmental stages of FBs on the leaf of C. pachystachya. A, Initial stage of FB formation, note the elevation of epidermis due to proliferation of the underlying tissue; B–C, FB at the expansion stage, note the effects of cell expansion and, in C, the persistence of meristematic activity in the stalk cells.
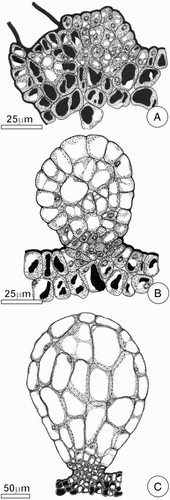
Ontogenetic studies indicated that FBs from C. pachystachya were formed by anticlinal cell divisions of protoderm, and divisions in various planes of the subjacent ground parenchyma. The meristematic activity persisted in the stalk region, even when the apical cells were fully differentiated (A–C).
Ultrastructure
The epidermal cells showed outer periclinal walls characteristically laminated (A–B), while the remaining faces had thin and uniform walls. The vacuole was located in a central position, and lacked reserve compounds and phenolic substances. The remaining cytoplasm was restricted to a thin layer in a peripheral position, and had a few mitochondria and endoplasmic reticulum as predominant organelles.
Figure 3. Ultrastructural aspects of FBs of the leaf of C. pachystachya. A–B, Epidermal cell with lamellar outer periclinal wall and organelles in peripheral cytoplasm; C–F, parenchyma of the core of the FBs. In C–D note the cell with large vacuole and lobated nucleus. Detail of peripheral cytoplasm with mitochondria is shown in E–F, note in F a large oil droplet. Abbreviations: di, dictyosome; er, endoplasmic reticulum; mi, mitochondria; nu, nucleus; od, oil droplet; va, vacuole.
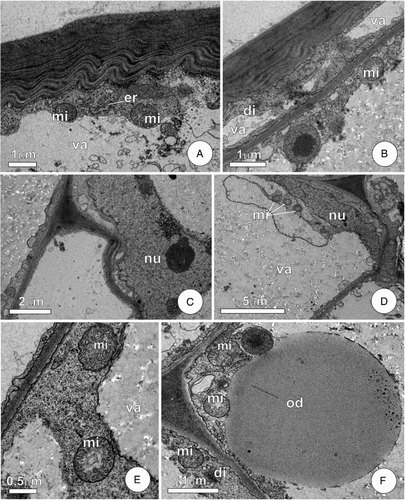
Cells in the central core of FBs possessed elongated nuclei with a variable contour that was sometimes lobed and prominent nucleoli (C–D). Given the large central vacuole, the nucleus occupied a peripheral position. The cytoplasm had a composition similar to that seen in epidermal cells, with mitochondria and the endoplasmic reticulum as predominant organelles. Mitochondria were globular and had well-developed cristae (E). Dictyosomes were also observed, from which sprouted vesicles (F). The peripheral cytoplasm was highlighted by large oil droplets dispersed in the ground cytoplasm (F). In the vacuoles, the presence of flocculated and amorphous material constituted the main morphological characteristics.
Discussion
The data obtained in the present study demonstrate that, in C. pachystachya, FBs are not restricted to trichilium situated at the petiole base, where there are special types of structures called Müllerian bodies. We found additional FBs dispersed on the petiole and leaf blade that were structurally distinct from Müllerian bodies. These additional FBs have previously been described by Rickson (Citation1976) in Cecropia peltata as trichomes termed ‘pearl glands’.
The distinction between FBs and pearl glands is unclear. However, in most case, structures described as pearl glands are randomly distributed throughout the plant body, are composed of highly vacuolated epidermal cells and apparently lack a precise function (Rickson & Risch Citation1984). According to these authors, FBs consist of feeding structures formed generally by epidermal and ground tissues, are filled with reserves and play an important role in ant–plant interactions. The structures that occur in the leaf of C. pachystachya are typical FBs and, due to participation of ground tissue in their composition, are emergences like the Müllerian bodies, although they have a distinct form and contents.
Due to the imprecise definition of pearl glands, studies to better understand their structure and function are important and can promote the redefinition of these structures. According to Paiva et al. (Citation2009), the pearl glands of Vitaceae should more correctly be defined as FBs, because they mediate plant–insect interactions and have a special structure. Our data for C. pachystachya show that structures previously described as ‘pearl glands’ are not trichomes and constitute true FBs. This redefinition proposed here is not simply a terminological question, rather it is of great ecological importance and should stimulate studies that can clarify the role of these structures in ant–plant interactions.
The occurrence of FBs exclusively on the larger veins of the young leaves, as we observed in C. pachystachya, has also been reported by Rickson (Citation1980) for Macaranga hypoleuca (Euphorbiaceae) and by Buono et al. (Citation2008) for Hovenia dulcis (Rhamnaceae). According to Buono et al. (Citation2008), parenchyma cells of the cortical region of the veins are active in the differentiation of FBs. According to these authors, this can explain the absence of FBs on the smaller veins, since they are embedded in the mesophyll and therefore have no cortical cells similar to those observed in major veins. The role of internal tissues in the ontogenesis of FBs is also reported by Rickson (Citation1976) for Müllerian bodies in C. peltata and by Solano et al. (Citation2005) for FBs in Cordia nodosa (Boraginaceae).
In contrast to what has been reported for Macaranga hypoleuca (Rickson Citation1980), Ryparosa kurrangii (Webber et al. Citation2007a), and Hovenia dulcis (Buono et al. Citation2008), in C. pachystachya the FBs also occur on the adaxial surface, although in smaller amounts. According to Buono et al. (Citation2008), FBs on the abaxial side of the leaf are protected from desiccation, but the young leaves of C. pachystachya are protected by a stipule, and FBs are collected soon after losing this protection. Thus, even though they are located on the adaxial surface of the leaf these FBs are not exposed to harmful excessive radiation and heat because they are quite ephemeral and quickly removed by associated ants.
Young and ephemeral organs allocate their resources to the production and maintenance of biotic defences, to the detriment of chemical and physical defences (Folgarait & Davidson Citation1994, Citation1995). For tropical plants, Coley & Barone (Citation1996) observed that the rate of herbivory on young leaves is considerably higher than on mature leaves, which seems to explain the investment in biotic defence of these organs during the expansion phase, as seems to be the case found in this study. In the genus Cecropia, some species do not produce FBs and show high levels of chemical defences in order to compensate for the absence of mutualistic ants (Latteman et al. Citation2014).
In C. pachystachya, in addition to the Müllerian bodies occurring in the trichilium, the FBs appear to be an additional incentive for ants to patrol the entire leaf blade. Rickson (Citation1976) followed the development of Müllerian bodies in C. peltata and found that these structures begin to be produced by the trichilium of a leaf when the immediately younger leaf breaks the stipule. This fact confirms the importance of the foliar FBs, the only resource available to ants during the early stages of leaf development. Rocha & Bergallo (Citation1992) reported that more patrolling by ants decreases the response time of these animals to the presence of a herbivorous threat, thereby reducing the leaf damage.
Lipid droplets were observed in the cytosol of the FBs of C. pachystachya, as well as in the Müllerian bodies of C. peltata (Rickson Citation1976) and the FBs of H. dulcis (Buono et al. Citation2008). The presence of lipid reserves in FBs appears to be widely distributed and has been described in different families, such as Euphorbiaceae, Rhamnaceae and Vitaceae (Rickson Citation1980; Buono et al. Citation2008; Paiva et al. Citation2009). In H. dulcis (Rhamnaceae), Buono et al. (Citation2008) reported the absence of organelles associated with lipid biosynthesis in the FBs during the synthesis of lipid reserves. According to these authors, the absence of organelles classically related to lipid metabolism suggests the role of lipogenic enzymes in the cytosol, similar to that observed by Harwood et al. (Citation1971) in the Ricinus communis (Euphorbiaceae) endosperm. In the C. peltata Müllerian bodies, Rickson (Citation1976) observed that lipid inclusions appear in the cytosol, which seems to reinforce the above observations. It is possible that something similar occurs in the FBs of C. pachystachya, because we did not observe any organelle associated with lipid biosynthesis, even when the lipid reserves were fully developed.
Rickson (Citation1976) reported that the FBs found in blades and petioles of Cecropia possess plastids that produce glycogen and lipid droplets. In ultrastructural analysis we did not observe plastids related to glycogen synthesis in either epidermal or parenchyma cells. The carbohydrate reserve is largely limited to the sugars that we observed within the vacuoles.
The occurrence of sugars in FBs, as we observed in C. pachystachya, was reported by Paiva et al. (Citation2009) for FBs of Cissus verticillata (Vitaceae). It is important to emphasise that there is high similarity between the FBs of these two species, especially regarding the fragility of the cell walls and the turgid, translucent appearance. In ant colonies, carbohydrates seem to be the main fuel for workers (see Davidson Citation1998) and are a key resource for arboreal ants (Davidson et al. Citation2003).
Energy resources are not distributed equally among the distinct developmental stages of ants. It is known that the larval diet of Hymenoptera consists of high-energy foods, while for most species the lowest energy foods are destined for adults. Howard & Tschinkel (Citation1981) reported that in colonies of Solenopsis invicta soluble sugars are eaten by worker ants, while amino acids are preferentially offered to growing larvae and lipids are shared by both developmental stages. In addition, although some ants can consume solid foods (Moreira et al. Citation2015), this is not the rule, whereas in several species the adults just ingest liquid foods and only by trophallaxis (Suarez & Thorne Citation2000). Considering these two sources of nutritive resources in C. pachystachya (FBs and Müllerian bodies), it is possible that they have complementary roles. Therefore, the FBs appear to be destined for feeding adults, while Müllerian bodies (which are rich in glycogen, lipids and proteins) are destined for the larval stage and indirectly used for adults by trophallaxis.
In C. pachystachya we observed that during the search for and protection of FBs, Azteca ants patrol the plant. According to several authors (Koptur Citation1992; Vesprini et al. Citation2003; Oliveira & Freitas Citation2004), this behaviour implies a reduction in the response time to the presence of herbivores and consequently reduces the damage to the plant. Rosumek et al. (Citation2009) observed that the absence of ants results in an increase in the rate of herbivory, and this increase is considerably greater in myrmecophytes.
It is important to note that the presence of energy reserves in these FBs can result in direct benefits to the ant colonies associated with C. pachystachya, as suggested by Byk & Del-Claro (Citation2011). Our results indicate the need to evaluate the significance of these foliar FBs in the diet, and consequently in the fitness, of the associated ant colony, especially considering that these FBs are widespread among species of Cecropia, as pointed out by Rickson (Citation1976).
Acknowledgements
The authors would like to acknowledge the CAPI (UFMG) and the Center of Microscopy (UFMG) for providing the equipment and technical support for experiments involving electron microscopy. The authors would also like to thank DMT Oliveira for her comments and assistance with language revision.
Associate Editor: Dr Janice Lord.
Disclosure statement
No potential conflict of interest was reported by the authors.
ORCID
Elder Antonio Sousa Paiva http://orcid.org/0000-0002-1440-4810
Additional information
Funding
References
- Bronstein JL, Alarcón R, Geber M. 2006. The evolution of plant-insect mutualisms. New Phytol. 172:412–428. doi:10.1111/j.1469-8137.2006.01864.x.
- Brundett MC, Kendrick B, Peterson CA. 1991. Efficient lipid staining in plant material with Sudan Red 7B or fluoral yellow 088 in polyethylene glycol-glycerol. Biotech Histochem. 66:111–116. doi:10.3109/10520299109110562.
- Buono RA, Oliveira AB, Paiva EAS. 2008. Anatomy, ultrastructure, and chemical composition of food bodies of Hovenia dulcis (Rhamnaceae). Ann Bot. 101:1341–1348. doi: 10.1093/aob/mcn052
- Byk J, Del-Claro K. 2011. Ant–plant interaction in the neotropical savanna: direct beneficial effects of extrafloral nectar on ant colony fitness. Popul Ecol. 53:327–332. doi:10.1007/s10144-010-0240-7.
- Coley PD, Barone JA. 1996. Herbivory and plant defenses in tropical forests. Ann Rev Ecol Syst. 27:305–335. doi: 10.1146/annurev.ecolsys.27.1.305
- Davidson DW. 1998. Resource discovery versus resource domination in ants: a functional mechanism for breaking the trade-off. Ecol Entomol. 23:484–490. doi:10.1046/j.1365-2311.1998.00145.x.
- Davidson DW. 2005. Cecropia and its biotic defenses. In: Berg CC, Rosselli PF, editors. Flora Neotropica 94. New York: The New York Botanical Garden; p. 214–226.
- Davidson DW, Cook SC, Snelling RR. 2003. Explaining the abundance of ants in lowland tropical rainforest canopies. Science. 300:969–972. doi:10.1126/science.1082074.
- Dejean A, Petitclerc F, Roux O, Orivel J, Leroy C. 2012. Does exogenic food benefit both partners in an ant-plant mutualism? The case of Cecropia obtusa and its guest Azteca plant-ants. CR Biol. 335:214–219. doi: 10.1016/j.crvi.2012.01.002
- Folgarait PJ, Davidson DW. 1994. Antiherbivore defenses of myrmecophytic Cecropia under different light regimes. Oikos. 71:305–320. doi:10.2307/3546279.
- Folgarait PJ, Davidson DW. 1995. Myrmecophytic Cecropia: antiherbivore defenses under different nutrient treatments. Oecologia. 104:189–206. doi:10.1007/BF00328584.
- Fuente MAS, Marquis RJ. 1999. The role of ant-tended extrafloral nectaries in the protection and benefit of a neotropical rainforest tree. Oecologia. 118:192–202. doi:10.1007/s004420050718.
- Gaume L, McKey D, Anstett MC. 1997. Benefits conferred by “timid” ants: active anti-herbivore protection of the rainforest tree Leonardoxa africana by the minute ant Petalomyrmex phylax. Oecologia. 112:209–216. doi: 10.1007/s004420050302
- Harwood JL, Sodja A, Stumpf PK, Spurr AR. 1971. On the origin of oil droplets in maturing castor bean seeds, Ricinus communis. Lipid. 6:851–854. doi: 10.1007/BF02531217
- Heil M, Fiala B, Kaiser W, Linsenmair KE. 1998. Chemical contents of Macaranga food bodies: adaptations to their role in ant attraction and nutrition. Funct Ecol. 12:117–122. doi:10.1046/j.1365-2435.1998.00158.x.
- Howard JJ, Tschinkel WR. 1981. The flow of food in colonies of the fire ant Solenopsis invicta: a multifactorial study. Physiol Entomol. 6:297–306. doi: 10.1111/j.1365-3032.1981.tb00274.x
- Janzen DH. 1969. Allelopathy by myrmecophytes: the ant Azteca as an allelopathic agent of Cecropia. Ecology. 50:147–153. doi:10.2307/1934677.
- Janzen DH. 1973. Dissolution of mutualism between Cecropia and its Azteca ants. Biotropica. 15:5–28.
- Johansen DA. 1940. Plant microtechnique. New York: McGraw Book Co.
- Karnovsky MJ. 1965. A formaldehyde–glutaraldehyde fixative of light osmolality for use in electron microscopy. J Cell Biol. 27:137A–138A.
- Koptur S. 1992. Extrafloral nectary-mediated interactions between insects and plants. In: Bernays E, editor. Insect-plant interactions. Boca Raton: CRC Press, p. 81–129.
- Latteman TA, Mead JE, DuVall MA, Bunting CC, Bevington JM. 2014. Differences in anti-herbivore defenses in non-myrmecohyte ad myrmecophyte Cecropia trees. Biotropica. 46:652–656. doi:10.1111/btp.12163.
- Longino JT. 1989. Geographic variation and community structure in an ant-plant mutualism- Azteca and Cecropia in Costa Rica. Biotropica. 21:126–132. doi:10.2307/2388703.
- Moreira DDO, Dattilo W, Morais V, Erthal M, Silva CP, Samuels RI. 2015. Diet type modifies ingestion rates and trophallactic exchanges in leaf-cutting ants. Entomol Exp Appl. 154:45–52. doi:10.1111/eea.12254.
- O’Brien TP, Feder N, McCully ME. 1964. Polychromatic staining of plant cell walls by toluidine blue. Protoplasma. 59:368–373. doi:10.1007/BF01248568.
- O’Dowd DJ. 1980. Pearl bodies of a neotropical tree, Ochroma pyramidale: ecological implications. American Journal of Botany. 67:543–549. doi: 10.2307/2442294
- Oliveira PS, Freitas AVL. 2004. Ant-plant-herbivore interactions in the neotropical cerrado savanna. Naturwissenschaften. 91:557–570. doi:10.1007/s00114-004-0585-x.
- Paiva EAS, Buono RA, Lombardi JA. 2009. Food bodies in Cissus verticillata (Vitaceae): ontogenesis, structure and functional aspects. Ann Bot. 103:517–524. doi: 10.1093/aob/mcn237
- Rickson FR. 1976. Anatomical development of the leaf trichilium and Müllerian bodies of Cecropia peltata L. Am J Bot. 63:1266–1271. doi: 10.2307/2441742
- Rickson FR. 1980. Developmental anatomy and ultrastructure of the ant-food bodies (Beccariian bodies) of Macaranga triloba and M. hypoleuca (Euphorbiaceae). Am J Bot. 67:285–292. doi: 10.2307/2442338
- Rickson FR, Risch SJ. 1984. Anatomical and ultrastructural aspects of the ant-food cell of Piper cenocladum C.DC. (Piperaceae). Am J Bot. 71:1268–1274. doi: 10.2307/2443651
- Robards AW. 1978. An introduction to techniques for scanning electron microscopy of plant cells. In: Hall JL, editor. Electron microscopy and cytochemistry of plant cells. New York: Elsevier; p. 343–403.
- Rocha CFD, Bergallo HG. 1992. Bigger ant colonies reduce herbivory and herbivore residence time on leaves of an ant-plant: Azteca muelleri vs. Coelomera ruficornis on Cecropia pachystachya. Oecologia. 91:249–252. doi: 10.1007/BF00317792
- Roland AM. 1978. General preparations and staining of thin sections. In: Hall JL, editor. Electron microscopy and cytochemistry of plant cells. New York: Elsevier; p. 1–62.
- Rosumek FB, Silveira FAO, Neves FS, et al. 2009. Ants on plants: a meta-analysis of the role of ants as plant biotic defenses. Oecologia. 160:537–549. doi:10.1007/s00442-009-1309-x.
- Solano PJ, Belin-Depoux M, Dejean A. 2005. Formation and structure of food bodies in Cordia nodosa (Boraginaceae). Comptes Rendus Biologies. 328:642–647. doi: 10.1016/j.crvi.2005.05.004
- Suárez ME, Thorne BL. 2000. Rate, amount, and distribution pattern of alimentary fluid transfer via trophallaxis in three species of termites (Isoptera: Rhinotermitidae, Termopsidae). Ann Entomol Soc Am. 93:145–155. doi:10.1603/0013-8746(2000)093[0145:RAADPO]2.0.CO;2.
- Stahl E. 1988. Thin-layer chromatography: a laboratory handbook. 2nd ed. Berlin: Springer; p. 1041.
- Vesprini JL, Galetto L, Bernardello G. 2003. The benefical effect of ants on the reproductive success of Dyckia floribunda (Bromeliaceae) an extrafloral nectar plant. Can J Bot. 81:24–27. doi: 10.1139/b03-003
- Webber BL, Abaloz BA, Woodrow IE. 2007a. Myrmecophilic food body production in the understorey tree, Ryparosa kurrangii (Achariaceae), a rare Australian rainforest taxon. New Phytol. 173:250–263. doi: 10.1111/j.1469-8137.2006.01916.x
- Webber BL, Moog J, Curtis ASO, Woodrow IE. 2007b. The diversity of ant–plant interactions in the rainforest understorey tree, Ryparosa (Achariaceae): food bodies, domatia, prostomata, and hemipteran trophobionts. Bot J Linn Soc. 154:353–371. doi:10.1111/j.1095-8339.2007.00651.x.