ABSTRACT
Seed endophytic bacteria and fungi are co-dispersed with seeds and are likely founders of the initial endophytic microbiome in developing seedlings. The effects of the seed microbiome on seedling survival and growth are of particular interest for their roles in protection against pathogens and plant resistance to environmental stress. The fungal pathogen Austropuccinia psidii (G. Winter) Beenken is the causal agent of myrtle rust, which infects more than 480 species of Myrtaceae globally and poses a severe threat to New Zealand endemic species such as Metrosideros excelsa Sol. ex Gaertn. We hypothesised that seedlings of M. excelsa may contain seed-derived microbial communities that may contribute to variation in susceptibility to A. psidii. Culture based methods were used to recover 22 species of fungi (n = 412 isolates) and 16 bacterial taxa (n = 156 isolates) from 1580 M. excelsa seeds collected from 30 trees. Our results indicate broad variation in the isolation frequency and species composition of seed-borne microorganisms among individual trees: while seeds sampled from four trees were free of culturable microorganisms, the other 26 trees accumulated up to eight culturable taxa (both bacteria and fungi) in their seeds, including some species known to be beneficial endophytes or phytopathogens. The most frequently isolated fungal species belong to genera Aureobasidium, Cladosporium, Penicillium, and Pestalotiopsis. These fungi were often isolated from seeds also containing bacteria of the genera Bacillus, Erwinia, and Methylobacterium. At the same time, seeds collected from four trees contained known plant pathogens Colletotrichum aff. fioriniae (Marcelino & Gouli) Pennycook, Neofusicoccum sp., and Robbsia aff. andropogonis (Smith) Lopes-Santos et al., which could affect seed germination. This is the first report of bacterial and fungal communities residing in seeds of endemic New Zealand trees and the potential effects of seed-borne microbiomes on germination.
Introduction
Seed germination, seedling development, and the resilience of seedlings to changing biotic and environmental conditions may be supported by a specific seed-borne microbiome transmitted vertically from the mother plant to seeds (Shahzad et al. Citation2018). Vertical transmissions can repeatedly occur for several generations or be transient, originating from a previous horizontal (from the environment or unrelated individual) transmission of microorganisms to flowers and developing seeds (Rodriguez et al. Citation2009; Rezki et al. Citation2017; Hardoim Citation2019; Jeong et al. Citation2021; Chesneau et al. Citation2022).
Seeds of pioneer and invasive plant species often carry diverse microbial compositions, enabling seedlings to survive in hostile conditions of dry habitats, soils with high salinity or heavy metal content, or on bare rocks (Puente et al. Citation2009; Caddell et al. Citation2019; Martinez-Rodriguez et al. Citation2019; Jeong et al. Citation2021). Such seed-associated microbiomes are adapted to withstand lack of water and high osmotic pressure in dry seeds (Dutta et al. Citation2022; Niza-Costa et al. Citation2022); later microorganisms disseminate in the germinating seedlings, which often have positive impacts on plant development (Compant et al. Citation2010; Truyens et al. Citation2015). However, host-microbial interactions can shift from mutualistic to commensal or pathogenic (Ridout and Newcombe Citation2018), depending on the host conditions (active growth and nutrient production, or stress and prolonged dormancy) and changing environment (Schulz et al. Citation2019; Mengistu Citation2020; Martin and Peter Citation2021). Fruits and seeds often accumulate pathogens, contributing to germination failure and high seedling mortality (Shetty et al. Citation2011; Ridout and Newcombe Citation2018).
Due to an increased understanding of the value of the plant microbiome, recent studies for biosecurity purposes have investigated plant-microbial interactions during the early stages of plant development, and this has included screening for potential biocontrol agents, which can protect the plant against pathogens (Verma et al. Citation2019; Saxena and Strobel Citation2021). For instance, fungi from genera Rhizophagus and Trichoderma are established biocontrol agents for Puccinia graminis Pers. (stem rust of wheat) (El-Sharkawy et al. Citation2018). Endophytic bacteria, Bacillus lentimorbus Dutky, Bacillus cereus Frankland & Frankland, and Klebsiella pneumoniae (Schroeter) Trevisan from leaves and branches of Coffea trees have been identified as effective biocontrol agents against coffee leaf rust caused by Hemileia vastatrix Berk. & Broome (Shiomi et al. Citation2006). Endophytic fungi Aureobasidium leucospermi Crous, Cladosporium tenuissimum Cooke, Papiliotrema flavescens (Saito) Xin Zhan Liu, F.Y. Bai, M. Groenew. & Boekhout, and Phialemoniopsis pluriloculosa Perdomo, Dania García, Gené, Cano & Guarro were isolated from healthy foliage and rust pustules caused by Austropuccinia psidii (G. Winter) Beenken (myrtle rust disease), indicating that some endophytic fungi can parasitise rust fungi (Chock et al. Citation2021). A recent study in Hawaii found that transplantation of the foliar microbiome from host species resistant to myrtle rust, onto the leaves of critically endangered Eugenia koolauensis O. Deg. effectively induced the resistance of E. koolauensis seedlings to the disease (Chock et al. Citation2021).
Myrtle rust disease caused by the pandemic biotype of A. psidii poses a severe threat to New Zealand’s endemic Myrtaceae (Sutherland et al. Citation2020; Toome-Heller et al. Citation2020). Environmental conditions in New Zealand are favourable for effective spread of this fungal pathogen, leading to high infection rates recorded on endemic Lophomyrtus bullata Burret (ramarama) and Metrosideros excelsa Sol. ex Gaertn. (pōhutukawa) as well as several introduced Myrtaceae (Sutherland et al. Citation2020).
Metrosideros excelsa occurs naturally and is extensively planted on North Island, New Zealand, where this tree demonstrates some variation in disease severity (Smith et al. Citation2020). Myrtle rust has particularly been observed in urban areas, predominantly on young trees or garden cultivars of M. excelsa (Sutherland et al. Citation2020). Hypothetically, trees could have genetic resistance to the pathogen (Smith et al. Citation2020), but endophytes within the plant tissues could also influence disease tolerance (Chock et al. Citation2021). However, infection of M. excelsa seedlings with A. psidii in greenhouse conditions yielded only a single resistant plant out of 570 tested, indicating that the genetic resistance of M. excelsa to A. psidii is low and unlikely to explain the observed variation in susceptibility (Smith et al. Citation2020). Thus, the objective of our study was to explore the endophytic microbiome of seeds of M. excelsa to determine if potentially beneficial and protective microbial taxa are present.
In this study, we describe the culturable seed-borne microbiome of M. excelsa from six locations within the Auckland area. Specifically, we addressed the following questions: (i) Which species of endophytic bacteria and fungi can be isolated from the seeds of M. excelsa? (ii) Is there a difference in species richness or isolation frequency of microorganisms in M. excelsa seeds collected from urban areas and regional parks? (iii) Do seeds contain putative plant pathogens or beneficial microbes?
Materials and methods
Study area and site characteristics
M. excelsa seeds were collected in March and April 2022 from six locations in the Auckland region, North Island, New Zealand. Sampling locations were selected based on the presence or absence of myrtle rust, different proximities to the coast, and accessibility. We noted the proximity to the coastline as our observations indicated that M. excelsa growing close to the sea were less affected by myrtle rust than trees located far away from the coastline ().
Table 1. Characteristics of the six locations where seed capsules of Metrosideros excelsa were collected in March-April 2022. Samples were collected from only uninfected trees, but presence of infection was recorded nearby.
Three Regional Parks north of the city centre: Tāwharanui, Wenderholm, and Shakespear, represented rural locations where myrtle rust had not been recorded at the time of sampling (although it subsequently arrived) (; ). For comparison, three sites in Auckland city: Maungakiekie / One Tree Hill, Tamaki Drive, and the University of Auckland campus, represented urban locations with high anthropogenic vegetation management and where trees affected by myrtle rust occurred at time of sampling.
Figure 1. A, Metrosideros excelsa seed capsules, B, study area within New Zealand (marked as white star), and C, locations sampled in the Auckland region. 1 is Tāwharanui, 2–Wenderholm, 3–Shakespear, 4–Tamaki Drive, 5–University of Auckland, and 6–One Tree Hill. Only mature but unopened seed capsules were collected for determination of seed microbiomes.
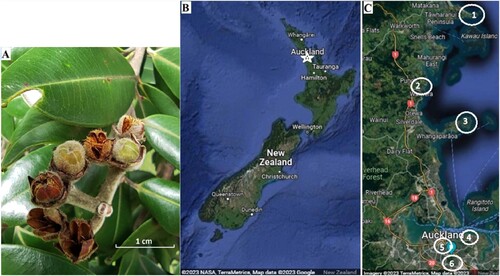
Seed capsules collection, surface sterilisation, and seed extraction
Five uninfected trees with ripe seed capsules were chosen in each location, and intact capsules with mature seeds were collected (). Five closed seed capsules were taken from one raceme, and ≥ 25 seed capsules were randomly collected from the lower branches around each tree to recover the culturable seed-borne microbial community.
The sampled plant material was processed in the laboratory within 48 h of collection. The collected capsules were surface sterilised by immersion in 70% ethanol for 30 s, then transferred to 2% sodium hypochlorite solution for two minutes, followed by a double wash in sterile water for two minutes each time (Sahu et al. Citation2022). After that procedure, seed capsules were again quickly dipped in 70% ethanol and left in a sterile laminar hood until ethanol evaporated; then, capsules were dissected using a scalpel in a sterile Petri dish and seeds were extracted. To avoid cross-contamination, the scalpel was sterilised in 70% ethanol and flamed after each seed capsule. Seeds from each tree were placed in sterilised 400 ml Systema (Sistema® Plastics, New Zealand) containers and stored in a fridge at 4°C.
Recovery and isolation frequency of culturable microorganisms
The initial isolation of bacteria and fungi from the seeds was performed on three standard media: Potato Dextrose Agar (PDA, Difco, Becton, Dickinson, and Company), Reasoner's 2A agar (R2A, Difco, Becton, Dickinson, and Company), and Nutrient Agar with Yeast Extract (YNA, Difco, Becton, Dickinson, and Company). A recent study of different cultivation techniques has shown that specifically tailored media could significantly increase isolation yield and species richness (Gerna et al. Citation2022). Complex isolation media, PDA or YNA, contain high amounts of nutrients such as saccharides and amino acids; therefore, most fast-growing bacterial strains often overgrow endophytic bacteria, which cannot compete with dominant species on media (Holland et al. Citation2000). In contrast, minimal microbial growth media such as M9 contain strict amounts of salts and nutrients, selectively excluding opportunistic bacteria while enabling the growth of only some endophytic prokaryotes (Gerna et al. Citation2022). Eevers et al. (Citation2015) found that extracts from host tissues included in media could also improve isolation outcomes. Hence, two further media, M9 minimal medium in 1.5% agar (M9, Sigma-Aldrich) and M9 salts in 1.5% agar with 0.5% methanol (Holland et al. Citation2000), were also employed to isolate slow-growing bacteria. In addition, an infusion of host leaves was prepared to increase the recovery yield of fastidious species: 20 g of fresh M. excelsa leaves were cut into small pieces and autoclaved in 0.5 L of sterile water. The resulting leaf infusion was poured onto all types of agar media and considered sterile if no growth was observed after two weeks of incubation.
Since only around 10–20% of M. excelsa ovules develop into fertile seeds resulting in approximately 50–60 viable seeds per capsule (Schmidt-Adam et al. Citation1999, Citation2002), we pooled the seeds from seed capsules of each tree and obtained 30 bulk samples, representing 30 trees, respectively. No less than 100 seeds from each bulk sample were soaked in sterile water overnight in individual sterile Petri dishes and crushed with a pestle and mortar. The sterile leaf infusion prepared earlier was added to the crushed seeds, which were then placed onto agar plates with each medium: PDA, R2A, YNA, M9, and M9 with methanol, respectively, and incubated for at least two months at an approximate temperature of 18–20°C. Initially, 48 drops of infusion with crushed seeds from each tree were placed on the cultivation media, and the Petri dishes were monitored every two days to check the presence of microbial growth. When microbial growth was not observed after two weeks of incubation, the entire procedure was repeated with another group of seeds from the same tree. The bacterial colonies and fungal hyphae that emerged from the seeds were transferred into new Petri dishes and coded with a number, reflecting the location and sampled tree, e.g. Tw1, W1, W2, etc (). The isolation frequency was calculated as the number of emerged colonies / the total number of crushed seeds in infusion drops × 100%.
Identification of isolated microorganisms
The obtained colonies were subcultured to ensure purity, then grouped into morphotypes based on their morphological features in the case of fungi. Yeasts were separated from bacteria after microscopic examination of isolates. Final identification was performed using DNA sequencing of taxonomically useful gene regions as endophytic fungi are often difficult to identify based solely on their morphology (Ko Ko et al. Citation2011). Bacterial colonies were not morphotyped and sequenced directly due to the lack of significant morphological features.
DNA was extracted from the bacterial and fungal isolates using the REDExtract-N-Amp™ Plant PCR Kit (Sigma-Aldrich) following the manufacturer’s protocol. The taxonomic identification of putative fungal species was performed by amplification and sequencing their nuclear ribosomal internal transcribed spacer (ITS) regions, according to the methodology developed by Johnston et al. (Citation2017). The amplification and the following sequencing of the ITS region were performed using the universal primers ITS1F and ITS4 (White et al. Citation1990; Gardes and Bruns Citation1993). For the identification of bacteria, the 16S rRNA gene was amplified using universal primers fD1 and rP2 (Weisburg et al. Citation1991). The obtained sequences were analysed, manually trimmed, and assembled de novo using Geneious R11 (Biomatters Limited, Auckland, New Zealand, USA). Then sequences were compared with deposited sequences in the GenBank database of the National Center for Biotechnology Information (NCBI) using the Basic Local Alignment Search Tool (BLAST) (https://blast.ncbi.nlm.nih.gov/Blast.cgi), and the taxonomic level was assessed as the nearest match to the top results in GenBank with the highest percent identity. The newly generated sequences were submitted to GenBank under accession numbers: OR388558, OR472901-OR472925, OR472927, OR499116–OR499165 (Sayers et al. Citation2019).
Seed germination test
Germination tests were performed after nine months of seed storage to assess seed viability and presence of seed-borne pathogens. Seeds (0.1 g; 130–150 viable seeds (Schmidt-Adam et al. Citation1999, Citation2002)) extracted from sterilised capsules of each tree were placed on 1.5% water agar (Difco, Becton, Dickinson, and Company) in individual Petri dishes. Germination conditions were as follows: day/night temperature 22/18°C with 13 h under natural daylight and 11 h in dark. Petri dishes were observed every three days for a month and seeds were considered germinated when the radicle protruded 2 mm (Bewley Citation1997). Some seeds and seedlings appeared to be killed by pathogens during germination, and these were transferred to media for microbial isolation and sequencing for confirmation as previously described.
Statistical analysis
All statistical analyses were performed in Past 4.0 software (Hammer et al. Citation2001). Welch's t-test was employed to compare the difference in species richness between the urban locations and regional parks. ANOVA and Tukey's Honest Significant Differences test were employed to evaluate differences among each sampled location. The Bray–Curtis index was used to evaluate the dissimilarity of microbial compositions among individual trees based on isolation frequencies for microbial taxa isolated from each tree, and then non-metric multidimensional scaling (NMDS) ordination and neighbour joining clustering based on the Bray–Curtis index were applied to visualise the results. Trees that did not yield any isolations on media were excluded from the analysis.
Assessment of ecological role and application potential of isolated microorganisms
The ecological roles of isolated microorganisms and their biocontrol properties or potential contribution to the development of seedlings were assessed by literature searches. The current taxonomic status of bacteria was checked in the List of Prokaryotic names with Standing in Nomenclature (Parte et al. Citation2020). Data about species occurrence in New Zealand and their properties was primarily gathered from the Biota of New Zealand database (https://biotanz.landcareresearch.co.nz), Centre for Agriculture and Biosciences International (https://www.cabidigitallibrary.org), Fungal Planet description sheets of the International Mycological Association (http://ima-mycology.org), and Mycobank (https://www.mycobank.org).
Results
Recovery and isolation frequency of culturable microorganisms
Of the approximately 1580 seed samples that were plated, 412 fungal isolates (from seeds of 25 trees) and 156 bacterial colonies (from seeds of 16 trees) were recovered. Seed-associated microorganisms were found in all sampled locations; however, no microbial growth was observed in seeds collected from trees Tw1, TD4, TD5, and U1 (located at Tāwharanui, Tamaki Drive, and the University of Auckland, respectively) after two months of incubation. The experiment was repeated three times with consistent results; hence, these seeds were deemed free of a culturable microbiome.
Each of the individual M. excelsa trees differed in the isolation frequency of recovered microorganisms, which ranged from 0% to 83% for bacteria and from 0% to 85% for fungi (). Overall fungal species richness in rural and urban locations was not significantly different (a, Welch’s t-test, P = 0.48). A general comparison of isolation rate of culturable seed-borne mycobiome between rural and urban locations also did not show a significant difference between the locations (P = 0.68, b). For the overall isolation rate of all microorganisms, bacteria and fungi, trees sampled from Wenderholm Regional Park had the highest values, while Tāwharanui displayed the lowest values (c). Results of an ANOVA on isolation rate amongst sites returned a P-value of 0.054, but Tukey’s Honest Significant Differences test showed that only Tāwharanui and Wenderholm were statistically different from each other (P = 0.046).
Figure 2. Isolation frequency of the seed-borne bacteria and fungi from individual M. excelsa trees. Error bars indicate standard error of the mean. Isolation frequency was calculated as the number of seed samples containing bacteria or fungi / total number of plated samples. Fungi are represented by blue bars and bacteria by red. Individual trees are marked according to locations: W–Wenderholm, Sh–Shakespear, Tw–Tāwharanui, TD–Tamaki Drive, U–University of Auckland, OTH–One Tree Hill, and W1, W2, W3–trees one, two, three sampled in Wenderholm, respectively.
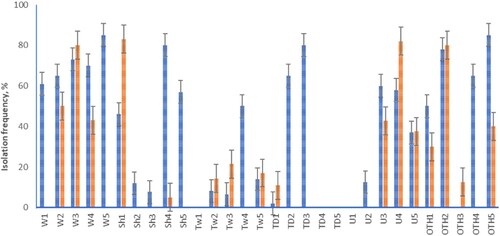
Identification of isolated microorganisms
No more than two bacterial taxa were isolated from the seeds of most trees except W2 and TD1, which yielded three and four taxa respectively (). The highest species richnesses of seed-borne fungi were recorded for trees located in Wenderholm Regional Park, as well as for trees Tw4 and OTH1. Generally, some individual M. excelsa contained richer microbial communities in their seeds than nearby trees, resulting in the large variation observed among trees in each location. Based on sequence results, the recovered microorganisms included 16 bacterial taxa and 22 species of fungi ( and ). Bacterial endophytes of the phylum Bacillota (Firmicutes) were represented by eight species dominated by the genus Bacillus. The second most common bacterial genera belonged to the phylum Pseudomonadota (Proteobacteria) represented by Erwinia, Methylobacterium, Pantoea, Robbsia, and Stenotrophomonas, and members of Actinomycetota (Actinobacteria) included genera Curtobacterium, Kocuria, and Mycolicibacterium (). Bacterial taxa were haphazardly distributed among individual trees: Methylobacterium sp. was isolated from four trees, Erwinia aff. billingiae Mergaert et al. from four other trees, and the rest of the bacteria came from single or two trees.
Figure 4. Species richness of the seed-borne fungi and bacteria from individual M. excelsa trees. Error bars indicate standard error of the mean. Fungi are represented by blue bars and bacteria by the red. Site codes as per .
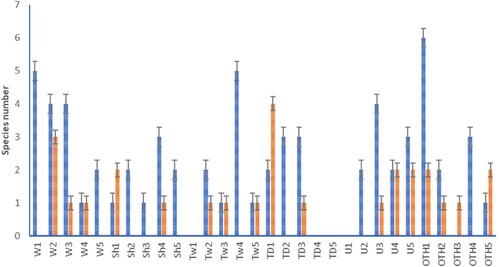
Table 2. Nearest matches of endophytic bacteria isolated from Metrosideros excelsa seeds. Individual trees are marked according to locations: W–Wenderholm, Sh–Shakespear, Tw–Tāwharanui, TD–Tamaki Drive, U–University of Auckland, OTH–One Tree Hill, and W1, W2, W3–trees one, two, three sampled in Wenderholm, respectively. Species identities reflect GenBank IDs based on sequence similarity values.
Table 3. Nearest matches of endophytic fungi isolated from M. excelsa seeds. Individual trees are marked according to locations: W–Wenderholm, Sh–Shakespear, Tw–Tāwharanui, TD–Tamaki Drive, U–University of Auckland, OTH–One Tree Hill, and W1, W2, W3–trees one, two, three sampled in Wenderholm, respectively. Species identities reflect GenBank IDs based on sequence similarity values.
Fungi from the genera Aureobasidium, Cladosporium, Colletotrichum, Cytospora, Sydowia, Penicillium, and Pestalotiopsis were the most abundant in seeds, but their community composition varied greatly among individual trees (). Aureobasidium sp., Cladosporium aff. perangustum Bensch, Crous & U. Braun, and Penicillium aff. glabrum (Wehmer) Westling were isolated from all the study locations except Tamaki Drive (). Based on sequence results, the genus Cytospora was possibly represented by two species: cultures OTH1 and W5 matched leaf eDNA detected previously only on Rangitoto Island, while the other three cultures obtained from OTH4, U4, TD2 possibly belong to introduced exotic species (P. Johnston, pers. comm.).
Figure 5. Relative abundance of the frequently isolated fungal endophytes in individual Metrosideros excelsa trees. Each bar represents the whole mycobiome recovered from an individual tree, and relative abundances of the seven most common fungal species were calculated for each tree as a percentage of isolation. Fungal genera isolated from only one tree or with average isolation rate lower than 5% are shown collectively as “others”. Site abbreviations as per .
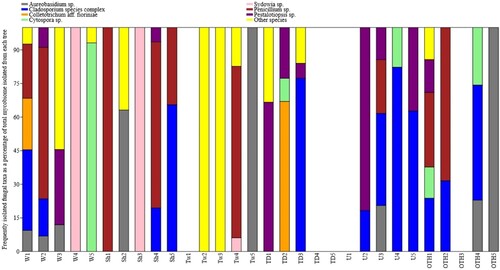
Although separate selective media were used for bacterial and fungal isolation, seeds from certain trees did not yield any bacteria on R2A, YNA, and M9 media. In contrast, samples from other trees produced primarily bacteria, and fungal isolations on PDA were scarce or absent. Several individual trees harboured unique species that were not obtained from any other sources. For example, seeds collected from TD1 contained an unknown fungal species from the Dothideomycetes together with bacteria Bacillus infantis Ko et al., Kocuria sp., and Peribacillus (Brevibacterium) sp. that were not found in other trees ( and ).
The non-metric multidimensional scaling (NMDS) ordination visualisation of the dissimilarities amongst trees showed that trees from different locations did not necessarily group together, nor did the sites group by whether they were urban or rural (). A neighbour-joining tree based on Bray–Curtis similarity index showed that trees Tw2, OTH3, Tw3, TD1, Sh2, Tw5, Sh3, and U2 clustered together based on the low isolation rates of both bacteria and fungi, while second group (TD3, OTH4, Sh5, W1, U3, U5, OTH1, OTH2, and Tw2) comprise trees whose seeds accumulated fungi predominantly from Cladosporium and Penicillium genera (Supplementary Information ). The third cluster included trees that did not contain Cladosporium in their seeds; Sh1, U4, and W3 displayed a greater bacterial isolation rate than fungi, which were represented by mostly Penicillium and Pestalotiopsis; W2 and Sh4 accumulated Penicillium together with putatively pathogenic bacteria (Curtobacterium aff. flaccumfaciens (Hedges) Collins and Jones and Robbsia aff. andropogonis (Smith) Lopes-Santos et al., respectively); and W5 was similar to TD2 based on the absence of culturable bacteria and the presence of Cytospora in seed samples.
Figure 6. Nonmetric multidimensional scaling ordination of the microbial composition in seeds from individual trees of Metrosideros excelsa based on Bray-Curtis distance metric (stress = 0.13). Each point represents a microbial community recovered from the seeds of one tree. Trees U1, TD4, TD5, Tw1 did not yield culturable microbiomes on media and were removed from the analysis. Urban locations: red triangle; rural locations: green diamond. Site abbreviations as per .
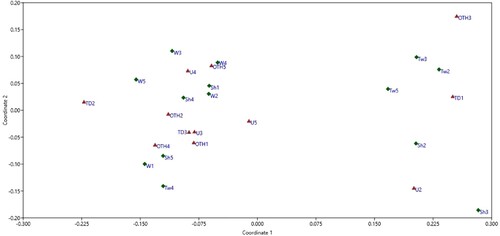
Seed germination test
Viable M. excelsa seeds started to germinate within seven days, and during the second week, seedlings opened cotyledons and developed dense root hairs. Seeds collected from trees W5, Tw4, U5, and OTH4 showed the highest germination rate, 93–100%, which is typical for freshly collected seeds of M. excelsa (Schmidt-Adam et al. Citation2002). Seeds from trees W4, Sh2, Tw2, and Tw3 germinated at a rate of 68–81% (). Germination lower than 20% was observed among seeds from trees Sh4, TD1, TD2, OTH3, and OTH5. All the seeds collected from tree W1 were overgrown on the plates and likely killed by fungi. These putative pathogens were isolated on media and identified by Sanger sequencing as Colletotrichum aff. fioriniae (Marcelino & Gouli) Pennycook and Neofusicoccum sp. Also, C. aff. fioriniae was isolated from non-viable TD2 seeds, and non-viable seeds from Sh4 accumulated a bacterium Robbsia aff. andropogonis. Based on sequence results, ungerminated seeds from the Sh1 tree contained Pantoea sp. related to P. ananatis (Serrano) Mergaert et al. which could probably cause germination failure.
Ecological roles and application potential
Most microbial species isolated from M. excelsa seeds are known seed-borne endophytes that have been associated with other plant hosts (Supplementary Information Table 1). Among bacterial taxa, 12 species (75% of total bacteria isolated) were identified as putative beneficial endophytes with well-documented activity against phytopathogens or/and growth-promoting properties, which include plant hormone production, nitrogen acquisition, nitrogen fixation, phosphorus solubilising, and acquisition of other essential micronutrients (Supplementary Information Table 1). At least nine species of bacteria are known as fungal antagonists, capable of inducing resistance to pathogens in various crops (Supplementary Information Table 1). Methylobacterium, Mycolicibacterium, and Paenibacillus can positively interact with beneficial fungal endophyte Serendipita ( = Piriformospora) indica, boosting plant protection against pathogenic fungi (Supplementary Information Table 1). Phytopathogenic bacteria were also represented in M. excelsa seeds by Pantoea sp. closely related to P. ananatis, and Robbsia aff. andropogonis.
Nine fungal taxa (41% of total fungi isolated) have been effectively applied as biocontrol agents in various crops (Supplementary Information Table 1). In addition, Papiliotrema flavescens (Saito) X.Z. Liu, F.Y. Bai, M. Groenew. & Boekhout has been isolated from healthy foliage and rust pustules of A. psidii and, therefore, considered to be a putative hyperparasite of A. psidii (Chock et al. Citation2021). In contrast, two fungal taxa, Colletotrichum aff. fioriniae and Neofusicoccum sp. are known as important postharvest phytopathogens that cause fruit rot and seed damage to various trees, including Eucalyptus and Syzygium spp. (Supplementary Information Table 1). The rest of the isolated fungi were represented by endophytes without any known functions.
Discussion
This research provides the first insight into the composition of culturable bacterial and fungal communities of M. excelsa seeds and their natural variation among individual trees. The culturable microbial diversity detected was similar to that recovered from the seeds of other Myrtaceae species (Lupo et al. Citation2001; Ferreira et al. Citation2008; Wicaksono et al. Citation2016; Hanin and Fitriasari Citation2019), even though research on endophytic communities inhabiting the Myrtaceae is scarce and mainly dedicated to commercially important trees such Eucalyptus spp., Leptospermum scoparium J.R.Forst. et G.Forst. (mānuka) and Psidium guajava L. (common guava).
The results indicate that the culturable seed-associated bacteriome of M. excelsa resembles that recovered from Eucalyptus (Ferreira et al. Citation2008). Bacillus, Paenibacillus, and Methylobacterium were isolated from Eucalyptus seeds, while Curtobacterium, Erwinia, and Pantoea were recovered from stems of several Eucalyptus species (Ferreira et al. Citation2008; Procópio et al. Citation2009). Four Bacillus species were isolated from fruits of Syzygium cuminii (L.) Skeels. from West Java (Indrawati et al. Citation2021); Bacillus sp. and Stenotrophomonas sp. were associated with leaves and tissue culture of Plinia peruviana (Poir.) Govaerts (jaboticaba) in Brazil (Queiroz et al. Citation2020). Erwinia billingiae and Paenibacillus sp. were also found in vegetative organs of Leptospermum scoparium, a species of New Zealand indigenous Myrtaceae (Wicaksono et al. Citation2016; Wicaksono et al. Citation2017).
It should be highlighted, however, that members of Bacillus and Paenibacillus are widespread tree endophytes that frequently occur in woody tissues and have often been isolated from reproductive organs and seeds (Ulrich et al. Citation2008; Chebotar et al. Citation2016; Newcombe et al. Citation2023). Also, Methylobacterium has been recovered from a wide variety of plants and their seeds (Dourado et al. Citation2015; Holland Citation2019; Roodi et al. Citation2020). Based on these data, we may conclude that the seeds of M. excelsa contain endophytic bacteria typically found in other woody plants. Nevertheless, our results demonstrated that a few bacterial taxa were isolated only from M. excelsa growing near the coastline, and similar bacteria have been previously associated with saline habitats and obtained from halophytes elsewhere. For instance, Methylobacterium was abundant in the foliage of halophytes and drought-tolerant species from Greece (Genitsaris et al. Citation2020), and we isolated Methylobacterium primarily from seeds of trees located close to the sea in Wenderholm and Tawharanui parks, while in the city this genus was found in only one tree near the University of Auckland. Some species of Methylobacterium are known as promoting plant growth and nutrient uptake under salt stress conditions or drought (Egamberdieva et al. Citation2015; Chanratana et al. Citation2017). Kocuria is another genus reported as colonising halophytes and conferring salt tolerance to the host (Zhao et al. Citation2016; Afridi et al. Citation2021); we isolated this bacterium only from the TD1 tree that is growing two metres from the sea. In addition, seeds collected from TD1 harboured Peribacillus (Brevibacterium) and Stenotrophomonas, which are also reported as endophytes of the New Zealand indigenous halophyte Tetragonia tetragonioides (Pall.) Kuntze (New Zealand spinach) (Egamberdieva et al. Citation2021). Notably, M. excelsa trees in coastal areas show the lowest incidence of myrtle rust disease, but the reasons for this observed resistance have not yet been determined. This trend continued since sampling: during the extremely wet 2023 summer season, trees were observed developing severe myrtle rust infection in the inner city of Auckland; however, those growing at the Auckland waterfront and roads close to the sea were less affected (pers. obs.). Taking into account that sampled trees growing in close proximity to the ocean appear to harbour bacteria associated with halophytes and the apparent low percentage of disease, it may be possible that these bacteria enhance tolerance to pathogenic fungi, but this assumption needs further investigation.
The seed mycobiome of M. excelsa also comprised the same genera as Eucalyptus seeds; the culture-based approach recovered Alternaria, Aureobasidium, Cladosporium, and Cytospora in the seeds of E. globulus Labill. (Lupo et al. Citation2001), and Alternaria, Cladosporium, Hormonema (asexual morph of Sydowia), Neofusicoccum, Penicillium, Pestalotiopsis, Sydowia, and Valsa (sexual stage of Cytospora) were observed by next-generation sequencing in E. grandis seeds (Jimu et al. Citation2016). Fruits of Syzygium cuminii also harboured species from Colletotrichum, Neofusicoccum, and Pestalotiopsis (Hanin and Fitriasari Citation2019). However, fruit mesocarp and seeds of S. cuminii have yielded different fungal taxa (Hanin and Fitriasari Citation2019), and similar results have been obtained for E. globulus, whose seeds contained five species of fungi that did not occur in flowers and seed capsules (Lupo et al. Citation2001). Although the exact mechanism creating niche specialisation in seeds is unknown, fungi may enter ovaries via pollen tubes and gametes, as proposed by Hodgson et al. (Citation2014) as a common type of transmission for Alternaria alternata (Fr.) Keissl. and Cladosporium sphaerospermum Penz. On the other hand, the vertical transmission of Aureobasidium and Cladosporium from flower buds to seed capsules was recently confirmed by next-generation sequencing for L. scoparium (Larrouy et al. Citation2023). If fungi enter ovules through the seed placenta directly via the xylem of fruit buds, they would accumulate in seeds, but not in the pericarp, and during fruit development the pericarp will be colonised by other species, arriving from the environment. As possible future research, the dissection of seeds, their microscopic examinations, and culturing would yield more information on the fine detail of niche specialisation.
The ability of phytopathogens to colonise fruits and seeds may have adverse effects on seed germination and survival of the seedlings. Evidence for vertical transmission of pathogens was obtained for E. globulus, where seed-borne Cytospora chrysosperma (Pers.) Fr., a latent fungal pathogen, colonised tissues of emerged seedlings, making them prone to environmental stresses (Lupo et al. Citation2001). Our results indicate that putative pathogenic bacteria Pantoea sp. and Robbsia aff. andropogonis, as well as fungi Colletotrichum aff. fioriniae and Neofusicoccum sp., were associated with M. excelsa seeds, which showed germination failure or high mortality during the germination test. It is unclear if these pathogens were vertically transmitted from the mother trees. If vertical transmission of pathogens can occur every fruiting season, the seed collection from such trees for reforestation purposes would provide contaminated material–that may inadvertantly co-disperse pathogens. Treatment with systemic fungicides could cure the seeds, but these fungicides may have adverse impacts on seedling development through depletion of beneficial taxa or direct phytotoxic effects.
In contrast to our hypothesis that trees in rural locations would have greater species richness than in the city, we did not find significant differences in the numbers of cultured microbial species between rural and urban locations combined. Instead, individual trees appeared to be the primary driver of microbial assembly, with some trees from different locations harbouring similar endophytic species within the seeds, and some trees located close together having a dissimilar culturable microbiome. This finding does not correspond to previous studies (Matsumura and Fukuda Citation2013; Abrego et al. Citation2020), which showed that urbanisation has an adverse impact on the species richness of endophytic fungi, and anthropogenic disturbance often shifts fungal endophytic communities of urban trees towards a prevalence of wood-decay pathogens (Robles et al. Citation2015). Our data showed that individual M. excelsa trees with seed-borne pathogens Colletotrichum aff. fioriniae and Neofusicoccum sp. occurred in both urban locations and regional parks. Moreover, only two planted trees in Shakespear Regional Park contained putative pathogenic bacteria Pantoea sp. and Robbsia aff. andropogonis, which were the likely cause of seed rot during the germination trial.
A high species variation among sites, which correlated with temperature and rainfall, was observed in Metrosideros polymorpha, a Hawaiian relative of M. excelsa (Zimmerman and Vitousek Citation2012). This study found that the foliar endophytic fungal communities of trees in a low-elevation wet site accumulated the most diverse mycobiome whereas M. polymorpha at higher elevations had less taxa due to lower temperatures and drier conditions (Zimmerman and Vitousek Citation2012). We did not observe this pattern potentially because our study locations are situated at sea level and low hills in northeastern and central parts of Auckland, where annual average temperatures and precipitation are evenly distributed (Chappell Citation2013). However, Zimmerman and Vitousek (Citation2012) showed that foliar fungal communities were diverse among M. polymorpha trees within each sampled location, similar to our findings for M. excelsa seed-borne fungi.
The similarity of some microbiomes amongst trees across our sampled locations could possibly be explained by a provenance or common nursery origin of certain sampled M. excelsa trees. Unfortunately, Auckland Council (the manager of the regional parks and responsible for the Tamaki Drive Street trees) did not have records for the sources of those trees sampled in our study that they planted; but some anecdotal information on their provenances does exist. For instance, many M. excelsa planted in Auckland Regional Parks from the early seventies were selections by Bert Blumhardt who collected seeds or cuttings from anything unusual, including M. excelsa with different flower colours and variegated clones of Metrosideros, which were planted at Wenderholm and Shakespeare Regional Parks but not at Tāwharanui (J. Hobbs, pers. comm.). Trees were probably selected from different regions that could then result in the mixture of different genotypes (M. Maitland, pers. comm.). For urban plantings, many cultivars were selected from wild populations and distributed around Auckland by Graeme Platt, a pioneer of the New Zealand plant industry. This indicates that trees planted on Tamaki Drive and One Tree Hill might likely include attractive selections of natural hybrids of M. excelsa with M. robusta or M. kermadecensis (E. Miller, pers.comm.), although we aimed not to sample hybrids. Juvenile M. excelsa trees may have initially acquired a microbiome from other species growing in a plant nursery or another source area, and retained that microbial assembly following planting around Auckland city and further afield in the regional parks. Plant nurseries are often viewed as artificially assembled plant communities that incorporate a high number of species, which may act as potential sources of new microbial associations for each other because these plants never occur together in the natural environment (Strobel Citation2018; Wolfe et al. Citation2018; Gioia et al. Citation2020).
Research on L. scoparium indicates support for the nursery hypothesis as in a comparison of foliar fungal diversity of natural and planted stands of L. scoparium a significant difference in species composition was observed and the species richness was lower in all planted stands, with similar species compositions of fungi isolated from individual trees within every planted area (Johnston Citation1998). In addition, latent pathogens were present in high numbers in all the planted stands, in spite of their close proximity to naturally regenerating trees that contained healthier and more diverse mycobiomes (Johnston Citation1998).
To our knowledge, this is the first attempt to evaluate the potential beneficial properties and application potential of the seed-borne microbiome of a New Zealand indigenous tree. Although we have based our assessment on the most common roles of the species we discovered, some caution needs to be exercised in assuming particular trophic modes of isolated microorganisms. Many of them can exist as asymptomatic endophytes in living plant tissues that may shift to a saprotrophic lifestyle. However, the assessment of literature records on the phytobeneficial potential of isolated species shows that 75% of all the isolated bacteria and 41% of the isolated fungi may positively influence plant development or protect against pathogens.
Metrosideros excelsa is a coastal pioneer tree, naturally growing on cliffs and lava fields (Simpson Citation2005). Although the dust-like seeds of this tree bear minuscule endosperms with low nutrient content, seedlings growing on lava fields can survive hot summer months in crevices containing a small amount of plant debris or moss (Whiting Citation1986). The seed-borne microbiome may facilitate the survival ability of such seedlings. Literature records indicate that species of Bacillus, Kocuria, Methylobacterium, and Paenibacillus can fix nitrogen, facilitate nutrient acquisition, and produce plant hormones, promoting plant growth (Supplementary Information Table 1). Mutualistic relationships with diazotrophic and plant growth-promoting bacteria may be highly beneficial during the early stages of seedling development due to increased vigour and resistance to environmental stresses, including pathogen attack. In addition, fungal endophytes of the genera Aureobasidium, Cladosporium, Papiliotrema, and Penicillium may potentially enhance seedling resistance to environmental stresses or act as antagonists of pathogenic fungi (Supplementary Information Table 1). These putative properties of recovered endophytes will need further testing in M. excelsa seedlings in inoculation experiments to find the most promising species for plant protection.
Our results show that seeds of certain trees accumulate several culturable bacterial and fungal taxa in greater abundance than other trees of the same species. Seed-borne endophytic fungi are traditionally studied separately from endophytic bacteria; however, recent studies indicate that endophytic fungi and bacteria are found in equal abundance, and their interactions can be defined as a “healthy balance of antagonisms” (Schulz et al. Citation2019). In a recent study, Newcombe et al. (Citation2023) found that several seed-borne Bacillus species can significantly inhibit the growth of seed-associated fungi while facilitating the growth of other fungal taxa in double-culture interaction assays on agar media. However, the finding that Bacillus is the most common and dominant genus of bacterial seed endophytes (Newcombe et al. Citation2023) may be explained as a cultivation bias originating from using only potato dextrose agar to isolate bacteria; the use of cultivation media with different nutrient compositions could shift the observed isolation frequency and give priority to other taxa (Eevers et al. Citation2015; Gerna et al. Citation2022).
It has been demonstrated that beneficial endophytic bacteria positively influence the mycobiome, and some bacteria can significantly enhance the biocontrol activity of endophytic fungi against phytopathogens (Furtado et al. Citation2019; del Barrio-Duque et al. Citation2020). In experiments where Solanaceae plants were co-inoculated with the endophytic fungus Serendipita indica and several bacterial species, this complex microbiome was found to provide better protection against pathogenic Fusarium oxysporum Schltdl. and Rhizoctonia solani J.G. Kühn than after inoculation with only S. indica (del Barrio-Duque et al. Citation2019; del Barrio-Duque et al. Citation2020; Vyshakhi and Anith Citation2021). Considering this accumulating body of knowledge, we propose testing bacteria and fungi isolated from the same trees as a community in co-inoculation trials to understand their cumulative effects in planta.
In conclusion, M. excelsa seeds contain culturable microorganisms and the final seed microbial assembly may depend on factors affecting the individual host tree. The broad variation in the taxonomic diversity of microorganisms recovered from individual trees indicates there may be a large amount of hidden microbial diversity; therefore next-generation sequencing of the whole seed-borne microbial community should be considered as a next step for the exploration of complex plant-microbial interactions and their influence on host development and pathogen resistance.
Supplemental material Fig. 1 - Neighbour-joining tree
Download JPEG Image (76.8 KB)Supplementary information
Download MS Word (298.9 KB)Acknowledgements
The authors acknowledge and thank Ngāti Manuhiri, Ngāti Whātua Ōrākei, Tūpuna Maunga Authority, and Auckland Council for gaining consent and providing mana whenua approval to collect seed capsules from trees on sites they administer. We are grateful to Dr Renee Johansen for her excellent management of this project and Dr Jo Peace for fieldwork assistance. We are thankful for the assistance that Dr Chantal Probst and Duckchul Park provided in the molecular laboratory at Manaaki Whenua–Landcare Research. We also thank Diane Lee and Rose Williams for their help with culture storage in the International Collection of Microorganisms from Plants (ICMP) hosted by Manaaki Whenua–Landcare Research. Finally, we thank the two reviewers for their valuable comments on the initial manuscript.
Disclosure statement
No potential conflict of interest was reported by the author(s).
Additional information
Funding
References
- Abrego N, Crosier B, Somervuo P, Ivanova N, Abrahamyan A, Abdi A, Hamalainen K, Junninen K, Maunula M, Purhonen J, et al. 2020. Fungal communities decline with urbanization-more in air than in soil. ISME J. 14(11):2806–2815. doi:10.1038/s41396-020-0732-1.
- Afridi MS, Van Hamme J, Bundschuh J, Sumaira N, Khan MN, Salam A, Waqar M, Munis MFH, Chaudhary HJ. 2021. Biotechnological approaches in agriculture and environmental management–bacterium Kocuria rhizophila 14ASP as heavy metal and salt-tolerant plant growth-promoting strain. Biologia. 76(10):3091–3105. doi:10.1007/s11756-021-00826-6.
- Bewley JD. 1997. Seed germination and dormancy. Plant Cell. 9(7):1055–1066. doi:10.1105/tpc.9.7.1055.
- Caddell D, Deng S, Coleman-Derr D. 2019. Role of the plant root microbiome in abiotic stress tolerance. In: Verma SK, White JF, editors. Seed endophytes. 1st ed. Cham: Springer Nature Switzerland; p. 273–311.
- Chanratana M, Han GH, Roy Choudhury A, Sundaram S, Halim MA, Krishnamoorthy R, Kang Y, Sa T. 2017. Assessment of Methylobacterium oryzae CBMB20 aggregates for salt tolerance and plant growth promoting characteristics for bio-inoculant development. AMB Express. 7(1):208. doi:10.1186/s13568-017-0518-7.
- Chappell PR. 2013. The climate and weather of Auckland. NIWA science and technology series 60. New Zealand: NIWA.
- Chebotar VK, Shcherbakov AV, Maslennikova SN, Zaplatkin AN, Kanarskiy AV, Zavalin AA. 2016. Endophytic bacteria of woody plants as the basis of complex microbial preparations for agriculture and forestry. Russian Agricultural Sciences. 42(5):339–342. doi:10.3103/S1068367416050037.
- Chesneau G, Laroche B, Préveaux A, Marais C, Briand M, Marolleau B, Simonin M, Barret M. 2022. Single seed microbiota: assembly and transmission from parent plant to seedling. mBio. 13(6):e01648–e01622. doi:10.1128/mbio.01648-22.
- Chock MK, Hoyt BK, Amend AS. 2021. Mycobiome transplant increases resistance to Austropuccinia psidii in an endangered Hawaiian plant. Phytobiomes Journal. 5(3):326–334. doi:10.1094/PBIOMES-09-20-0065-R.
- Compant S, Clément C, Sessitsch A. 2010. Plant growth-promoting bacteria in the rhizo- and endosphere of plants: their role, colonization, mechanisms involved and prospects for utilization. Soil Biology and Biochemistry. 42(5):669–678. doi:10.1016/j.soilbio.2009.11.024.
- del Barrio-Duque A, Ley J, Samad A, Antonielli L, Sessitsch A, Compant S. 2019. Beneficial endophytic bacteria-Serendipita indica interaction for crop enhancement and resistance to phytopathogens. Front Microbiol. 10:2888. doi:10.3389/fmicb.2019.02888.
- del Barrio-Duque A, Samad A, Nybroe O, Antonielli L, Sessitsch A, Compant S. 2020. Interaction between endophytic Proteobacteria strains and Serendipita indica enhances biocontrol activity against fungal pathogens. Plant and Soil. 451(1–2):277–305. doi:10.1007/s11104-020-04512-5.
- Dourado MN, Aparecida Camargo Neves A, Santos DS, Araújo WL. 2015. Biotechnological and agronomic potential of endophytic pink-pigmented methylotrophic Methylobacterium spp. BioMed Research International. 2015:909016.
- Dutta S, Choi SY, Lee YH. 2022. Temporal dynamics of endogenous bacterial composition in rice seeds during maturation and storage, and spatial dynamics of the bacteria during seedling growth. Front Microbiol. 13:877781. doi:10.3389/fmicb.2022.877781.
- Eevers N, Gielen M, Sanchez-Lopez A, Jaspers S, White JC, Vangronsveld J, Weyens N. 2015. Optimization of isolation and cultivation of bacterial endophytes through addition of plant extract to nutrient media. Microb Biotechnol. 8(4):707–715. doi:10.1111/1751-7915.12291.
- Egamberdieva D, Alimov J, Shurigin V, Alaylar B, Wirth S, Bellingrath-Kimura SD. 2021. Diversity and plant growth-promoting ability of endophytic, halotolerant bacteria associated with Tetragonia tetragonioides (Pall.) Kuntze. Plants (Basel). 11(1):1–13.
- Egamberdieva D, Wirth S, Alqarawi AA, Abd Allah EF. 2015. Salt tolerant Methylobacterium mesophilicum showed viable colonization abilities in the plant rhizosphere. Saudi J Biol Sci. 22(5):585–590. doi:10.1016/j.sjbs.2015.06.029.
- El-Sharkawy HHA, Rashad YM, Ibrahim SA. 2018. Biocontrol of stem rust disease of wheat using arbuscular mycorrhizal fungi and Trichoderma spp. Physiological and Molecular Plant Pathology. 103:84–91. doi:10.1016/j.pmpp.2018.05.002.
- Ferreira A, Quecine MC, Lacava PT, Oda S, Azevedo JL, Araujo WL. 2008. Diversity of endophytic bacteria from Eucalyptus species seeds and colonization of seedlings by Pantoea agglomerans. FEMS Microbiol Lett. 287(1):8–14. doi:10.1111/j.1574-6968.2008.01258.x.
- Furtado BU, Gołębiewski M, Skorupa M, Hulisz P, Hrynkiewicz K. 2019. Bacterial and fungal endophytic microbiomes of Salicornia europaea. Appl Environ Microbiol. 85(13). doi:10.1128/AEM.00305-19.
- Gardes M, Bruns TD. 1993. ITS primers with enhanced specificity for basidiomycetes - application to the identification of mycorrhizae and rusts. Molecular Ecology. 2(2):113–118. doi:10.1111/j.1365-294X.1993.tb00005.x.
- Genitsaris S, Stefanidou N, Leontidou K, Matsi T, Karamanoli K, Mellidou I. 2020. Bacterial communities in the rhizosphere and phyllosphere of halophytes and drought-tolerant plants in Mediterranean ecosystems. Microorganisms. 8(11). doi:10.3390/microorganisms8111708.
- Gerna D, Clara D, Allwardt D, Mitter B, Roach T. 2022. Tailored media are key to unlocking the diversity of endophytic bacteria in distinct compartments of germinating seeds. Microbiology Spectrum. 10(4):e0017222–e0017222. doi:10.1128/spectrum.00172-22.
- Gioia L, d’Errico G, Sinno M, Ranesi M, Woo SL, Vinale F. 2020. A survey of endophytic fungi associated with high-risk plants imported for ornamental purposes. Agriculture. 10(12). doi:10.3390/agriculture10120643.
- Hammer Ø, Harper DAT, Ryan PD. 2001. PAST: paleontological statistics software package for education and data analysis. Palaeontologia Electronica. 4:1–9.
- Hanin NA, Fitriasari PD. 2019. Identification of endophytic fungi from fruits and seeds of jambolana (Syzygium cumini L.) Skeels. IOP Conference Series Earth and Environmental Science. 276(1):12060. doi:10.1088/1755-1315/276/1/012060.
- Hardoim P. 2019. The ecology of seed microbiota. In: Verma SK, White JF, editors. Seed endophytes. 1st ed. Cham: Springer Nature Switzerland; p. 103–125.
- Hodgson S, de Cates C, Hodgson J, Morley NJ, Sutton BC, Gange AC. 2014. Vertical transmission of fungal endophytes is widespread in forbs. Ecol Evol. 4(8):1199–1208.
- Holland M, Davis R, Moffitt S, O'Laughlin K, Peach D, Sussan S, Wimbrow L, Tayman B. 2000. Using “leaf prints” to investigate a common bacterium. The American Biology Teacher. 62(2):128–131.
- Holland MA. 2019. Thinking about PPFM bacteria as a model of seed endophytes: Who are they? Where did they come from? What are they doing for the plant? What can they do for us? In: Verma SK, White JF, editors. Seed endophytes. 1st ed. Cham: Springer Nature Switzerland; p. 21–34.
- Indrawati IDA, Rossiana NIA, Fathurrohim MF. 2021. Diversity of endophytic bacteria and microfungi in Syzygium cumini fruit from West Java, Indonesia. Biodiversitas Journal of Biological Diversity. 22(9):3943–3948.
- Jeong S, Kim TM, Choi B, Kim Y, Kim E. 2021. Invasive Lactuca serriola seeds contain endophytic bacteria that contribute to drought tolerance. Sci Rep. 11(1):13307. doi:10.1038/s41598-021-92706-x.
- Jimu L, Kemler M, Wingfield MJ, Mwenje E, Roux J. 2016. The Eucalyptus stem canker pathogen Teratosphaeria zuluensis detected in seed samples. Forestry. 89(3):316–324. doi:10.1093/forestry/cpv037.
- Johnston PR. 1998. Leaf endophytes of manuka (Leptospermum scoparium). Mycological Research. 102(8):1009–1016. doi:10.1017/S0953756297005765.
- Johnston PR, Park D, Smissen RD. 2017. Comparing diversity of fungi from living leaves using culturing and high-throughput environmental sequencing. Mycologia. 109(4):643–654.
- Ko Ko TW, Stephenson SL, Bahkali AH, Hyde KD. 2011. From morphology to molecular biology: can we use sequence data to identify fungal endophytes? Fungal Diversity. 50(1):113–120. doi:10.1007/s13225-011-0130-0.
- Larrouy JL, Dhami MK, Jones EE, Ridgway HJ. 2023. Physiological stage drives fungal community dynamics and diversity in Leptospermum scoparium (manuka) flowers. Environ Microbiol. 25(3):766–771. doi:10.1111/1462-2920.16324.
- Lupo S, Tiscornia S, Bettucci L. 2001. Endophytic fungi from flowers, capsules and seeds of Eucalyptus globulus. Rev Iberoam Micol. 18(1):38–41.
- Martin PL, Peter KA. 2021. Quantification of Colletotrichum fioriniae in orchards and deciduous forests indicates it is primarily a leaf endophyte. Phytopathology. 111(2):333–344. doi:10.1094/PHYTO-05-20-0157-R.
- Martinez-Rodriguez A, Macedo-Raygoza G, Huerta-Robles AX, Reyes-Sepulveda I, Lozano-Lopez J, García-Ochoa EY, Fierro-Kong L, Medeiros MHG, Di Mascio P, White JF, et al. 2019. Agave seed endophytes: ecology and impacts on root architecture, nutrient acquisition, and cold stress tolerance. In: Verma SK, White JF, editors. Seed endophytes. 1st ed. Cham: Springer Nature Switzerland; p. 139–170.
- Matsumura E, Fukuda K. 2013. A comparison of fungal endophytic community diversity in tree leaves of rural and urban temperate forests of Kanto district, eastern Japan. Fungal Biol. 117(3):191–201. doi:10.1016/j.funbio.2013.01.007.
- Mengistu AA. 2020. Endophytes: colonization, behaviour, and their role in defense mechanism. Int J Microbiol. 2020:6927219. doi:10.1155/2020/6927219.
- Newcombe G, Marlin M, Barge E, Heitmann S, Ridout M, Busby PE. 2023. Plant seeds commonly host Bacillus spp., potential antagonists of phytopathogens. Microb Ecol. 85(4):1356–1366. doi:10.1007/s00248-022-02024-6.
- Niza-Costa M, Rodríguez-dos Santos AS, Rebelo-Romão I, Ferrer MV, Sequero López C, Vílchez JI. 2022. Geographically disperse, culturable seed-associated microbiota in forage plants of alfalfa (Medicago sativa L.) and pitch clover (Bituminaria bituminosa L.): characterization of beneficial inherited strains as plant stress-tolerance enhancers. Biology. 11(12):1838. doi:10.3390/biology11121838.
- Parte AC, Sarda Carbasse J, Meier-Kolthoff JP, Reimer LC, Goker M. 2020. List of prokaryotic names with standing in nomenclature (LPSN) moves to the DSMZ. Int J Syst Evol Microbiol. 70(11):5607–5612. doi:10.1099/ijsem.0.004332.
- Procópio RFL, Maccheroni W, Jr., Azevedo JL. 2009. Characterization of an endophytic bacterial community associated with Eucalyptus spp. Genetics and Molecular Research. 8(4):1408–1422. doi:10.4238/vol8-4gmr691.
- Puente ME, Li CY, Bashan Y. 2009. Endophytic bacteria in cacti seeds can improve the development of cactus seedlings. Environmental and Experimental Botany. 66(3):402–408. doi:10.1016/j.envexpbot.2009.04.007.
- Queiroz EG, Degenhardt J, Quoirin M, Silva K. 2020. Endophytic bacteria associated with tissue culture and leaves of Plinia peruviana. Pesquisa Agropecuária Brasileira. 55(1):1–10.
- Rezki S, Campion C, Simoneau P, Jacques M-A, Shade A, Barret M. 2017. Assembly of seed-associated microbial communities within and across successive plant generations. Plant and Soil. 422(1-2):67–79. doi:10.1007/s11104-017-3451-2.
- Ridout M, Newcombe G. 2018. Sydowia polyspora is both a foliar endophyte and a preemergent seed pathogen in Pinus ponderosa. Plant Dis. 102(3):640–644. doi:10.1094/PDIS-07-17-1074-RE.
- Robles CA, Lopez SE, McCargo PD, Carmarán CC. 2015. Relationships between fungal endophytes and wood-rot fungi in wood of Platanus acerifolia in urban environments. Canadian Journal of Forest Research. 45(7):929–936. doi:10.1139/cjfr-2014-0560.
- Rodriguez RJ, White JF, Jr., Arnold AE, Redman RS. 2009. Fungal endophytes: diversity and functional roles. New Phytol. 182(2):314–330. doi:10.1111/j.1469-8137.2009.02773.x.
- Roodi D, Millner JP, McGill C, Johnson RD, Jauregui R, Card SD. 2020. Methylobacterium, a major component of the culturable bacterial endophyte community of wild Brassica seed. PeerJ. 8:e9514. doi:10.7717/peerj.9514.
- Sahu PK, Tilgam J, Mishra S, Hamid S, Gupta A KJ, Verma SK, Kharwar RN. 2022. Surface sterilization for isolation of endophytes: ensuring what (not) to grow. J Basic Microbiol. 62(6):647–668. doi:10.1002/jobm.202100462.
- Saxena S, Strobel GA. 2021. Marvellous Muscodor spp.: update on their biology and applications. Microb Ecol. 82(1):5–20. doi:10.1007/s00248-020-01644-0.
- Sayers EW, Cavanaugh M, Clark K, Ostell J, Pruitt KD, Karsch-Mizrachi I. 2019. Genbank. Nucleic Acids Research. 48(D1):D84–D86.
- Schmidt-Adam G, Gould KS, Murray BG. 1999. Floral biology and breeding system of pohutukawa (Metrosideros excelsa, Myrtaceae). New Zealand Journal of Botany. 37(4):687–702. doi:10.1080/0028825X.1999.9512663.
- Schmidt-Adam G, Gould KS, Murray BG. 2002. Seed biology of Metrosideros excelsa (Myrtaceae). New Zealand Journal of Botany. 40(3):419–425. doi:10.1080/0028825X.2002.9512803.
- Schulz BJ, Rabsch L, Junker C. 2019. Chemical warfare in the plant microbiome leads to a balance of antagonisms and a healthy plant. Cham: Springer International Publishing. 171–189.
- Shahzad R, Khan AL, Bilal S, Asaf S, Lee IJ. 2018. What is there in seeds? Vertically transmitted endophytic resources for sustainable improvement in plant growth. Front Plant Sci. 9:24. doi:10.3389/fpls.2018.00024.
- Shetty KG, Minnis AM, Rossman AY, Jayachandran K. 2011. The Brazilian peppertree seed-borne pathogen, Neofusicoccum batangarum, a potential biocontrol agent. Biological Control. 56(1):91–97. doi:10.1016/j.biocontrol.2010.09.016.
- Shiomi HF, Silva HSA, Melo I, Nunes FV, Bettiol W. 2006. Bioprospecting endophytic bacteria for biological control of coffee leaf rust. Scientia Agricola. 63:32–39. doi:10.1590/S0103-90162006000100006.
- Simpson P. 2005. Pōhutukawa & rātā : New Zealand's iron-hearted trees. Wellington, N.Z: Te Papa Press.
- Smith GR, Ganley BJ, Chagne D, Nadarajan J, Pathirana RN, Ryan J, Arnst EA, Sutherland R, Soewarto J, Houliston G, et al. 2020. Resistance of New Zealand provenance Leptospermum scoparium, Kunzea robusta, Kunzea linearis, and Metrosideros excelsa to Austropuccinia psidii. Plant Dis. 104(6):1771–1780. doi:10.1094/PDIS-11-19-2302-RE.
- Strobel G. 2018. The emergence of endophytic microbes and their biological promise. J Fungi (Basel). 4(2).
- Sutherland R, Soewarto J, Beresford R, Ganley B. 2020. Monitoring Austropuccinia psidii (myrtle rust) on New Zealand Myrtaceae in native forest. New Zealand Journal of Ecology. 44:2. doi:10.20417/nzjecol.44.23.
- Toome-Heller M, Ho WWH, Ganley RJ, Elliott CEA, Quinn B, Pearson HG, Alexander BJR. 2020. Chasing myrtle rust in New Zealand: host range and distribution over the first year after invasion. Australasian Plant Pathology. 49(3):221–230. doi:10.1007/s13313-020-00694-9.
- Truyens S, Weyens N, Cuypers A, Vangronsveld J. 2015. Bacterial seed endophytes: genera, vertical transmission and interaction with plants. Environmental Microbiology Reports. 7(1):40–50. doi:10.1111/1758-2229.12181.
- Ulrich K, Stauber T, Ewald D. 2008. Paenibacillus — a predominant endophytic bacterium colonising tissue cultures of woody plants. Plant Cell, Tissue and Organ Culture. 93(3):347–351. doi:10.1007/s11240-008-9367-z.
- Verma SK, Kharwar RN, White JF. 2019. The role of seed-vectored endophytes in seedling development and establishment. Symbiosis. 78(2):107–113. doi:10.1007/s13199-019-00619-1.
- Vyshakhi AS, Anith KN. 2021. Co-inoculation with the root endophytic fungus Piriformospora indica and endophytic bacteria improves growth of solanaceous vegetable seedlings. International Journal of Vegetable Science. 27(6):536–551. doi:10.1080/19315260.2021.1885555.
- Weisburg WG, Barns SM, Pelletier DA, Lane DJ. 1991. 16S ribosomal DNA amplification for phylogenetic study. J Bacteriol. 173(2):697–703. doi:10.1128/jb.173.2.697-703.1991.
- White T, Bruns T, Lee S, Taylor J, Innis M, Gelfand D, Sninsky J. 1990. Amplification and direct sequencing of fungal ribosomal RNA genes for phylogenetics. In: Innis MA, Gelfand DH, Sninsky JJ, White TJ, editors. PCR protocols. San Diego: Academic Press; p. 315–322
- Whiting DC. 1986. Vegetation colonisation of Rangitoto Island: the role of crevice microclimate. MSc thesis (unpub.), University of Auckland. 107 p.
- Wicaksono WA, Jones EE, Monk J, Ridgway HJ. 2016. The bacterial signature of Leptospermum scoparium (manuka) reveals core and accessory communities with bioactive properties. PLoS One. 11(9):e0163717. doi:10.1371/journal.pone.0163717.
- Wicaksono WA, Jones EE, Sansom CE, Perry NB, Monk J, Black A, Ridgway HJ. 2017. Indigenous bacteria enhance growth and modify essential oil content in Leptospermum scoparium (mānuka). New Zealand Journal of Botany. 55(3):306–317. doi:10.1080/0028825X.2017.1330272.
- Wolfe ER, Kautz S, Singleton SL, Ballhorn DJ. 2018. Differences in foliar endophyte communities of red alder (Alnus rubra) exposed to varying air pollutant levels. Botany. 96(12):825–835. doi:10.1139/cjb-2018-0085.
- Zhao S, Zhou N, Zhao Z-Y, Zhang K, Wu G-H, Tian C-Y. 2016. Isolation of endophytic plant growth-promoting bacteria associated with the halophyte Salicornia europaea and evaluation of their promoting activity under salt stress. Current Microbiology. 73(4):574–581. doi:10.1007/s00284-016-1096-7.
- Zimmerman NB, Vitousek PM. 2012. Fungal endophyte communities reflect environmental structuring across a Hawaiian landscape. Proc Natl Acad Sci USA. 109(32):13022–13027. doi:10.1073/pnas.1209872109.