ABSTRACT
Reprocessing of mine wastes is a growing procedure around the world, in order to extract more resources from already-mined rocks. Mineralogical and geometallurgical investigations are useful for planned reprocessing, and in this study at Round Hill placer gold mine we characterise fine (<100 µm) and super-fine (micron-scale) particulate gold that has passed through the mine separation stages without being extracted. The gold was discharged with water and suspended sediments to settling ponds, separate from the more voluminous gravel and sand tailings. Authigenic clay and pyrite are abundant in the placer deposit and the settled suspension, and oxidised pyrite yielded gypsum and ferric oxyhydroxide. These minerals coat surfaces of gold particles and are locally intergrown with micron-scale authigenic gold. Most of the gold has toroidal shapes characteristic of beach derivation, and many of these are hollow or clay-filled. Minor super-fine cinnabar and mercury-bearing gold occur with clay. Annealing of gold has liberated silver, and micron-scale authigenic native silver occurs in the settled sediment. Reconcentration of fine and super-fine gold used abundant clean water and slow reprocessing and may be currently uneconomic at this site. The observations on suspended gold loss are relevant to gold recovery at placer mines around the world.
Introduction
Mine tailings are a major component of the global waste stream, and there is growing economic and environmental interest in reprocessing tailings for valuable mineral residues (Hudson-Edwards et al. Citation2011; Edraki et al. Citation2014; Kinnunen et al. Citation2022). Gravity-driven separation of dense (‘heavy’) minerals is an important aspect of both initial processing and reprocessing of some tailings (Guney et al. Citation2001; Edraki et al. Citation2014; Wang et al. Citation2014; Leistner et al. Citation2016; Kinnunen et al. Citation2022). Efficiency of this gravity separation is affected by the density contrasts and the particle sizes and shapes of the target minerals (Guney et al. Citation2001; Donoso et al. Citation2013; Wang et al. Citation2014; Leistner et al. Citation2016; Kinnunen et al. Citation2022). In this paper, we address these potential reprocessing issues for an active placer gold mine in New Zealand by defining the nature of remnant heavy minerals, especially gold, in mine wastes with extremely fine particle sizes.
Finely particulate placer gold (<100 µm) is notoriously difficult to concentrate and extract by casual panning or in commercial mining situations that rely on the high density of gold to settle the particles (Kungurova Citation2021; Palmer and Craw Citation2023). Extraction is hindered or made inefficient because fine gold can float on water and/or can be carried off with other, less-dense, heavy minerals during concentration processes (Craw et al. Citation2013; Citation2015; Shuster et al. Citation2016; Ritchie et al. Citation2019; Craw and Kerr Citation2021; Kungurova Citation2021). Clay-rich processing water further inhibits gold concentration processes, and this issue is common in paleoplacer deposits where groundwater alteration has caused decomposition of labile minerals in the hosting sediments (Druzbicka and Craw Citation2012; Craw et al. Citation2013, Citation2015; Malloch et al. Citation2017; Craw and Kerr Citation2021). Hence, most placer mining operations tend to extract the coarsest and medium sized particles (commonly 100 µm to 5 mm) most efficiently and extraction of finer gold is haphazard and inefficient at best (Kungurova Citation2021; Palmer and Craw Citation2023).
The problem of fine gold extraction is particularly acute when mining beach placers, where a high proportion of the gold is finely particulate (Boyle Citation1979; Ritchie et al. Citation2019; Kungurova Citation2021; Craw and Kerr Citation2021; Palmer and Craw Citation2023). This issue is a well-known phenomenon on beaches of the west and south coasts of the South Island of New Zealand where there has been a long history of mining since the mid-nineteenth Century (Williams Citation1974; Fraser Citation2011; Craw et al. Citation2013; Ritchie et al. Citation2019; Palmer and Craw Citation2023). At the Round Hill mine site on the south coast that is the topic of this study, the fine gold even resists chemical amalgamation with mercury that is added to heavy mineral concentrates to enhance the gold extraction process (Palmer and Craw Citation2023).
In this study we present mineralogical descriptions of fine and super-fine gold and associated metallic minerals that were recovered from the clay-rich sludge discharged from an active placer gold mine. A principal aim of this study is to define the nature, particle size, and associated minerals of the gold so that (a) redesign of fine gold processing streams can be considered; and (b) future recovery technology can focus on particles of this type in mine wastes. The sludge was obtained from a settling pond that receives mine process waters, implying that the fine gold bypassed the concentration processes in suspension in the water. Gold in this unique sample has a wide range of sizes and shapes, from flakes that are almost 200 µm across down to submicron irregular particles and coatings on other minerals. We describe these metallic materials from the perspective of their geological and geochemical origins in the deposit and in the region, with particular focus on the super-fine component of this particle size spectrum (arbitrarily defined here as <30 µm). We also use a geometallurgical approach to evaluate the potential for future mine extraction based on the mineralogy and morphology of this gold that escaped from the processing plant in suspension.
General setting
The Round Hill mining area that is the site for this study is 3 km north of the Foveaux Strait coastline in the southern South Island (a,b). The mining area was developed in Pleistocene marginal marine sediments that have been raised by on-going slow tectonic uplift (Turnbull and Allibone Citation2003; Craw and Kerr Citation2021). The gold was ultimately derived from the Otago Schist, ∼200 km to the north (a), and was transported southwards during the Cenozoic, with various stages of recycling and particle modification during river drainage reorientation (Craw et al. Citation2013, Citation2015; Upton and Craw Citation2016). Fine gold accumulated on beaches as sea levels changed through the Pleistocene, and these uplifted beaches then shed gold into short stream channels that led back to the coast (Turnbull and Allibone Citation2003; Craw et al. Citation2013, Citation2015; Craw and Kerr Citation2021). Historic and modern mining was focused on these channels in which fine beach gold was locally concentrated in basal gravels (b–e); Turnbull and Allibone Citation2003; Craw and Kerr Citation2021).
Figure 1. Location and stratigraphic setting for the sediments extracted for this study. (a) Location of Round Hill mining area in southern South Island. (b) Geological setting of the Round Hill mining area. (c) Outcrop of the basal Pleistocene gravel and immediately overlying sand that is processed for gold extraction at the modern mine. (d) Oxidised, weakly acidic groundwater runoff from the Pleistocene sediments. (e) Close view of a water discharge zone in c. (f) Outcrop view of partially oxidised authigenic pyrite in Pleistocene sand, with iron oxyhydroxide (HFO), gypsum and jarosite oxidation products.
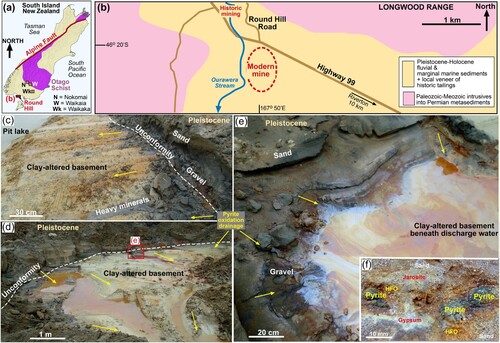
The Pleistocene sediments rest unconformably on Paleozoic-Mesozoic basement that is dominated by granitoid and mafic intrusive rocks (b); Turnbull and Allibone Citation2003; Ashley et al. Citation2012). In particular, the Longwood Range (b) is dominated by gabbroic intrusives (Turnbull and Allibone Citation2003; Ashley et al. Citation2012) that contributed locally-derived sediments to the Pleistocene gravels and sands. Groundwater-driven alteration of the labile minerals in the basement rocks and overlying sediments has caused widespread clay formation, especially near to the unconformity (c–e; Craw and Kerr Citation2021). This clay alteration was also accompanied by calcite and abundant authigenic pyrite cementation in permeable sites in the basement and the sedimentary sequence (f; Craw et al. Citation2015; Craw and Kerr Citation2021). Oxidation of the pyrite has occurred where the sediments were raised above the low redox conditions of the coastal plain, creating widespread formation of iron oxyhydroxide (HFO; hydrous ferric oxide) cements and weak acidic groundwater (pH 5-7) at the local scale (centimetres to metres; f), although the authigenic calcite neutralises this at a larger scale (Craw and Kerr Citation2021). This oxidation process is locally enhanced by mining excavations (c–f). As a consequence, gypsum, jarosite and HFO have formed in the mine excavations, and also near the water table prior to mining (c–f; Craw and Kerr Citation2021).
Methods
Sample extraction
The principal sample for this study was obtained by the modern mine operators who were investigating losses of gold in their processing system (a–e). The processing plant extracts gold through several stages of detrital particle size separation accompanied by gravity settling and high-speed centrifugal spinning (a). Water washing occurs at all stages, leading to abundant processing waters containing suspended sediments rich in clays as the agitated clay-rich feed materials (c–f) are decomposed and disaggregated. These processing waters are discharged to a chain of settling ponds (c,d) so that the suspended components can settle out (e) and the resultant clean water can be either re-used or discharged into the environment.
Figure 2. Modern Round Hill mine processing site, and source of the material examined in this study. (a) General view of the processing plant. (b) Portion of gold extraction process with Gemeni table. (c) Settling pond that receives the first discharge water from the processing plant. (d) An inactive settling pond that had been dewatered prior to extraction of the sediment. (e) Wet sediment from the first settling pond (as in c), showing general particle size variations and bedded structure (white dashed lines). (f,g) Stereomicroscopic views of dried settling pond sediment (fine sand layers) with rare gold flakes (centre) oriented on bedding surfaces.
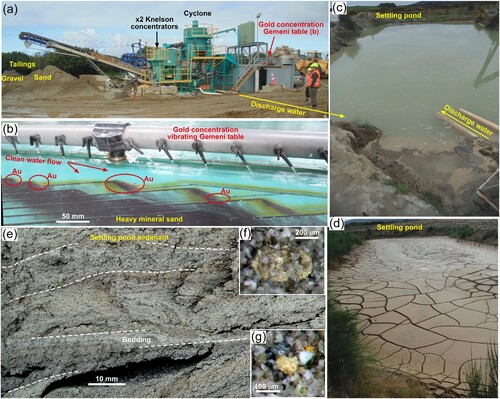
The gold-rich material for this study was obtained from the fist settling pond. No gold has yet been found in subsequent ponds. A bulk sample of sludge was dug from the floor of the first settling pond (c) with a two cubic metre excavator bucket. From that bulk sample, a 20 L bucket full of sludge was slowly reworked through the latter stages of the gold-saving system in the plant on the Gemeni table (a,b) to remove the dominant clay-rich sediment and leave a heavy mineral concentrate containing the gold and detrital particles with attached gold (a–f). This process was done without the routine mine processing activities in operation, so that only the sludge was treated and only sludge-derived gold was saved.
Figure 3. Light microscope views of gold extracted from settling pond sediment. (a) General view showing typical particle sizes and abundance of toroidal shapes. (b) Close view showing rarer flakes and rough irregular fine particles. (c-e) Close view of typical particles. (f) Close view of a quartz sand particle with adhering fine gold particles.
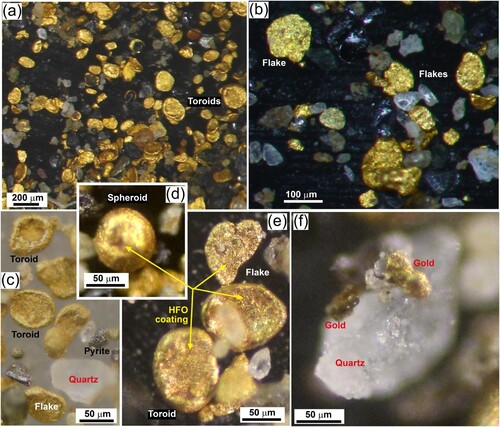
A second sludge sample (e) was obtained in a similar manner from the first settling pond in order to examine the sludge material itself in more detail. This sludge material is essentially identical to the material that was reprocessed at the mine to obtain the gold concentrate. A portion of this second sample was essentially intact, with bedding preserved. A two kg portion of this bedded sludge was dried on a laboratory bench for two months and became firm enough to place as blocks beneath a stereoscopic light microscope for examination of gold particles in situ (f,g).
Scanning electron microscopy
Sample particles were mounted onto aluminium stubs using double-sided carbon tape by pressing the stubs down on to the sample in a watch glass. Observations of particle morphology and composition were made using a scanning electron microscope (SEM) with energy-dispersion analytical attachment (EDX). A Zeiss Sigma variable-pressure SEM instrument at the Otago Micro and Nanoscale Imaging (OMNI; University of Otago, New Zealand) was used to obtain backscatter-electron images (BEI) with an accelerating voltage of 15 kV and an aperture of 120 μm. Low pressure nitrogen gas was introduced to the instrument vacuum to limit electron-charging of non-conducting materials without a carbon coat. This approach enhanced resolution of fine-scale features in images at the micron scale.
Analysis by EDX of rough surfaces on fine grained materials is semiquantitative at best, and our aim was to define principal elements and approximate relative proportions. The electron beam is ∼3 µm diameter, so many analyses of fine materials are composites. A particular issue for this study was the overlap of Au and Hg peaks in the energy spectrum. The presence of several weight percent Hg in gold can be detected by asymmetric broadening of the combined Mα1 peak that is the principal element identifier. Likewise the secondary Lα1.
Au peak was broadened asymmetrically. A further complication was partial overlap of Hg Mα1 with the S Kα1 peak, but these were sufficiently resolved to identify cinnabar (HgS) provided little or no Au was present in the composite analysis. Gypsum was also readily identifiable in conjunction with Au as the S Kα1 peak was resolved and separate Ca and O peaks were visible. Silver was readily identified as a separate phase and as a minor component (>1 wt%) in gold particles. The presence of C and O in carbonate minerals was partially obscured by the underlying carbon mounting tape, and presence of oxygen in all silicates and in iron oxyhydroxide (HFO) stains and cements.
Results
Detrital gold
Gold particles were observed in various parts of the 2 kg dried sludge sample, and two of these were on a single bedding plane surface (f,g). Rare coarse flakes (up to 300 µm; f) occur in situ on the bedding surfaces, whereas most gold observed in situ in the dried sludge was at least 100 µm across (e.g. g). The in situ gold was almost all flaky in shape and oriented sub-parallel to the bedding surfaces, although one small (∼90 µm) rounded toroid was observed as well. The observed in situ gold was in fine sand that was similar in particle size or finer than the gold particles (f,g).
Most of the detrital gold in the reprocessed sludge sample has medium to fine particle sizes (50-150 µm; a–f; a–c; a–c,f) compared to the coarsest gold produced at the site. This medium sized particle population in the processed sludge sample is dominated by toroidal gold, with thickened rims and thinned centres (a,c,e; a,c). Dumbell shapes are similar to toroids but are elongate with thickened ends and thinned central portions (a,b). All these particles have smooth surfaces, although in detail there are numerous small embayments (b,c). Smooth spheroidal particles are also abundant (d; a–c,f). In addition, there are numerous flakes, mostly with rough surfaces but with rounded rims, and many flakes have smooth thickened rims to give a dish shape that is a precursor to toroidal shapes (a–c,e; a,b).
Figure 4. SEM electron backscatter images of gold extracted from the settling pond sediment. Clay appears as dark grey or black areas in most images. (a) General view showing ranges of coarser particle sizes and shapes. (b) Close view of some medium and fine particles, as in a. (c) A typical small toroid with internal clay and micron-scale authigenic gold in that clay. (d) Irregular and elongated fine gold particle with adhering clay. (e) Ragged fine gold particle with adhering clay. (f) Clean smooth fine Ag-bearing gold particle.
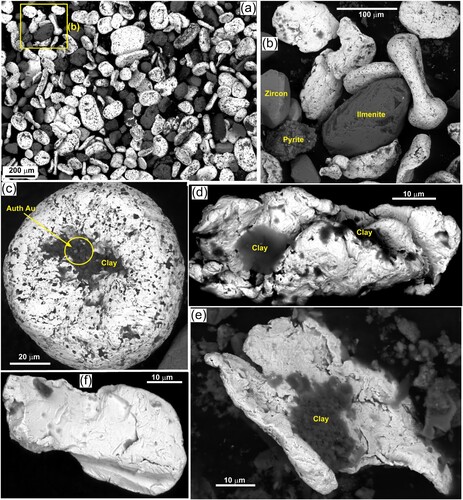
Figure 5. Gold with exterior coatings. (a) Spheroid with large attachment of clay and minor authigenic pyrite. Minor micron-scale authigenic gold impregnates clay. (b,c) Iron oxyhydroxide (HFO) coatings on spheroidal gold particles. (d) Rough margin of a detrital gold particle pokes through a thick clay coating. (e) Close view of portion of d. (f) Spheroidal particle with internal and external clay, with localised gypsum coating. (g) Close view of gypsum-rich coating in f. (h) Small smooth detrital gold particle adhering to detrital zircon, with gypsum and HFO partial coating.
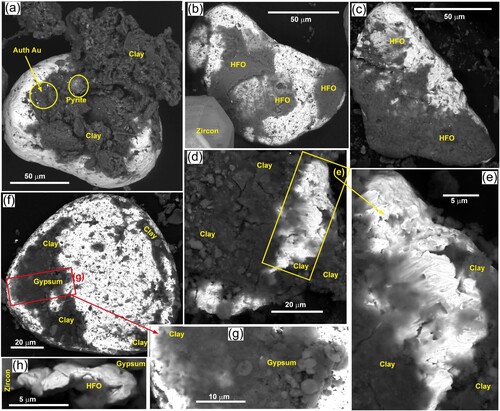
In contrast, finer particles (∼50 µm and smaller) are generally more irregular in shape, although most are broadly equant rather than flaky (a–f; a,b,d–f). These smaller particles commonly have rough and irregular surfaces and edges (b,d,e; d,e). However, rare small smooth particles also occur (f). Despite the generally irregular shapes of many of these smaller particles, most edges have some evidence for deformation and rounding, with locally thickened or folded margins (b,d–f; d,e,h).
Most of the medium and fine particles (<300 µm) have partial coatings of clay, which is preserved in embayments in the gold surfaces (a–e). Some of the clay fills internal cavities in toroids and spheroids and is only visible externally where those cavities are coinnected to the surface (c,d; f). In contrast, large accumulations of clay locally dominate and overwhelm gold particles (a,d,e). Clay coatings have highly variable compositions but are dominated by Al–Fe-Mg–Ca smectites. Minor detrital muscovite or its illitised equivalent accompanies the smectites. Some gold particles are partially coated with iron oxyhydroxide (HFO) as analysed via SEM (b,c,h). Minor HFO coatings are also abundantly observed as pale red patches on exterior surfaces in light observations (a,c–e).
Gypsum is a common authigenic precipitate mineral associated with oxidised pyrite and HFO in mine pits, where it coats outcrops, detrital particles and partially oxidised authigenic pyrite (d). Gypsum locally coats some gold surfaces (f–h), typically in conjunction with clay coatings.
Super-fine authigenic gold
Some of the medium-fine detrital gold particles with clay coatings have micron-scale gold particles dispersed through the clay (c; a) and this is presumed to be of authigenic rather than detrital origin based on previously published observations (Craw et al. Citation2015; Craw and Kerr Citation2021). Some of the highly irregular margins on fine detrital gold, and delicate micron-scale gold protrusions in surface embayments may also have similar origins (d,e; d–h). Authigenic pyrite forms framboidal spheres (a) and irregular crystalline coatings on some detrital minerals (b,c) and these common features have locally provided substrates for deposition of authigenic gold (b,c,e,f). However, oxidised pyrite framboids that now consist of HFO, and HFO coatings of detrital particles, apparently have not attract authigenic gold deposition (b,c; d).
Figure 6. SEM electron backscatter images of some iron minerals relevant to accumulation of super-fine authigenic gold, extracted from the settling pond sediment. (a) Authigenic pyrite framboid. (b) Authigenic pyrite coating a fracture surface on detrital uraninite particle. (c) Close view of pyrite in b, with authigenic gold. (d) Fully oxidised pyrite framboid. (e) Partially oxidised and clay-coated authigenic pyrite framboid, with super-fine authigenic gold. (f) Close view of authigenic gold in e. (g) Detrital magnetite particle with localised coatings of authigenic gold. (h) Close view of largest coat of authigenic gold in g.
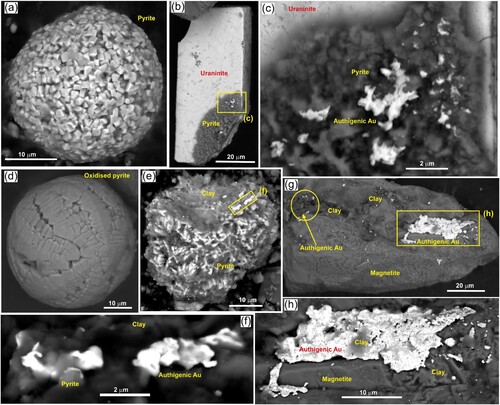
Detrital magnetite can also attract authigenic gold, as numerous irregularly shaped micron-scale particles and as larger encrustations (g,h). The example in g also has micron-scale authigenic gold intergrown with some of the clay coating. Three particles of what appears to be detrital native iron also have micron-scale spots of authigenic gold and larger marginal coatings (a–d), similar to the magnetite in g,h. The apparent native iron particles beneath this authigenic gold lacks oxygen in EDX analyses, and has low but detectable Al and Cu contents (a,b). Potential origins of this anomalous host for authigenic gold are outlined in a Discussion section below.
Figure 7. EDX spectra and backscatter electron images for super-fine authigenic gold in association with contrasting redox minerals. (a,b) Representative EDX spectra for authigenic gold on native iron, at points indicated in c and d. Red arrow indicates the position for oxygen peak which, if present, is small and overlaps with other peaks. (c,d) Authigenic gold coating fragments of detrital native iron that contains traces of Al and Cu (as in a,b). Black background is carbon mounting tape that contributes a distinct carbon peak in both spectra in a and b. (e,f,g) Authigenic gold intergrown with authigenic gypsum.
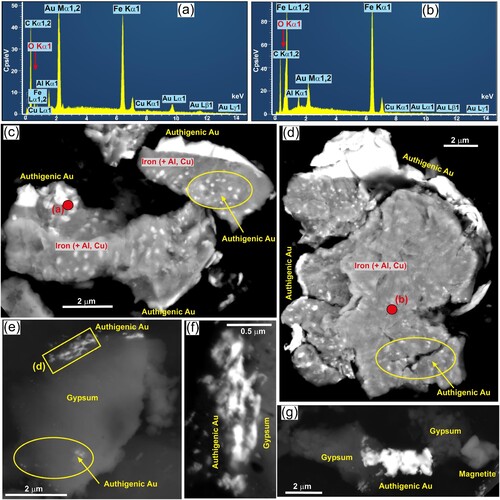
Gypsum occurs at the other, highly oxidised, end of the redox spectrum (g) from the relatively low redox magnetite, pyrite and native iron, and some authigenic gypsum is intergrown with authigenic gold as well (e–g). The authigenic gold that has become intergrown with gypsum is highly irregular in shape and forms super-fine particles, some of which are sub-micron in size (e–g).
Mercury-bearing gold
Gold with detectable Hg occurs in the sludge sample with a wide range of particle sizes <50 µm (a–f). Rounded crystalline particles, which are presumed to be of detrital origin (a,b) are equant in shape and 30-50 µm in diameter, and therefore quite distinct from the typical detrital gold particles described above (a; a). In contrast, Hg-bearing gold coatings and impregnations are typically micron scale and have highly irregular shapes (c–e) and these are presumed to be of authigenic origin like the Hg-free particles with similar textures described above (e.g. c; a). Coatings of Hg-bearing gold on a super-fine particle of corroded cinnabar have two distinct textures: delicate vermiform micron-scale patches, and smooth-surfaced thick coverings (c). Micron-scale Hg-bearing gold pervades some agglomerated clay masses (d,e), and some similar super-fine particles are intergrown with clay on the surface of a detrital Hg-bearing gold particle (b). Some larger (10-50 µm) particles of Hg-bearing gold are irregular in shape and are closely related to clay masses (d,f). It is not clear from these textures whether these particles are detrital or authigenic in origin.
Figure 8. SEM electron backscatter images of super-fine mercury-bearing gold from the settling pond sediment. (a, b) Detrital Hg-bearing gold with rounded remnants of crystalline shapes and partial clay coatings. (c) Detrital cinnabar particle with Hg-bearing gold partially coating the surface. (d) Super-fine Hg-bearing gold dispersed through clay. (e) Super-fine Hg-bearing gold abundantly dispersed through an agglomerate of clay, surrounded by clay with scattered Hg-bearing gold. (f) Hg-bearing gold intimately interleaved with clay.
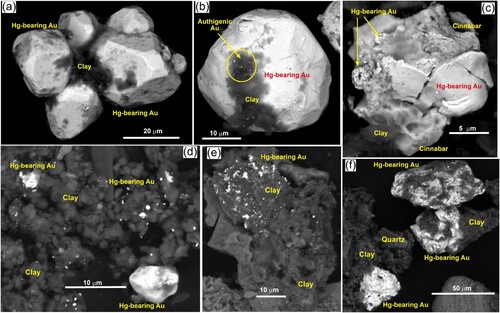
Silver
Some of the cores of toroidal gold particles retain some of their original Ag contents (typically <10 wt%) in their interior structure but most of the exterior portions of the toroids have had their Ag leached (Ag <1 wt%; Palmer and Craw Citation2023; Palmer et al. Citation2024). The detrital gold particles from the sludge sample in this study likewise have <1 wt% Ag. However, some native Ag occurs within the sludge sample, as separate particles and as intergrowths with clay (a–d). The silver particles are all super-fine (<20 µm) and highly irregular in shapes (a–d). Some of the silver in the particles has micron-scale vermiform textures (a,b). This vermiform silver is intergrown with, or coated by, smoother plates of silver, and the silver intergrown with clay is similarly plate-like rather than vermiform (c,d).
Discussion
Natural versus anthropogenic materials
Mercury is used in the latter stages of gold concentration in the modern mining process (Palmer and Craw Citation2023), but this is done in a laboratory setting distant from the mine site. Nevertheless, it is possible that some mercury contamination has remained in various receptacles used to transfer concentrates. Further, historic mine tailings, locally several metres thick, cover parts of the modern mining area, and these tailings may contain anthropogenic mercury compounds as well. However, anthropogenic mercury contamination is typically distinctive, occurring as liquid Hg droplets and particle coatings and as white Hg-Au amalgam stains on partially amalgamated gold (a; Malloch et al. Citation2017; Palmer and Craw Citation2023). Alternatively, highly irregular shaped grey Au-Hg amalgam particles and/or highly irregular shaped Au that has had Hg extracted from amalgam in the latter stages of gold cleaning may also occur (Youngson et al. Citation2002). However, none of these features are apparent in the sludge sample examined in this study. On the other hand, there appear to be detrital Hg-bearing materials in the sample, including crystalline but rounded Hg-bearing gold particles (a,b) and detrital cinnabar (c). The cinnabar depicted in c has a scalloped and corroded surface which may indicate localised dissolution of the particle and associated liberation of Hg. Hence, we conclude that at least some, and probably all, the Hg-bearing materials in the sludge sample are of natural origin, either detrital or authigenic.
Figure 10. Anthropogenic artefacts in heavy mineral concentrates extracted from Southland fluvial gold paleoplacer deposits. (a) Gold toroid (Au) from Round Hill mine that passed through Hg amalgamation off the site, and has minor Hg coating on rim (AuHg). Residual Hg droplets on other particles are in background. (b) Magnetic concentrate from Waikaka (a), showing abundant anthropogenic Cr-bearing steel fragments. The predominant spherical fragments were derived from welding (Weld) and related construction activities. Angular shards were torn from steel machinery that was used in extraction of sediments. Some minor natural detrital magnetite and chromite particles are circled. (c) SEM sample mount of particles from Waikaia (a). Two shards of anthropogenic Cr-bearing steel (top) are partly coated in brown Fe oxyhydroxide that was disrupted during mounting. Detrital cinnabar (red, bottom) also has fine particles that were shed during the mounting procedure.
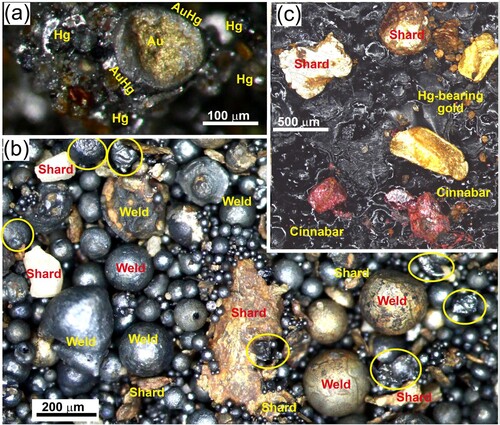
The apparent native iron particles (a–d) are geologically anomalous, as native iron is extremely rare in the natural environment (Ulff-Moller Citation1990; Ryabov and Lapkovsky Citation2010; Pernet-Fisher et al. Citation2017). Meteoritic iron typically contains abundant Ni (Pernet-Fisher et al. Citation2017), which is absent from the sludge particles. Anthropogenic steel fragments are common in placer gold mining concentrates, and we present two nearby examples (b,c) to emphasise the differences between these anthropogenic materials and the native Fe in the Round Hill sludge. Anthropogenic steel typically occurs as highly angular sharp shards torn from mining equipment, or as spherical residues from production of the steel item or subsequent welding (a,b). All these fragments contain detectable Cr (0.5–4 wt%) but no detectable Al or Cu. Further, these anthropogenic steel fragments commonly have at least some surficial brown or bronze coloured Fe oxyhydroxide film (a,b), but no oxidation film was detected on the particles from the Round Hill sludge (a–d).
From these above observations, we discount extraterrestrial or anthropogenic sources for the apparent native iron particles in c,d. The scattered authigenic gold that coats these particles also attests to long-term groundwater mobilisation and redeposition of gold, rather than this occurring on human time-scales. Consequently, we speculate that the native iron was originally formed during intrusion of mafic magmas into low-redox metasediments of the Longwood Range (b; Ashley et al. Citation2012), as has been described elsewhere in the world (Ulff-Moller Citation1990; Ryabov and Lapkovsky Citation2010; Pernet-Fisher et al. Citation2017). Subsequent erosion has presumably transported clasts of this native iron into the Pleistocene sediments where they must have remained well below the water table to resist oxidation. The presence of a particle of uraninite (b), also coated with authigenic pyrite, both of which are unstable in oxygenated environments (Boonchayaanant et al. Citation2009; Wang et al. Citation2013) supports that latter conclusion on redox setting.
Sources, compositions, and transport of detrital gold
The Otago Schist belt (a) is the general source for the detrital gold in Southland in general and also the Round Hill area (Williams Citation1974; Craw et al. Citation2013, Citation2015; Upton and Craw Citation2016; Palmer and Craw Citation2024). Presumably gold in the sludge sample was derived from this ultimate source. Deformation, flattening and associated internal recrystallization of the gold particles during and after transport has resulted in loss of original Ag from most of the gold (Stewart et al. Citation2017; Craw et al. Citation2017; Palmer and Craw Citation2023, Citation2024; Palmer et al. Citation2024). Further Ag leaching has occurred during formation of toroids by sand-blasting on the beaches of the south coast, and subsequent recycling into the paleodrainage system that is currently being mined (Craw and Kerr Citation2021; Palmer and Craw Citation2023; Palmer et al. Citation2024). Some of that leached Ag has apparently precipitated in situ or nearby (). Consequently, there are few original compositional features preserved from the gold sources.
The Hg-bearing gold in the sludge sample is one important exception to the above observation, as the Hg content is distinctive. The most abundant Hg-bearing gold in the potential source areas occurs on the southern margin of the Otago Schist belt in the Nokomai area (a; Youngson et al. Citation2002). This potential source area is directly upstream of the Round Hill area in the late Cenozoic paleodrainage system (Upton and Craw Citation2016). In that area, Hg-bearing gold contains up to 10 wt% Hg, and some of that gold has crystalline morphology (Youngson et al. Citation2002). Hence, this area is the most likely source for the distinctive Hg-bearing crystalline particles in the sludge (a,b). Some secondary Hg-bearing gold in the Nokomai area contains up to 37 wt% Hg (Youngson et al. Citation2002), but the textures are different (porous overgrowths) from those in the sludge sample. The Nokomai area is also a source of detrital cinnabar, and some of that cinnabar has been altered to contribute Hg to the secondary Hg-bearing gold, and also to produce small amounts of natural liquid Hg (Youngson et al. Citation2002).
The cinnabar particles in the sludge sample (e.g. c) may have been derived from this Nokomai source, or from nearby Waikaia area (a) where cinnabar also occurs with Hg-bearing gold (c; McLachlan et al. Citation2018). Cinnabar also occurs in the vicinity of the Waikaka area (a; Williams Citation1974), so detrital cinnabar and associated Hg-bearing gold are common features of the Southland placer system. Detrital cinnabar readily fractures and breaks down physically to fine particles in the sedimentary system (Garnett and Bassett Citation2005), as indicated by fine debris created during minor anthropogenic handling of particles (c). This may explain the super-fine particle size of cinnabar in the Round Hill sludge (c) compared to upstream fluvial particles (c). Addition of Hg-bearing gold to the surface of cinnabar is known to have occurred in the Nokomai source area, for example (Youngson et al. Citation2002), so the particle in c may be wholly detrital.
Super-fine authigenic gold
Gold is readily chemically mobilised at low temperatures and neutral-alkaline pH as sulphur complexes (a; Webster and Mann Citation1984; Webster Citation1986). Under low-redox conditions, Au forms the bisulphide complex (Au[HS]2-) from low-redox dissolved sulphur species (a; Webster and Mann Citation1984; Webster Citation1986). Gold is not soluble in oxidised waters where sulphate ions generally prevail (a). However, Au can be mobilised as thiosuphate complexes, as metastable thiosulphate ions (S2O32−) are formed ephemerally as intermediate species during oxidation of sulphide minerals, especially pyrite (a; Webster and Mann Citation1984; Webster Citation1986). Hence, there is a field of Au solubility in pH-redox space (red dashed area; a) in which dissolution can occur, with redeposition occurring wherever appropriate conditions change (a; Webster and Mann Citation1984; Webster Citation1986).
Figure 11. Summary diagram depicting chemical and physical processes that lead to fine and superfine gold in suspension in discharge waters. (a) Eh-pH diagram (calculated with Geochemists Workbench) showing the principal chemical processes affecting sediment mineralogy and associated metal mobility (see text for further explanation). (b) Geological setting of clay alteration at the site of placer gold accumulation in the basal gravels. (c) Low-redox mineralogy (lower panel) and high-redox mineralogy (upper panel) in the basal gravels. (d) Principal features of gold, following on from a, b and c, that result in suspension in discharge waters.
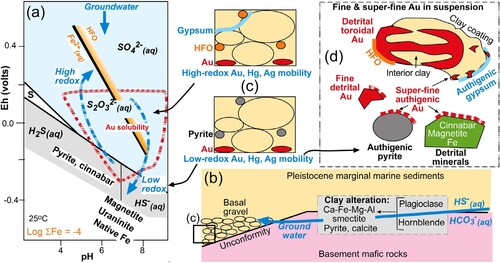
The authigenic gold textures and substrates described in this study suggest that there has been a combination of low-redox and high-redox processes involved in mobilising and reprecipitating gold (b–d). Authigenic pyrite has been a substrate for redeposition of Au under low-redox conditions, with additional low-redox maintenance by cinnabar, magnetite, uraninite and native iron (a,b,d). In addition, high-redox conditions that led to formation of gypsum from oxidation of pyrite were also favourable for Au mobilisation and redeposition, presumably as thiosulphate complexes (a,c,d). These processes were accompanied by localised mobilisation and redeposition of Hg and Ag (c; Palmer et al. Citation2024), although the details of the chemistry of these processes are beyond the scope of this study.
Geometallurgical issues
Our observations on the fine and superfine gold in this sludge sample provide some context for understanding the reasons why there is significant gold carried away from the processing plant in suspension in the discharge waters. Experimental work by Gul et al. (Citation2012) has shown that two serially deployed Knelson concentrators, as used in the Round Hill mine (a), are effective at settling gold and gold-bearing clasts at the 100 µm particle size level. The fine and super-fine Round Hill particles, as well as some larger particles, are clearly escaping this processing stage although the exact mechanisms that hinder gold settling are not clear. Fine gold is notorious for floating on water because its surface properties make it hydrophobic (Allan and Woodcock Citation2001) and this is presumably one of the factors that limits gravity settling (Palmer and Craw Citation2023). The hydrophobicity is exacerbated by mechanical deformation of the surficial grains that make up the particles (Allan and Woodcock Citation2001), and this type of deformation is a major component of the evolution of the Round Hill gold, especially the toroids and spheroids (Palmer and Craw Citation2023, Citation2024; Palmer et al. Citation2024).
The intense clay alteration of the hosting sediments and underlying basement (c–f; b) may be a major contributor to gold suspension, and this has a double effect: clay-rich processing water, and clay adhering to particles. The presence of the clay in the processed sediments, and inevitable incorporation of some clay-altered basement material in the ore-feed, causes processing water to develop a higher density with the abundant suspended clay particles, and this inhibits density-driven extraction of the gold (Malloch et al. Citation2017; Palmer and Craw Citation2023). Mechanical entrainment of gold with the suspended clay further facilitates this effect (Liu et al. Citation2018).
A clay film does not form on the surface of the gold while it is in a clay-rich suspension such as a processing slurry (Liu et al. Citation2018). However, gold particles can have a negative electrostatic surficial charge at neutral-alkaline pH (Monte et al. Citation2000), such as that which prevails in the Round Hill processing system. Hence, it is possible that fine gold may be electrostatically attracted to positively charged surfaces of clay or other suspended minerals. Further, formation of the authigenic clay around the gold particles in situ in the paleoplacer deposit means that many particles retain an interior infilling and exterior coatings of clay (c,d; a–e; d). This issue may be more widespread than the general view in a suggests, as this sample was slowly and carefully washed with clean water on the Gemeni table (b) so that external and internal clay was almost certainly removed in this process. Clay-related mechanisms may therefore be important factors in retaining gold in suspension through to the discharge water at Round Hill mine.
Conclusions
Some gold passes through an active beach placer mine processing system while suspended in clay-rich water and this gold ends up in settling ponds. The resultant settled slurry accumulates in the settling ponds as mine waste deposits separate from the more voluminous gravel and sand tailings. Some of the gold in settling pond deposits is present as fine particles (<100 µm) that are mostly toroidal in shape, and many toroids have hollow interiors that contain clay, air or water. A minor component of the gold is coarser (up to 300 µm) and has flaky morphology. In addition, some super-fine (micron scale) gold adheres to other sedimentary particles in the suspended slurry that passes through the processing system to the settling pond. This super-fine fraction includes authigenic gold with complex and delicate shapes. Particles of super-fine (<20 µm) detrital Hg-bearing gold occur as well, and some of this gold is intimately intergrown with clay.
Partial clay or Fe-oxyhydroxide coatings are common on many gold particles. The unmined deposit is rich in clay from groundwater alteration of labile minerals in the underlying igneous basement and gravel clasts derived from that basement. Authigenic pyrite is abundant within the sediments, and this has been partially oxidised in situ to Fe-oxyhydroxide and gypsum. Deformation of gold particles in the sedimentary system prior to deposition, followed by crystallographic annealing, has caused partial leaching of Ag from the gold, with localised minor deposition of authigenic native Ag. These authigenic minerals accompany the fine and super-fine gold into the settling ponds, and their intergrowths with gold, and/or coatings on the gold, contribute to the tendency of the gold to remain in suspension through the mine processing system. This knowledge of the nature of the fine and super-fine gold may assist in redesign of placer mine processing streams at this site, and elsewhere in the world, to prevent these losses in suspension.
Despite the fine and super-fine particle sizes of the gold in the settling ponds, successful reprocessing of the fine sediments from the settling ponds has been shown to be technically possible, as the samples for this study attest. This reprocessing requires slow gravity settling during constant shaking with abundant clean water. It is unlikely that this reprocessing is economically viable with the present processing plant, but additional stages may be possible in future. However, by being forewarned of the potential value of this mine waste slurry, the mine operation now includes selective stockpiling of the sludge material from the first settling pond for possible future reprocessing, rather than more dispersed disposal. Even if this stockpile is not processed in the current mine life, future miners may be able to process it economically. These observations and mine site experiments have positive significance for processing of placer gold, especially beach gold, elsewhere in the world where the fine and super-fine fractions have commonly been lost. Most placer mines monitor the gold lost to their gravel and sand tailings, but this study identifies another possible pathway for loss of gold suspended in discharge waters.
Acknowledgements
Friendly co-operation of Round Hill miners, especially Ray Stewart, Mark Skinner and Mike McDonald were instrumental in obtaining the unique samples that formed the basis for this study. The miners also patiently explained and demonstrated the workings of the processing system. Noel Becker kindly supplied the magnetic concentrate depicted in . Scanning electron microscopy was carried out at Otago Micro and Nanoscale Imaging (OMNI) facility at University of Otago. Helpful suggestions from two anonymous reviewers improved the presentation of the paper.
Disclosure statement
No potential conflict of interest was reported by the author(s).
Data availability statement
All data relevant to this study are included within this paper or within cited references.
Additional information
Funding
References
- Allan GC, Woodcock JT. 2001. A review of the flotation of native gold and electrum. Minerals Engineering. 14:931–962. doi:10.1016/S0892-6875(01)00103-0.
- Ashley P, Craw D, MacKenzie D, Rombouts M, Reay A. 2012. Mafic and ultramafic rocks, and platinum mineralisation potential, in the Longwood Range, Southland, New Zealand. New Zealand Journal of Geology and Geophysics. 55:3–19. doi:10.1080/00288306.2011.623302.
- Boonchayaanant B, Nayak D, Du X, Criddle CS. 2009. Uranium reduction and resistance to reoxidation under iron-reducing and sulfate-reducing conditions. Water Research. 43:4652–4664. doi:10.1016/j.watres.2009.07.013.
- Boyle RW. 1979. The geochemistry of gold and its deposits. Geological Survey of Canada Bulletin. 280:579.
- Craw D, Kerr G. 2021. Physical and chemical evolution of a Pleistocene marginal marine gold paleoplacer deposit, southern New Zealand. Ore Geology Reviews. 138:104341. doi:10.1016/j.oregeorev.2021.104341.
- Craw D, Kerr G, Reith F, Falconer D. 2015. Pleistocene paleodrainage and placer gold redistribution, western Southland, New Zealand. New Zealand Journal of Geology and Geophysics. 58:137–153. doi:10.1080/00288306.2015.1007462.
- Craw D, McLachlan C, Negrini M, Becker N. 2017. Quantification and prediction of bulk gold fineness at placer gold mines: a New Zealand example. Minerals. 7:226. doi:10.3390/min7110226.
- Craw D, Mitchell M, McCann R, Reay A. 2013. Compositional variations and morphological evolution in platinum beach placers, southern New Zealand. Mineralium Deposita. 48:81–97. doi:10.1007/s00126-012-0417-4.
- Donoso M, Robles PA, Galvez ED, Cisternas LA. 2013. Particle size effect on the efficient use of water and energy in mineral concentration processes. Industrial & Engineering Chemistry Research. 52:17686–17690. doi:10.1021/ie402099n.
- Druzbicka J, Craw D. 2012. Turbidity development and dissipation in paleoplacer gold deposits, southern New Zealand. Environmental Earth Sciences. 68:1575–1589. doi:10.1007/s12665-012-1851-4.
- Edraki M, Baumgartl T, Manlapig E, Bradshaw D, Franks DM, Moran CJ. 2014. Designing mine tailings for better environmental, social and economic outcomes: a review of alternative approaches. Journal of Cleaner Production. 84:411–420. doi:10.1016/j.jclepro.2014.04.079.
- Fraser N. 2011. Technical report for offshore sampling of PP51979, West Coast. Seafield Resources Ltd. Mineral Report Series MR4761, NZ Petroleum and Minerals, Wellington, New Zealand, p. 89.
- Garnett RHT, Bassett NC. 2005. Placer deposits. Economic Geology 100th Anniversary volume, p. 813–843.
- Gul A, Kangal O, Sirkeci AA, Onal G. 2012. Beneficiation of the gold bearing ore by gravity and flotation. International Journal of Minerals, Metallurgy, and Materials. 19:106–110. doi:10.1007/s12613-012-0523-4.
- Guney A, Onal G, Atmaca T. 2001. New aspect of chromite gravity tailings re-processing. Minerals Engineering. 14:1527–1530. doi:10.1016/S0892-6875(01)00165-0.
- Hudson-Edwards KA, Jamieson HE, Lottermoser BG. 2011. Mine wastes: past, present, future. Elements. 7(6):375–380. doi:10.2113/gselements.7.6.375.
- Kinnunen P, Karhu M, Yli-Rantala E, Kivikyto-Reponen P, Makinen J. 2022. A review of circular economy strategies for mine tailings. Cleaner Engineering and Technology. 8:100499. doi:10.1016/j.clet.2022.100499.
- Kungurova VE. 2021. Evaluating the effectiveness of fine gold extraction technologies on the example of titanomagnetite beach placers of the western coast of Kamchatka. Journal of Mining Institute. 252:840–853. doi:10.31897/PMI.2021.6.6.
- Leistner T, Embrechts M, Leissner T, Chehreh Chelgani S, Osbahr I, Mockel R, Peuker UA. 2016. A study of the reprocessing of fine and ultrafine cassiterite from gravity tailing residues by using various flotation techniques. Minerals Engineering. 96–97:94–98. doi:10.1016/j.mineng.2016.06.020.
- Liu S, Chen X, Lauten RA, Peng Y, Liu Q. 2018. Mitigating the negative effects of clay minerals on gold flotation by a lignosulfonate-based biopolymer. Minerals Engineering. 126:9–15. doi:10.1016/j.mineng.2018.06.021.
- Malloch K, Kerr G, Craw D. 2017. Placer gold in the Cretaceous Blue Spur Conglomerate at Waitahuna, southern New Zealand. New Zealand Journal of Geology and Geophysics. 60:239–254. doi:10.1080/00288306.2017.1311931.
- McLachlan C, Negrini M, Craw D. 2018. Gold and associated minerals in the Waikaia placer gold mine, Northern Southland, New Zealand. New Zealand Journal of Geology and Geophysics. 61:164–179. doi:10.1080/00288306.2018.1454482.
- Monte MBM, Lins F, Oliveira JF. 2000. Electrokinetic properties of gold-particles in the presence of several electrolytes and of amyl xanthate. Electrochemistry in Mineral and Metal Processing V: Proceedings of the international symposium. The Electrochemical Society, Pennington, NJ, USA. p. 129–140.
- Palmer M, Craw D. 2023. Morphology and structural evolution of fine beach gold in comparison to detrital platinum, southern New Zealand. Mineralium Deposita. 59:69–83. doi:10.1007/s00126-023-01200-z.
- Palmer M, Craw D. 2024. Detrital gold morphology and recycling around the early Miocene St Bathans paleovalley, Central Otago, New Zealand. New Zealand Journal of Geology and Geophysics. doi:10.1080/00288306.2023.2284281.
- Palmer M, Ritchie T, Craw D. 2024. Contrasting evolution of beach gold on two sides of an active Orogen, Southern Alps, New Zealand. New Zealand Journal of Geology and Geophysics. doi:10.1080/00288306.2024.2314468.
- Pernet-Fisher JF, Day JMD, Howarth GH, Ryabov VV, Taylor LA. 2017. Atmospheric outgassing and native-iron formation during carbonaceous sediment–basalt melt interactions. Earth and Planetary Science Letters. 460:201–212. doi:10.1016/j.epsl.2016.12.022.
- Ritchie T, Scott J, Craw D. 2019. Garnet compositions track longshore migration of beach placers in western New Zealand. Economic Geology. 114:513–540. doi:10.5382/econgeo.4647.
- Ryabov VV, Lapkovsky AA. 2010. Native iron (-platinum) ores from the Siberian Platform trap intrusions. Australian Journal of Earth Sciences. 57:707–736. doi:10.1080/08120091003739056.
- Shuster J, Lengke M, Marquez-Zavalia MF, Southam G. 2016. Floating gold grains and nanophase particles produced from the biogeochemical weathering of a gold-bearing ore. Economic Geology. 111:1485–1494. doi:10.2113/econgeo.111.6.1485.
- Stewart J, Kerr G, Prior D, Halfpenny A, Pearce M, Hough R, Craw D. 2017. Low temperature recrystallisation of alluvial gold in paleoplacer deposits. Ore Geology Reviews. 88:43–56. doi:10.1016/j.oregeorev.2017.04.020.
- Turnbull IM, Allibone AH. 2003. Geology of the Murihiku area. Institute of Geological and Nuclear Sciences 1:250 000 Geological map 20. Lower Hutt, New Zealand.
- Ulff-Moller F. 1990. Formation of native iron in sediment-contaminated magma: I. A case study of the Hanekammen Complex on Disko Island, West Greenland. Geochimica et Cosmochimica Acta. 54:57–70. doi:10.1016/0016-7037(90)90195-Q.
- Upton P, Craw D. 2016. Coeval emplacement and orogen-parallel transport of gold in oblique convergent orogens. Tectonophysics. 693(Part B):197–209. doi:10.1016/j.tecto.2016.01.015.
- Wang C, Harbottle D, Liu Q, Xu Z. 2014. Current state of fine mineral tailings treatment: a critical review on theory and practice. Minerals Engineering. 58:113–131. doi:10.1016/j.mineng.2014.01.018.
- Wang Z, Lee S-W, Kapoor P, Tebo BM, Giammar DE. 2013. Uraninite oxidation and dissolution induced by manganese oxide: A redox reaction between two insoluble minerals. Geochimica et Cosmochimica Acta. 100:24–40. doi:10.1016/j.gca.2012.09.053.
- Webster JG. 1986. The solubility of Au and Ag in the system Au–Ag–S–O2–H2O at 25 °C and 1 atm. Geochimica et Cosmochimica Acta. 50:1837–1845. doi:10.1016/0016-7037(86)90242-5.
- Webster JG, Mann AW. 1984. The influence of climate, geomorphology and primary geology on the supergene migration of gold and silver. Journal of Geochemical Exploration. 22:21–42. doi:10.1016/0375-6742(84)90004-9.
- Williams GJ. 1974. Economic geology of New Zealand. Australasian Institute of Mining and Metallurgy Monograph. 4:490.
- Youngson JH, Wopereis P, Kerr LC, Craw D. 2002. Au-Ag-Hg and Au-Ag alloys in Nokomai and Nevis valley placers, northern Southland and Central Otago, New Zealand, and their implications for placer-source relationships. New Zealand Journal of Geology and Geophysics. 45:53–69. doi:10.1080/00288306.2002.9514959.