Abstract
Placental growth factor (PlGF) and soluble fms-like tyrosine kinase 1 (sFlt-1) are biomarkers used for diagnosis and risk estimation of preeclampsia. Stability in room temperature (RT) may affect the usefulness of these analyses, as shipping at ambient temperature is the most practical and suitable way to ship samples. To date, scientific studies of such stability are lacking. We aimed to assess the stability of PlGF and sFlt-1 at RT in serum from pregnant women. In addition, a smaller study of stability at 4 °C was performed. Serum was collected from 69 pregnant women and stored at RT or at 4 °C for up to 192 h. Analytes were considered stable if the mean percent change ± 90 confidence interval of the mean was within the baseline concentration ± allowable bias. Allowable bias was calculated from data on biological variation. In addition, an instability equation was calculated to assess loss of stability, in line with recent European Federation of Clinical Chemistry and Laboratory Medicine (EFLM) recommendations. The mean percent change was <3.5% for PlGF, <1% for sFlt-1 and <4.5% for sFlt-1/PlGF ratio up to 192 h. PlGF was considered stable for 168 h, and sFlt-1 and sFlt-1/PlGF ratios were considered stable for 192 h at RT. At 4 °C, PlGF was considered stable for 120 h, sFlt-1 for 168 h and sFlt-1/PlGF ratio for 120 h. Both PlGF and sFlt-1 as well as sFlt-1/PlGF ratio show sufficient stability (minimum 168 h) for samples to be shipped at RT.
Introduction
Pre-eclampsia (PE) is a leading cause of maternal and perinatal morbidity and mortality worldwide [Citation1]. Placental dysfunction and an angiogenic imbalance of placental growth factor (PlGF) and soluble fms-like tyrosine kinase 1 (sFlt-1) are central pathophysiological elements in PE [Citation2,Citation3]. sFlt-1, a soluble receptor for PlGF and vascular endothelial growth factor (VEGF), is produced in the placenta, and is a potent antagonist of the pro-angiogenic factors PlGF and VEGF as it binds them in the circulation and prevents interaction with their receptors [Citation3]. Numerous papers have reported increased maternal circulating levels of sFlt-1 and decreased levels of PlGF in PE, as well as an increased sFlt-1/PlGF ratio [Citation3,Citation4]. The concentration of sFlt-1 often correlates with disease severity, and is higher in severe PE compared to mild PE [Citation4]. Clinical guidelines recommend using PlGF together with other clinical risk factors for first trimester PE risk estimation, as low concentration of PlGF is linked to increased risk of PE [Citation5]. Women with high risk (≥1:100) are offered low-dose aspirin, which has been shown to reduce the risk of early onset PE [Citation6]. Later in pregnancy (after week 20), the sFlt-/PlGF ratio can be a useful tool to predict the imminent risk of developing PE, allowing for a closer monitoring of high risk women. A low ratio can be used to rule out PE. Using the ratio is recommended in the National Institute for Health and Care Excellence (NICE) guidelines [Citation7]. Thus, measurements of both PlGF and sFlt-1/PlGF ratio could improve both prediction and diagnosis of PE.
Specialized analyses such as PlGF and sFlt-1 are often performed at central laboratories, requiring shipment of samples. Shipment at ambient temperature (i.e. room temperature, RT) is practically the best way to transport samples, as shipping of frozen samples is cumbersome and expensive, and dry ice can affect assay results [Citation8,Citation9].
To date, only a few studies have assessed the stability of PlGF and sFlt-1 [Citation10–12]. Armstrong-Buisseret et al. investigated stability of both PlGF and sFlt-1 in five serum samples stored at RT, but only for up to 24 h [Citation10]. Cowans et al. investigated stability of PlGF at RT in serum pools made from leftover first trimester samples, and found that PlGF was stable for 3.3 days [Citation11]. Rowson et al. stored whole blood at 4 °C for up to 48 h, and found that stability was acceptable for PlGF and sFlt-1 up to 48 h. However, no assessment of stability at RT was made [Citation12]. For PlGF, the assay manufacturer Thermo Fisher claimed stability at RT for 24 h [Citation13], Perkin Elmer claimed five days stability at RT [Citation14] and Roche provided no data on sample stability at RT [Citation15]. For sFlt-1, Thermo Fisher claimed that sFlt-1 is stable at RT for 72 h [Citation16], whereas Roche provided no stability data at RT [Citation18].
Shipping samples frozen is both unpractical and expensive, and it is important to determine the stability of PlGF and sFlt-1 at RT. This work aimed to determine the stability of PlGF and sFlt-1 at RT in serum from pregnant women. The stability of serum PlGF and sFlt-1 at RT is important to determine, as it has a great impact on the utility of these analyses.
Materials and methods
Pregnant women at various gestational ages (1st to 3rd trimester) were recruited from the hospital’s pregnancy outpatient clinic. Written informed consent was obtained from all participants. Samples were de-identified, and no information regarding any diagnoses and gestational age were collected. Five milliliters of blood was drawn in Vacuette Serum Sep Clot Activator Tube (Greiner Bio-One, Frickenhausen, Germany). Samples were centrifuged at 2200 × g for 10 min at 20 °C, most (87%) within one hour from sample collection and some (13%) within three hours. One aliquot was transferred into a polypropylene tube and immediately put in the freezer (T0). The remaining serum was divided in two; one aliquot was put in a refrigerator (4 °C), and the other kept at RT (22–23 °C). Aliquots for the different time intervals were pipetted into polypropylene tubes (200 μL) and subsequently frozen at 24, 48, 72, 96, 120, 144, 168 and 192 h after sample collection (see Supplementary Figure 1 for a flowchart of the sample collection). If there was limited serum volume available, aliquots for storage at RT was prioritized. After the work on collecting the samples was well underway, we observed that the stability was better than first anticipated and prioritized samples to be stored for longer time intervals. This explains why n is larger at 168 h than at 144 h. When all aliquots from a participant were fully collected, aliquots were thawed simultaneously and analyzed in the same run within two hours after thawing. The majority of samples (85%) were analyzed in duplicate to reduce random analytical variation, and the mean of the two replicates were used in further calculations. Samples from any participant were analyzed within two weeks after completing the sample collection.
Serum sFlt-1 and PlGF concentrations were measured using BRAHMS Kryptor sFlt-1 and PlGF plus assays (Thermo Scientific, Waltham, MA) on a Kryptor compact PLUS analyzer at the Department of Clinical Chemistry, St. Olav’s University Hospital, Trondheim, Norway. The limit of quantitation was 6.91 ng/L for PlGF and 34 ng/L for sFlt-1 (measuring range: sFlt-1, 22–90,000 ng/L; PlGF, 3.6–7000 ng/L). The assays were calibrated against internal reference standards prepared from recombinant human sFlt-1 or PlGF. Internal quality control intermediate coefficients of variation (CV) were <1.7% at 1500 ng/L, <2.2% at 3000 ng/L and <2.4% at 10,000 ng/L for sFlt-1; and <4.9% at 32 ng/L, <3.3% at 90 ng/L and <3.1% at 400 ng/L for PlGF (Thermo Fisher, Waltham, MA). We also evaluated the within-run analytical CV for PlGF at very low concentrations, and found it to be 8.4% at 11.5 ng/L using 24 replicates. For external quality control, the laboratory used the Preeclampsia Marker survey from UK NEQAS.
Figure 1. Concentration of serum PlGF for all samples (A), serum PlGF for 21 samples with concentration <35 ng/L (B), serum sFlt-1 for all samples (C), as well as sFlt-1/PlGF ratio in the full range (D) and in the range close to the recommended decision limit at 66 (E) at various storage times at room temperature.
Mean percent change was calculated from values at baseline (T0) compared to the various storage time points at RT (24, 48, 72, 96, 120, 144, 168 and 192 h). Not all samples were stored in the same time intervals due to practical reasons, as samples were handled during working hours on weekdays. The analyte was considered stable if the mean percent change in concentration ± 90 confidence interval (CI) of the mean was within baseline concentration ± allowable bias, in accordance with national Norwegian recommendations [Citation19]. In addition, we calculated an instability equation in line with the newly published recommendations for the design of stability studies on clinical specimens from the European Federation of Clinical Chemistry and Laboratory Medicine (EFLM) Working Group Preanalytical Phase [Citation20]. More specifically, the mean percent change was fitted with a least squares regression line through the origin, weighted by the number of observations at each time point. The goodness of fit was assessed by R2, and significant deviations from baseline values assessed by testing (with a t-test) whether the slope of the regression line was significantly different from zero.
Figure 2. Mean percentage change of serum PlGF concentration (A), sFlt-1 concentration (B) and sFlt-1/PlGF ratio (C) ±90% confidence intervals at various storage times at room temperature compared to baseline values (T0). The regression line for the instability equation is indicated in black. The horizontal red lines indicate the allowable bias (PlGF: ÷8.5 to 9.3%, sFlt-1: ÷12 to 13.6% and sFlt-1/PlGF ratio ÷15.4 to 18.2%).
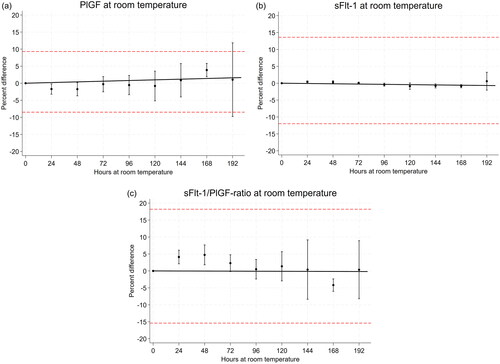
We defined allowable bias or maximum permissible systematic error as 0.25 times the standard deviation of the distribution of reference values from a healthy population, as recommended [Citation21]. Our analytical goal was derived from reference limits from healthy pregnant women [Citation13,Citation16]. Reference values of sFlt-1, PlGF, and the sFlt-1/PlGF ratio may best be described as log-normally distributed [Citation22], so the analytical goal of 0.25 times the standard deviation had to be applied on log-transformed reference values [Citation23,Citation24]. We used the 5- and 95 percentile of the most narrow reference range of each analyte (for sFlt-1 only gestational weeks after week 20), as given by Thermo Fisher [Citation13,Citation16], and calculated the standard deviation on the log scale as ln_sd = (ln(95 percentile) ÷ ln(5 percentile))/3.29 (in a normal distribution there are 3.29 standard deviations between the 5 and 95 percentile). Then, we calculated the allowable ln_bias as +/÷0.25 × ln_sd, which backtransforms to 100 × (exp(ln_bias) ÷ 1) % as the allowable positive bias and 100 × (1/exp(ln_bias) ÷ 1) % as the allowable negative bias on the measurement scale [Citation24]. In this way, we defined the allowable positive and negative bias of sFlt-1 as +13.6% and ÷12.0%, respectively. The corresponding values for PlGF were +9.3% and ÷8.5%, and for the sFlt-1/PlGF ratio +18.2% and ÷15.4%.
Further, to assess the possible clinical impact of a bias equal to the allowable bias for the sFlt-1/PlGF ratio, we used the dataset of Andersen et al. [Citation25, and personal communication June 2022] with logistic regression to derive a function for the likelihood ratio (LR) of the sFlt-1/PlGF ratio [Citation26]. Andersen et al. recommended a cut-off value of 66 [Citation25]. We calculated the LR associated with a sFlt-1/PlGF ratio of 66, and compared that value with the LRs associated sFlt-1/PlGF ratio of 66 ÷ 15.4% = 56 and 66 + 18.2% = 78.
Results
A total of 69 serum samples from pregnant women were collected. The concentration range was 9–916 ng/L for PlGF, 49–20,940 ng/L for sFlt-1 and 0.8–1432 for the sFlt-1/PlGF ratio (). At RT, the mean percent change in concentration was <3.5% for PlGF, <1% for sFlt-1 and <4.5% for sFlt-1/PlGF ratio for up to 192 h (eight days) (). At 4 °C, the mean percent change was <5% for PlGF up to 120 h and <7.5% up to 168 h. The mean percent change in concentration was <2.3% for sFlt-1 and <6.5% for sFlt-1/PlGF ratio up to 192 h (eight days), except for the sFlt-1/PlGF ratio at 144 h which was 8.5%. (Supplementary Table 1), wich has fallen out of the manuscript:According to national recommended criteria for stability [19], where stability is considered acceptable if the mean percent change in concentration ± 90 % CI of the mean is within mean baseline value ± allowable bias, we found PlGF to be stable for 168 hours, and sFlt-1 and sFlt-1/PlGF ratio to be stable for 192 hours at RT (, ).
Table 1. Changes in serum PlGF and sFlt-1 concentrations in samples stored at room temperature at various times compared to baseline concentrations (T0).
At 4 °C, PlGF was stable for 120 h, sFlt-1 for 168 h and sFlt-1/PlGF ratio for 120 h, using the criteria for stability as described above (Supplementary Table 1 and Figure 2). Using the instability equation proposed by EFLM [Citation20], we found no significant loss of stability in RT up to 192 h for PlGF and sFlt-1/PlGF ratio (p = .23 and .91, respectively). For sFlt-1, the slope of the regression line was −0.004 (p = .049, r2 = 0.4), i.e. marginally significantly different from zero. At 4 °C, a decrease in PlGF concentration (−0.043, p < .01, r2 = 0.96) and increase in sFlt-1/PlGF ratio (0.047, p < .01, r2 = 0.88) were observed. No significant loss of stability was observed for sFlt-1 for up to 168 h at 4 °C, p = .72 (Supplementary Figure 1). By using the PE dataset from Andersen et al. [Citation25], we calculated the LR associated with a sFlt-1/PlGF cutoff at 66 ± allowable bias. The LR of PE was 2.0 with no bias at cutoff 66, 2.5 with 18.2% positive bias and 1.7 with 15.4% negative bias.
Discussion
The stability of serum PlGF and sFlt-1 at RT is important to determine, as it has a great impact on the utility of these analyses. In this work, we found that both serum PlGF and sFlt-1 as well as the sFlt-1/PlGF ratio were stable for at least 168 h at RT. By using the instability equation proposed by EFLM [Citation20], both PlGF and sFlt-1/PlGF ratio was stable for 192 h, but as we only have three samples at 192 h, stability beyond 168 h should be interpreted with caution. We report considerably longer stability of sFlt-1 at RT than Armstrong-Buisseret et al. who only investigated stability for up to 24 h in five samples [Citation10]. Thermo Fisher found that sFlt-1 was stable for 72 h at RT, using 10 samples and allowing up to 10% mean change in concentration from baseline [Citation17].
Regarding PlGF, Thermo Fisher claim 24 h stability at RT [Citation13], whereas Perkin Elmer claim five days [Citation14], both using less than 10% mean change in concentration from baseline as a criterion for stability. Armstrong-Buisseret et al. only investigated stability of PlGF for 24 h at RT [Citation10], whereas Cowans et al. found that PlGF was stable for 3.3 days at 20 °C, with shorter stability (1.1 day) at higher temperatures (30 °C) and longer stability at 2–4 °C, using pools of first trimester serum samples and less than 10% mean change as a criterion [Citation11]. Our results, with RT stability for at least 168 h are in line with these data, but we used stricter criteria for stability. Cowans et al. [Citation11] and others [Citation13,Citation14] postulated that PlGF concentrations increase over time, possibly due to the dissociation of PlGF bound to sFlt-1. We found no such pattern of increased PlGF concentration over time at RT. This pattern was not obvious in other publications either [Citation10,Citation12]. However, it is possible that a dissociation of PlGF from sFlt-1 occurs simultaneously with a degradation of PlGF, possibly eradicating this pattern, or that the different antibodies used have varying affinity for the degradation products.
In whole blood, the reported stability of PlGF at RT is relatively short at only 19 h [Citation11], but longer if kept refrigerated before serum separation [Citation12]. At 2–4 °C, PlGF is reported to be stable for at least 30 days [Citation11] and sFlt-1 up to seven days [Citation27]. In line with this, we found that PlGF was stable for 120 h (five days), and that sFlt-1 and sFlt-1/PlGF ratio was stable for at least 168 h (seven days) at refrigerator temperature. Serum sFlt-1 and PlGF are reported to be stable for at least three years stored at ÷80 °C [Citation28], and for up to seven freeze–thaw cycles [Citation27].
There are no universally accepted criteria to define sample stability, and a wide variety of statistical approaches are used to assess stability. These include testing for statistically significant changes in mean analyte concentration over time [Citation12], or demanding that the average concentration at each time point should be within baseline value ± 1 analytical standard deviation [Citation29]. Such criteria for stability are rarely justified. We have previously published a paper on determination of analyte stability [Citation30], arguing that the mean concentration ± the 90% CI of the mean should be within baseline concentration ± allowable bias, for analytical results to retain their supposed clinical usefulness, which also provides at least a 95% probability of detecting a change greater than the allowable bias.
In previously published data assessing PlGF and sFlt-1 stability, a ±10% mean change in concentration from baseline is most frequently used as a stability criterion for PlGF and sFlt-1 [Citation10,Citation11,Citation27,Citation28]. We believe that using total biological variation to determine allowable bias is a more appropriate approach. So far, only one study has assessed the biological variation of PlGF and sFlt-1 [Citation31]. If we had used these estimates of intra- and interindividual biological variation to determine the total biological variation, the maximum allowable bias would have been 6% for PlGF and 3% for sFlt-1 [Citation31]. This is unfeasible as the analytical CV alone is 8% and 3%, respectively, for these analyses in the lower concentration ranges. Such studies of the components of the total biological variation often include relatively few individuals. In addition, samples in the study from Braga et al. [Citation31] were from non-pregnant women and may not be representative for biological variation in pregnancy. We therefore chose to use the reference values from a healthy pregnant population [Citation22] to determine the total biological variation. We defined allowable bias as 0.25 times the standard deviation of the distribution of reference values [Citation21,Citation24], which amounted to an allowable positive and negative bias of sFlt-1 as +13.6% and ÷12.0%, respectively. The corresponding values for PlGF were +9.3% and ÷8.5%, and for the sFlt-1/PlGF ratio +18.2% and ÷15.4%. The allowable bias of sFlt-1/PlGF should have virtually no clinical consequences, as changes in LR from 2.0 to 2.5 (18.2% positive bias) or from 2.0 to 1.7 (15.4% negative bias) are relatively small differences on the LR scale [Citation32]. Recently, the EFLM Working Group Preanalytical Phase (WG-PRE) published recommendations for the design and evaluation of stability studies on clinical specimens, recommending an instability equation to model the change in stability [Citation20]. Using this approach, we found no significant loss of stability up to 192 h for both PlGF and sFlt-1/PlGF ratio at RT, and a very small decrease (−0.004% per hour) in sFlt-1 concentration, of little clinical significance. However, we only had three samples at 192 h, and stability beyond 168 h should be interpreted with caution.
Scientific studies of PlGF and sFlt-1 stability are scarce, and so far, none has investigated sFlt-1 stability for more than 24 h. We believe this is the largest study of stability for sFlt-1 and PlGF at RT, using 69 samples from a clinically relevant population of pregnant women in 1st to 3rd trimester, providing data in a broad concentration range for both analytes. Overall, sFlt-1 and PlGF in serum kept at RT was stable for at least 168 h, which allows for sample shipment at RT.
Ethical approval
The study was carried out in full accordance with the ethical principles of the Declaration of Helsinki. According to the Norwegian Health Research Act, this type of method validation and quality assurance study does not require approval by the Regional Ethical Committee (REC).
Supplemental Material
Download PDF (206.7 KB)Disclosure statement
No potential conflict of interest was reported by the author(s).
Data availability statement
For access to data, contact the corresponding author.
Additional information
Funding
References
- Duley L. The global impact of pre-eclampsia and eclampsia. Semin Perinatol. 2009;33(3):130–137. doi: 10.1053/j.semperi.2009.02.010.
- Young BC, Levine RJ, Karumanchi SA. Pathogenesis of preeclampsia. Annu Rev Pathol. 2010;5(1):173–192. doi: 10.1146/annurev-pathol-121808-102149.
- Erez O, Romero R, Jung E, et al. Preeclampsia and eclampsia: the conceptual evolution of a syndrome. Am J Obstet Gynecol. 2022;226(2S):S786–S803. doi: 10.1016/j.ajog.2021.12.001.
- Wikström AK, Larsson A, Eriksson UJ, et al. Placental growth factor and soluble FMS-like tyrosine kinase-1 in early-onset and late-onset preeclampsia. Obstet Gynecol. 2007;109(6):1368–1374. doi: 10.1097/01.AOG.0000264552.85436.a1.
- Poon LC, Shennan A, Hyett JA, et al. The international federation of gynecology and obstetrics (FIGO) initiative on pre-eclampsia: a pragmatic guide for first-trimester screening and prevention. Int J Gynaecol Obstet. 2019;145(Suppl. 1):1–33. doi: 10.1002/ijgo.12802.
- Rolnik DL, Wright D, Poon LC, et al. Aspirin versus placebo in pregnancies at high risk for preterm preeclampsia. N Engl J Med. 2017;377(7):613–622. doi: 10.1056/NEJMoa1704559.
- NICE (National Institute for Health and Care Excellence). PlGF-based testing to help diagnose suspected pre-eclampsia. Diagnosis guidance 23; 2022 [cited 2022 May 15]. Available from: https://www.nice.org.uk/guidance/dg23
- Murphy BM, Swarts S, Mueller BM, et al. Protein instability following transport or storage on dry ice. Nat Methods. 2013;10(4):278–279. doi: 10.1038/nmeth.2409.
- Trondsetås L, Mikkelsen G, Lian IA. The effects of dry ice exposure on plasma pH and coagulation analyses. Clin Chem Lab Med. 2017;56(1):59–64. doi: 10.1515/cclm-2017-0263.
- Armstrong-Buisseret LK, Haslam S, James T, et al. Verification of placental growth factor and soluble-fms-like tyrosine kinase 1 assay performance in late pregnancy and their diagnostic test accuracy in women with reduced fetal movement. Ann Clin Biochem. 2020;57(3):223–233. doi: 10.1177/0004563220911993.
- Cowans NJ, Alfthan H, Stenman UH, et al. Stability of first trimester placental growth factor in serum and whole blood. Prenat Diagn. 2011;31(12):1193–1197. doi: 10.1002/pd.2894.
- Rowson S, Reddy M, Rolnik DL, et al. Stability of placental growth factor, soluble fms-like tyrosine kinase 1, and soluble fms-like tyrosine kinase 1 e15a in human serum and plasma. Placenta. 2019;86:1–3. doi: 10.1016/j.placenta.2019.08.001.
- Thermo Fisher Scientific. BRAHMS PlGF plus Kryptor. Product insert. Hennigsdorf: Thermo Fisher Scientific GmbH; 2018.
- Perkin Elmer PlGF application note. Waltham: Perkin Elmer; 2011 [cited 2022 Jun 2]. Available from: https://resources.perkinelmer.com/corporate/pdfs/downloads/samplestabilityofplgf.pdf
- Roche Diagnostics. Elecsys PlGF cobas. Product insert. Mannheim: Roche Diagnostics GmbH; 2020.
- Thermo Fisher Scientific. BRAHMS sFlt-1Kryptor. Product insert. Hennigsdorf: Thermo Fisher Scientific GmbH; 2018.
- Thermo Fisher Scientific. Prenatal screening – compendium. Analytes – PlGF, s-Flt-1, PAPP-A, free ßhCG, AFP. Handling, stability, storage. Hennigsdorf: Thermo Fisher Scientific GmbH; 2021.
- Roche Diagnostics. Elecsys sFlt-1 cobas. Product insert. Mannheim: Roche Diagnostics GmbH; 2021.
- Noklus; 2022 [cited 2022 Oct 15]. Available from: https://www.noklus.no/helsepersonell-sykehus-ogprivatelaboratorier/holdbarhetsdatabase/utfore-holdbarhetsforsok/
- Gomez-Rioja R, Von Meyer A, Cornes M, et al. Recommendation for the design of stability studies on clinical specimens. Clin Chem Lab Med. 2023;61(10):1708–1718. doi: 10.1515/cclm-2023-0221.
- Gowans EM, Hyltoft Petersen P, Blaabjerg O, et al. Analytical goals for the acceptance of common reference intervals for laboratories throughout a geographical area. Scand J Clin Lab Invest. 1988;48(8):757–764. doi: 10.3109/00365518809088757.
- Hirashima C, Ohkuchi A, Arai F, et al. Establishing reference values for both total soluble fms-like tyrosine kinase 1 and free placental growth factor in pregnant women. Hypertens Res. 2005;28(9):727–732. doi: 10.1291/hypres.28.727.
- Hyltoft Petersen P, Gowans EM, Blaabjerg O, et al. Analytical goals for the estimation of non-Gaussian reference intervals. Scand J Clin Lab Invest. 1989;49(8):727–737. doi: 10.3109/00365518909091551.
- Åsberg A, Lian IA, Mikkelsen G. TSH: biased estimate of allowable bias. Clin Chem Lab Med. 2022;60(11):e241–e242. doi: 10.1515/cclm-2022-0791.
- Andersen LLT, Helt A, Sperling L, et al. Decision threshold for Kryptor sFlt-1/PlGF ratio in women with suspected preeclampsia: retrospective study in a routine clinical setting. J Am Heart Assoc. 2021;10(17):e021376.
- Albert A. On the use and computation of likelihood ratios in clinical chemistry. Clin Chem. 1982;28(5):1113–1119. doi: 10.1093/clinchem/28.5.1113.
- Higgins V, Ma L, Ragosnig BL, et al. Analytical performance and quality indicators of fully automated immunoassays for sFlt-1 and PlGF. J Appl Lab Med. 2022;7(2):555–567. doi: 10.1093/jalm/jfab135.
- Law LW, Sahota DS, Chan LW, et al. Effect of long-term storage on placental growth factor and fms-like tyrosine kinase 1 measurements in samples from pregnant women. J Matern Fetal Neonatal Med. 2010;23(12):1475–1480. doi: 10.3109/14767051003678242.
- Thiers RE, Wu GT, Reed AH, et al. Sample stability: a suggested definition and method of determination. Clin Chem. 1976;22(2):176–183. doi: 10.1093/clinchem/22.2.176.
- Åsberg A, Solem KB, Mikkelsen G. Prøvematerialets holdbarhet – kriterier og vurderinger. Klinisk Biokemi i Norden. 2011;23:34–38.
- Braga F, Ferraro S, Borille S, et al. Biological variation of two serum markers for preeclampsia prediction. Clin Chem Lab Med. 2020;58(2):e27–e28. doi: 10.1515/cclm-2019-0649.
- Grimes DA, Schulz KF. Refining clinical diagnosis with likelihood ratios. Lancet. 2005;365(9469):1500–1505. doi: 10.1016/S0140-6736(05)66422-7.