Abstract
The synthesis of nine new carbohydrate-based quaternary ammonium salts and two new triazolium salts starting from d-glucose has been accomplished. Our synthesis utilized the regio- and stereoselective ring opening reaction of 2,3-anhydro sugars by nucleophilic reagents to afford the key intermediates. In these new types of phase transfer catalysts, the ammonium and triazolium functions are directly attached to the carbohydrate scaffold in different positions (2-, 3-, 6-positions of the sugar). The efficiency of the altrose- and glucose-based quaternary salts were tested in the alkylation of N-(diphenyl) methylene glycine tert-butyl ester with benzyl bromide. To our knowledge this is the first example when sugar-based quaternary ammonium or triazolium salts were used successfully as phase transfer catalysts. The enantiomeric recognition ability of the synthesized salts towards racemic Mosher’s acid silver salt was also investigated by 19F NMR spectroscopy.
Graphical Abstract
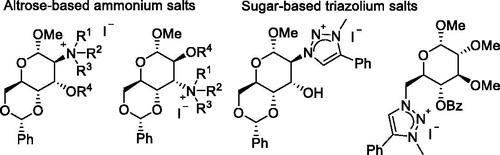
Introduction
Phase transfer catalysis has been long recognized as a powerful tool for organic synthesis both in the industry and academia, as it offers several advantages, such as operational simplicity, mild reaction conditions, inexpensive and environmentally benign reagents and solvents and suitability for large-scale synthesis.[Citation1] Over more than the past thirty years asymmetric phase transfer catalysis, based on chiral, non-racemic catalysts has become an attractive protocol for the synthesis of optically active compounds.[Citation2] The most common and efficient type of chiral phase transfer catalysts are the quaternary ammonium salts. Cinchona alkaloid-derived, TADDOL-derived, binaphthyl-based and many other types of ammonium salts have all been successfully used in various reactions with excellent enantioselectivities.[Citation3] More recently Ooi and coworkers synthesized amino acid-derived 1,2,3-triazolium salts. These new types of phase transfer catalysts generated high asymmetric induction in some alkylation reactions.[Citation4] Despite the numerous reported catalysts, the development of new and efficient chiral phase transfer catalysts is still a challenging area in current organic chemistry. Carbohydrate derivatives have been widely used for stereochemical control as chiral auxiliaries,[Citation5] ligands,[Citation6] organocatalysts,[Citation7] and phase transfer catalysts.[Citation8] Building the sugars into the chiral catalyst has several advantages: carbohydrates used as starting materials are in most cases inexpensive and easily available commercial products; they are biocompatible and available in enantiomerically pure form with known chiroptical properties. Carbohydrates have functionalities which can be used to establish secondary binding sites, as well as catalytic sites. These sugar-based compounds may be more readily biodegradable, although the biological activity of most synthetic phase transfer catalysts has not been tested. In our group monoaza-15-crown ethers annelated to different sugar units were synthesized and applied as phase transfer catalysts. These chiral macrocycles proved to be highly enantioselective in some reactions.[Citation9] In this work we intended to spread the scope of our research to carbohydrate-based quaternary ammonium salts.
In the literature only a limited number of articles are dealing with this type of compounds. Most often the sugar-based quaternary ammonium salts are used as ionic liquids.[Citation10] Malhotra and coworkers synthesized isomannide-derived quaternary ammonium salts and examined their chiral recognition ability towards racemic Mosher’s acid silver salt by NMR spectroscopy.[Citation11] Later, Reddy achieved remarkable chiral recognition in the same experiment with a xylose-derived imidazolium-based ionic liquid.[Citation12] Vo-Thanh applied isosorbide-based ammonium salts in an aza-Diels-Alder reaction both as solvent and as catalyst with moderate diastereoselectivity.[Citation13] The synthesis and characteristics of some other ionic liquids derived from glucose, arabinose, xylose and ribose have also been reported.[Citation14]
Sugar-based surfactants have aroused much attention due to the fact that they are biodegradable, very mild to the skin, and are derived from renewable sources. Surfactants derived from glucose, gluconamide and lactobionic acid have been synthesized and their surface chemical and antibacterial properties were investigated.[Citation15] Dmochowska and coworkers tested the mutagenicity of some glucose-based quaternary ammonium salts.[Citation16] Triazolium salts derived from glucose and xylose have also been synthesized and used as solvent in the amination of aryl halides.[Citation17]
Up to now, there are no examples of using carbohydrate-based ammonium or triazolium salts as catalyst, although some of the sugar-based ionic solvents had catalytic role in certain reactions, but these compounds have been used in excess, as solvent, so these ionic liquids cannot be considered as real catalysts. The aim of our research was to design new phase transfer catalysts starting from cheap and naturally occurring monosaccharides and to investigate the catalytic properties of these compounds. In these molecules the catalytic center (ammonium or triazolium function) is connected directly to the carbohydrate scaffold, thus the enantioselectivity in the catalyzed reactions can be influenced by the sugar unit. The possible effect arising from the chirality of the carbohydrate was taken into consideration in the design, by placing the quaternary center at various positions of the carbohydrate unit. Thus the synthesis of compounds having ammonium or triazolium functions in positions 2, 3, and 6 were planned.
Results and discussion
Synthesis of sugar-based quaternary ammonium and triazolium salts
For the preparation of catalysts having ammonium function in position 2, methyl 2,3-anhydro-4,6-O-benzylidene-α-d-allopiranoside (1) was selected as starting material, which was synthesized in our laboratory in four steps starting from d-glucose in high yields according to literature procedure.[Citation18] The oxirane ring of allopyranoside 1 was opened with three different amines (butylamine, 3,4-diethoxyphenylethylamine, and morpholine, respectively) affording altropyranoside-derivatives 2a–c, following the procedure elaborated by Iglesias-Guerra (Scheme 1).[Citation19] The reactions took place in a regio- and stereoselective manner, according to the Fürst–Plattner rule,[Citation20] to provide the products (2a–c) in good yields (78–91%) after recrystallization.
We assumed that the substituent in the third position of the sugar unit may have an important role in the generation of asymmetric induction, because it is close to the quaternary center. Therefore in two cases the third OH group was left free, and in some cases it was alkylated with either ethyl bromide or benzyl bromide. The alkylation of the OH group was performed with 2 equivalents of alkylating agent, in boiling THF or DMF (70 °C) using NaH as the base (Scheme 1) to afford products 3a–c in excellent yields (91–98%). It is noteworthy that the reactions were completely selective for the O-alkylation under these conditions. Formation of N-alkylated products could not be observed, despite the fact that the alkylating agents were used in excess.
Intermediates 2a, 2c and 3a–c were further converted to tertiary amines and quaternized with methyl iodide in one step, in boiling acetonitrile in the presence of K2CO3 (Scheme 1). In most cases reactions were complete in 10 h; however, in the quaternization of compound 2a and 2c full conversion was not achieved even after boiling for 40 h and further addition of methyl iodide (2 equivalents per day). These two products were separated from the starting materials by aqueous extraction giving onium salts 4a and 4d in moderate yield (75% and 66%, respectively). In all other cases no purification was needed. The targeted ammonium salts (4b, 4c, 4e) were isolated in pure form after standard work-up procedure in excellent yields (95–98%). We intended to synthesize other derivatives of these compounds by using different alkylating agents (ethyl iodide, benzyl bromide, allyl bromide), however, the quaternizations were unsuccessful, only tertiary amines could be isolated, which can be explained by steric hindrance.
The starting material for the synthesis of altrose-based onium salts having the ammonium function in position 3 was the methyl 2,3-anhydro-4,6-O-benzylidene-α-d-mannopyranoside (5), which was synthesized starting from d-glucose in four steps, in our laboratory according to literature methods.[Citation21] The ring opening of mannopyranoside 5 with amines, as expected,[Citation22] resulted in 3-amino altropyranose derivatives. The reactions were performed with butylamine and morpholine, in boiling acetonitrile, in the presence of LiClO4, giving intermediates 6a and 6c in good yields (88% and 89%, respectively) after column chromatography (Scheme 2). Amino sugar 6a was then O-alkylated with ethyl bromide applying the same method as mentioned before, giving compound 6b with excellent yield (98%). In the final step compounds 6a and 6c were methylated and quaternized with methyl iodide. As in the case of molecule 4d full conversion was not reached in the alkylation of 6c even after 40 h of reflux and daily addition of extra methyl iodide, therefore ammonium salt 7b had to be separated by aqueous extraction from the starting material. This process afforded product 7b in moderate yield (61%). Onium salt 7a could be obtained in pure form after standard work-up procedure in good yield (92%).
We also envisioned the synthesis of a diammonium salt in which two altrose units are linked via an ethylene bridge and the quaternary centers are in positions 2. To accomplish that two altrose units were first connected by the ring opening reaction of allopyranoside 1 with ethylenediamine. The reaction was performed in 2:1 stoichiometry (anhydrosugar:amine) applying the same reaction conditions as before (Scheme 3). Product 8 was obtained in 77% yield after column chromatography. Thereafter we intended to perform methylation and quaternization of the nitrogen atoms in compound 8 with methyl iodide, however, the solubility of compound 8 was poor even in dipolar aprotic solvents, therefore, no quaternary product could be isolated. To improve the solubility of compound 8 the free OH-groups were alkylated with ethyl bromide in DMF (Scheme 3). Since no byproducts were observed, intermediate 9 was obtained in excellent yield (97%) without any further purification. The methylation and quaternization of diamine 9 was performed using the same conditions as before, applying large excess of methyl iodide and refluxing the mixture for 40 h. The main product of the reaction was isolated by column chromatography; however, the 1H NMR analysis clearly demonstrated that instead of the expected bisquaternary product only the monoquaternary salt 10 was formed. Further attempts of using even larger excess of methyl iodide, longer reaction time and applying DMF instead of acetonitrile all failed to provide the desired diammonium salt, nevertheless onium salt 10 can also be used as catalyst.
The synthesis of the glucosamine-based ammonium salt 13 was also targeted. With this compound we intended to study the role of the sugar unit in the catalysts. The two hexopyranoses are of similar structure, only the configuration at C-2 and at C-3 alters: in the α-d-glucopyranoside- and α-d-altropyranoside-units the C-centers mentioned can be described as 2R,3S, and 2S,3R, respectively, which may have a significant influence on the effectiveness of the catalysts. For the preparation of ammonium salt 13 methyl-4,6-O-benzylidene-α-d-glucosamine (11) was chosen as starting material, which was synthesized according to literature procedure.[Citation23] First the OH group of compound 11 was alkylated with ethyl bromide, using the abovementioned method, in 99% yield (Scheme 4). The alkylation was again completely selective for the OH group, despite using an excess of the alkylating agent. Amino sugar 12 was then reacted with methyl iodide in boiling acetonitrile affording quaternary salt 13 in a pure form, in good yield (91%).
After the successful synthesis of the ammonium salts our attention turned toward carbohydrate-based 1,2,3-triazolium salts. Recently increasing attention has been focused on triazolium-based chiral phase transfer catalyst,[Citation4] due their unique properties and easy accessibility via copper(I)-catalyzed Huisgen 1,3-dipolar cycloaddition of terminal alkynes with organic azides, which represents one of the prime examples of click chemistry.[Citation24] For the preparation of triazolium salt 16 having the triazolium function in position 2, oxirane ring of allopyranoside 1 was opened with NaN3.[Citation25] Azido sugar 14 was then reacted with phenylacetylene in the presence of CuSO4 and sodium ascorbate in DMF. The pure triazolium derivative 15 was formed in a yield of 89% without any further purification after the work-up procedure. In the final step intermediate 15 was alkylated with methyl iodide in boiling acetonitrile. After 4 days of reflux and daily addition of extra methyl iodide the conversion was not complete, therefore column chromatography was necessary to separate the product from the starting compound. Triazolium salt 16 was obtained in 65% yield after the purification (Scheme 5).
Finally, we intended to synthesize the glucose-based catalyst 19 having the triazolium ring in position 6. Azido sugar 14 was synthesized according to literature procedure in five steps starting from d-glucose,[Citation26] which was then reacted with phenylacetylene in the copper(I)-catalyzed Huisgen 1,3-dipolar cycloaddition, affording intermediate 18 in good yield (84%). This compound was reacted with methyl iodide providing the targeted triazolium salt 19 after chromatography in 59% yield (Scheme 6).
Application of the sugar-based quaternary salts in the alkylation of glycine-derived schiff base 20
The catalytic activity of the new carbohydrate-based ammonium and triazolium salts were tested in the alkylation of glycine-derived Schiff base 20 with benzyl bromide. This model reaction has been widely studied by different groups using cinchona alkaloids, cyclohexyldiamine salts and TADDOL-based catalysts.[Citation3a,b,Citation27] The reactions were performed at 0 °C in a liquid–liquid two phase system, applying a mixture of toluene and 50% aqueous NaOH solution in the presence of 10 mol% catalyst. The crude products were purified using preparative TLC in all cases. The enantiomeric excesses were determined using chiral HPLC analysis, in comparison with authentic racemic material. The results are summarized in .
Table 1. Alkylation of glycine-derived Schiff base 20 with benzyl bromide catalyzed by carbohydrate-based ammonium and triazolium salts.
It can be seen from , that all synthesized quaternary salts proved to be efficient phase transfer catalysts. Product 22 was obtained in moderate and good yields (67–88%) in every case. The new catalysts favored the formation of the product with S configuration, the enantioselectivity, however, was low (2–21% ee). The most promising result was achieved with altrose-based ammonium salt 4e, which generated 21% asymmetric induction. This example clearly demonstrate that carbohydrate-based quaternary salts can be used as enantioselective phase transfer catalysts, although more experiments have to be made in order to improve the achieved ee values. Further investigation of the catalytic activity of prepared quaternary salts in different reactions and optimization of the reaction conditions is in progress in our laboratory.
The investigation of the enantiomeric recognition ability of the synthesized quaternary salts by 19F NMR spectroscopy
Besides testing the new catalysts in asymmetric synthesis we also decided to study the enantiomeric recognition ability of the new ammonium and triazolium salts by investigating the diastereomeric interaction between racemic Mosher’s acid silver salt and the new carbohydrate-based salts. The prepared iodide salts and racemic Mosher’s acid silver salt were dissolved in CD3CN and the mixture was stirred for 30 min, then the precipitated silver-iodide was filtered. The remaining solution was examined using 19F NMR spectroscopy. The diastereomeric interaction between the ammonium and triazolium cation and Mosher’s carboxylate resulted in the splitting of the CF3 signal. The difference, in chemical shift (Δδ), of the two signals describes the enantiomeric recognition ability of the applied salts. The results are summarized in .
Table 2. The enantiomeric recognition ability of carbohydrate-based ammonium and triazolium salts toward racemic Mosher’s acid studied using 19F NMR spectroscopy.
It can be seen from that the structure of the carbohydrate-based salts had a significant effect on the Δδ values. The highest Δδ (9 Hz) was observed in case of compound 4d. It is interesting, that analog 6b having the morpholino function in position 3 did not show enantiomeric recognition toward Mosher’s acid (Δδ < 1 Hz). Another notable result is the 4.7 Hz chemical shift difference generated by ammonium salt 13, however, in all other cases the synthesized sugar-based salts showed poor enantiomeric recognition. Compound 10 could not be tested in this experiment, because no precipitation was formed after mixing iodide salt 10 and Mosher’s acid silver salt. Presumably the tertiary amine function of salt 10 prevented the silver-iodide from precipitating by complexation.
Conclusion
The synthesis of a few new carbohydrate-based ammonium and triazolium salts was elaborated in several steps. The ammonium or triazolium functions were placed in different positions of the altrose and glucose scaffold in order to examine structure-efficiency relationship of the new catalysts. The synthesized quaternary salts promoted the alkylation of a glycine-derived Schiff base, although the achieved enantioselectivities were low. However the 21% asymmetric induction generated by catalyst 4e demonstrates that this type of compounds can be used as enantioselective catalyst, although further research and optimization is needed to improve their efficiency. The 19F NMR study of the sugar-based ammonium or triazolium Mosher’s carboxylate salts also confirmed that some of these new compounds (4d and 13) have notable chiral recognition ability.
Experimental
General
Melting points were determined by using a Stuart SMP10 apparatus and are uncorrected. The specific rotation was measured on a Perkin Elmer 341LC polarimeter at 22 °C. Nuclear magnetic resonance spectra were obtained on a Bruker (Billerica, MA) DRX-500 or Bruker 300 instrument in CDCl3, DMSO-d6, CD3CN or CD3OD with Me4Si as an internal standard. The exact mass measurements were performed by using quadrupole time of flight mass spectrometer Premier mass spectrometer (Waters, Milford, MA) in positive electrospray ionization mode. Analytical and preparative thin layer chromatography (TLC) was performed on silica gel plates (60 GF 254, Merck, Darmstadt, Germany), while column chromatography was carried out by using 70 to 230 mesh silica gel (Merck). The eluent rations are always given in vol/vol. The chemicals were purchased from Aldrich Chemical (Milwaukee, WI).
General procedure for the ring opening reaction of anhydrosugars 1 and 5
The mixture of anhydrosugar 1 or 5, LiClO4 (2 equivalents), and the appropriate amine (4 equivalents) was dissolved in dry CH3CN and refluxed for 8 h. After the completion of the reaction (followed by TLC) the solvent was evaporated in vacuum and the residue was dissolved in CHCl3, washed with water, dried (Na2SO4) and concentrated. The crude product was purified by either recrystallization from EtOH or column chromatography.
General procedure for the O-ethylation of amino sugars 2a, 6a and 11
The appropriate amino sugar was dissolved in dry THF, and 2 equivalents of NaH was added. After stirring the mixture for 30 min on 50 °C, 2 equivalents of ethyl bromide was added and the mixture was refluxed for further 10 h. After the completion of the reaction water was added dropwise (3 equivalents to the amount of NaH) and the solvent was removed under reduced pressure. The residue was dissolved in CHCl3, washed with water, dried (Na2SO4) and concentrated, giving the O-alkylated products in pure form.
General procedure for the O-alkylation of amino sugars 2a–b and 8
The appropriate amino sugar was dissolved in dry DMF, and 2 equivalents of NaH was added. After stirring the mixture for 30 min on 50 °C, 2 equivalents of the alkylating agent (ethyl bromide or benzyl bromide) was added and the mixture was refluxed for 10 h. After the completion of the reaction water was added dropwise and the aqueous phase was washed three times with EtOAc. The combined organic layers were dried (Na2SO4) and concentrated, giving the O-alkylated products in pure form.
General procedure for the quaternization of amino sugars
The appropriate amino sugar was dissolved in dry CH3CN. Methyl iodide (tertiary amines: 3 equivalents, secondary amines: 6 equivalents, primary amines: 9 equivalents) and K2CO3 (tertiary amines: 0 equivalents, secondary amines: 2 equivalents, primary amines: 4 equivalents) was added. The reaction mixture was refluxed for 1-4 days. Additional amounts (2 equivalents) of methyl iodide were added every day. After the reactions were complete (followed by TLC) the mixture was filtered and the solvent was removed under reduced pressure. The purification processes of the different ammonium salts altered from here on and are mentioned at the appropriate compounds below.
General procedure for the huisgen cycloaddition of azido sugars (14, 17) and phenylacetylene
The appropriate azido sugar, phenylacetylene (1.1 equivalent), CuSO4•5H2O (0.08 equivalent) and sodium ascorbate (0.35 equivalent) was dissolved in DMF and the mixture was stirred at ambient temperature. After the completion of the reaction (followed by TLC) water was added and the product was extracted with EtOAc three times. The combined organic layers were dried (Na2SO4) and concentrated.
General procedure for the quaternization of triazolo sugars
The appropriate triazolo sugar was dissolved in dry CH3CN and methyl iodide (3 equivalents) was added. The reaction mixture was kept at boiling temperature for 40 h. Additional amounts (2 equivalents) of methyl iodide were added every day. After that the mixture was filtered and the solvent was removed under reduced pressure. The crude products were purified by column chromatography on silica gel.
General procedure for the benzylation of N-(diphenyl)methylene glycine tert-butyl ester (20)
The mixture of N-(diphenyl)methylene glycine tert-butyl ester (20) (148 mg, 0.5 mmol), the appropriate carbohydrate-based salt (0.05 mmol, 0.1 equivalent) in toluene (3 ml) was treated sequentially with benzyl bromide (94 mg, 0.55 mmol) and 50% aq. NaOH and the mixture was stirred for 5-8 h, at 0 °C. The two phases were separated and the organic layer was washed with water three times, dried on Na2SO4 and concentrated. The crude product was purified on preparative TLC affording the desired product as colorless oil. The enantiomeric excesses were determined by measuring the specific rotation of the products and comparing it with data for the pure enantiomer obtained from literature.[Citation27]
19F NMR experiments
The racemic Mosher’s acid silver salt (0.015 mmol) and the appropriate sugar-based quaternary salt (0.015 mmol) was dissolved in CD3CN. The mixture was stirred for 30 min, then filtered. The remaining solution was transferred to NMR tube and 19F NMR spectrum was recorded.
Full experimental detail, characterization data of all compounds can be found via the “Supplementary Content” section of this article’s webpage.
Additional information
Funding
References
- (a) Dehmlow, E. V.; Dehmlow, S. S. Phase Transfer Catalysis; VCH: Weinheim, 1993. (b) Starks, C. M.; Liotta, C. L.; Halpern, M. Phase-Transfer Catalysis; Chapman & Hall: New York, 1994. (c) Sasson, Y.; Neumann, R. Handbook of Phase-Transfer Catalysis; Blackie Academic & Professional: London, 1997. (d) Halpern, M. E. Phase-Transfer Catalysis; American Chemical Society: Washington, DC, 1997.
- (a) Donnell, M. I. O. Asymmetric Phase-Transfer Reactions. In Catalytic Asymmetric Synthesis; Ojima, I., Eds.; Wiley-VCH: New York, 2000, pp. 231–280. (b) Marouka, K. Asymmetric Phase Transfer Catalysis; Wiley‐VCH Verlag GmbH & Co. KgaA: Weinheim, 2008.
- (a) Ooi, T.; Maruoka, K. Angew. Chem. Int. Ed. 2007, 46, 4222–4266. ‐ DOI: 10.1002/anie.200601737. (b) Maruoka, K.; Shirakawa, S. Angew. Chem. Int. Ed. 2013, 52, 4312–4348. ‐ DOI: 10.1002/anie.201206835. (c) Schörgenhumer, J.; Tiffner, M.; Waser, M. Beilstein J. Org. Chem. 2017, 13, 1753–1769. DOI: 10.3762/bjoc.13.170.
- (a) Ohmatsu, K.; Kiyokawa, M.; Ooi, T. J. Am. Chem. Soc. 2011, 133, 1307–1309. DOI: 10.1021/ja1102844. (b) Ohmatsu, K.; Goto, A.; Ooi, T. Chem. Commun. 2012, 48, 7913–7915. DOI: 10.1039/c2cc32398b. (c) Ohmatsu, K.; Ando, Y.; Ooi, T. J. Am. Chem. Soc. 2013, 135, 18706–18709. DOI: 10.1021/ja411647x. (d) Ohmatsu, K.; Furukawa, Y.; Nakaguro, D.; Ooi, T. Chem. Lett. 2015, 44, 1350–1352. DOI: 10.1246/cl.150581.
- (a) Kunz, H.; Ruck, K. Angew. Chem. Int. Ed. Engl. 1993, 32, 336–358. DOI: 10.1002/anie.199303361. (b) Boysen, M. M. K. 2013, Carbohydrates – Tools for Stereoselective Synthesis; Wiley-VCH Verlag GmbH & Co. KGaA: Weinheim.
- (a) Lehnert, T.; Özüduru, G.; Grugel, H.; Albrecht, F.; Telligmann, S. M.; Boysen, M. M. K. Synthesis 2011, 17, 2685–2708. DOI: 10.1055/s-0030-1260143. (b) Henderson, A. S.; Bower, J. F.; Galan, M. C. Org. Biomol. Chem. 2016, 14, 4008–4017. DOI: 10.1055/s-0030-1260143.
- Phillips, A. M. F. Eur. J. Org. Chem. 2014, 2014, 7291–7303. DOI: 10.1002/ejoc.201402689.
- (a) Bakó, P.; Keglevich, G.; Rapi, Z.; Toke, L. Coc. 2012, 16, 297–304. DOI: 10.2174/138527212799499877. (b) Bakó, P.; Keglevich, G.; Rapi, Z. Loc. 2010, 7, 645–656. DOI: 10.2174/138527212799499877. (c) Bakó, P.; Rapi, Z.; Keglevich, G. Period. Polytech. Chem. Eng. 2015, 59, 51–58. DOI: 10.3311/PPch.7308.
- (a) Rapi, Z.; Démuth, B.; Keglevich, G.; Grűn, A.; Drahos, L.; Sóti, P. L.; Bakó, P. Tetrahedron: Asymmetr. 2014, 25, 141–147. DOI: 10.1016/j.tetasy.2013.12.007. (b) Rapi, Z.; Nemcsok, T.; Pálvölgyi, Á.; Keglevich, G.; Grün, A.; Bakó, P. Chirality 2017, 6, 257–252. DOI: 10.1002/chir.22678. (c) Rapi, Z.; Grün, A.; Keglevich, G.; Stirling, A.; Bakó, P. New J. Chem. 2016, 40, 7856–7865. DOI: 10.1039/C6NJ02030E. (d) Rapi, Z.; Nemcsok, T.; Grün, A.; Pálvölgyi, Á.; Samu, G.; Hessz, D.; Kubinyi, M.; Kállay, M.; Keglevich, G.; Bakó, P. Tetrahedron 2018, 74, 3512–3526. DOI: 10.1016/j.tet.2018.04.087.
- (a) Marra, A.; Chiappe, C.; Mele, A. Chimia. (Aarau) 2011, 65, 76–80. DOI: 10.2533/chimia.2011.76. (b) Thruong, T.-K.-T.; Van Buu, O. N.; Aupoix, A.; Pégot, B.; Vo-Thanh, G. Cos. 2012, 9, 53–64. DOI: 10.2174/157017912798889143.
- (a) Kumar, V.; Olsen, C. E.; Schaffer, S. J. C.; Parmar, V. S.; Malhotra, S. V. Org. Lett. 2007, 9, 3905–3908. DOI: 10.1021/ol071390y. (b) Kumar, V.; Pei, C.; Olsen, C. E.; Schaffer, S. J. C.; Parmar, V. S.; Malhotra, S. V. Tetrahedron: Asymmetr. 2008, 19, 664–671. DOI: 10.1016/j.tetasy.2008.02.009.
- Jayachandra, R.; Reddy, S. R. RSC Adv. 2016, 6, 39758–39761. DOI: 10.1039/C6RA02792J.
- Van Buu, O. N.; Vo-Thanh, G. Loc. 2007, 4, 158–167. DOI: 10.2174/157017807780737219.
- (a) Poletti, L.; Chiappe, C.; Lay, L.; Pieraccini, D.; Polito, L.; Russo, G. Green Chem. 2007, 9, 337–341. DOI: 10.1039/b615650a. (b) Plaza, P. G. J.; Bhongade, B. A.; Singh, G. Synlett 2008, 19, 2973–2976. DOI: 10.1055/s-0028-1087343. (c) Pernak, J.; Czerniak, K.; Biedziak, A.; Marcinkowska, K.; Praczyk, T.; Erfurt, K.; Chrobok, A. RSC Adv. 2016, 6, 52781–52789. DOI: 10.1039/C6RA06703D.
- (a) Nyuta, K.; Yoshimura, T.; Tsuchiya, K.; Ohkubo, T.; Sakai, H.; Abe, M.; Esumi, K. Langmuir 2006, 22, 9187–9191. DOI: 10.1021/la061688h. (b) Torigoe, K.; Tasaki, A.; Yoshimura, T.; Sakai, K.; Esumi, K.; Takamatsu, Y.; Sharma, S. C.; Sakai, H.; Abe, M. Colloid Surface A 2008, 326, 184–190. DOI: 10.1016/j.colsurfa.2008.05.036. (c) Gan, C.; Wang, H.; Zhao, Z.; Yin, B. J. Surfact. Deterg. 2014, 17, 465–470. DOI: 10.1007/s11743-014-1562-9. (d) Parus, A.; Framski, G. Turk. J. Chem. 2018, 42, 1095–1104. DOI: 10.3906/kim-1711-35.
- (a) Dmochowska, B.; Piosik, J.; Woziwodzka, A.; Sikora, K.; Wiśniewski, A.; Węgrzyn, G. J. Hazard. Mater. 2011, 193, 272–278. DOI: 10.1016/j.jhazmat.2011.07.064. (b) Dmochowska, B.; Sikora, K.; Woziwodzka, A.; Piosik, J.; Podgórska, B. Beilstein J. Org. Chem. 2016, 12, 1434–1439. DOI: 10.3762/bjoc.12.138.
- (a) Jha, A. K.; Jain, N. Tetrahedron Lett. 2013, 54, 4738–4741. DOI: 10.1016/j.tetlet.2013.06.114. (b) Ferlin, N.; Gatard, S.; Van Nhien, A. N.; Courty, M.; Bouquillon, S. Molecules 2013, 18, 11512–11525. DOI: 10.3390/molecules180911512.
- Richtmeyer, N. K. Methods Carbohydr. Chem. 1962, I, 107.
- Vega-Perez, J. M.; Candela, J. I.; Vega, M.; Iglesias-Guerra, F. Carbohyd. Res. 1995, 279, C5–C8. DOI: 10.1016/0008-6215(96)83597-X.
- Fürst, A.; Plattner, P. A. Hca. 1949, 32, 275–283. DOI: 10.1002/hlca.19490320139.
- Hicks, D. R.; Fraser-Reid, B. Synthesis 1974, 1974, 203–204. DOI: 10.1055/s-1974-23284.
- Willams, N. R. Adv. Carbohyd. Chem. Bi. 1970, 25, 109–179. DOI: 10.1016/S0065-2318(08)60427-8.
- Goyal, N.; Cheuk, S.; Wang, G. Tetrahedron 2010, 66, 5962–5971. DOI: 10.1016/j.tet.2010.05.071.
- (a) Kolb, H. C.; Finn, M. G.; Sharpless, K. B. Angew. Chem. Int. Ed. 2001, 40, 2004–2021. DOI: 10.1002/1521-3773(20010601)40:11 < 2004::AID-ANIE2004 > 3.0.CO;2-5. (b) Wu, P.; Fokin, V. V. Aldrichim. Acta 2007, 40, 7–19. http://www.scopus.com/inward/record.url?scp=33947526772&partnerID=8YFLogxK. (c) Moses, J. E.; Moorhouse, A. D. Chem. Soc. Rev 2007, 36, 1249–1262. DOI: 10.1039/B613014N.
- Walvoort, M. T. C.; Moggré, G.-J.; Lodder, G.; Overkleeft, H. S.; Codée, J. D. C.; van der Marel, G. A. J. Org. Chem. 2011, 76, 7301–7315. DOI: 10.1021/jo201179p.
- (a) Fuller, T. S.; Stick, R. V. Aust. J. Chem. 1980, 30, 2509–2515. DOI: 10.1071/CH9802509. (b) Ágoston, K.; Fügedi, P. Carbohyd. Res 2014, 389, 50–56. DOI: 10.1016/j.carres.2013.12.026.
- (a) Corey, E. J.; Xu, F.; Noe, M. C. J. Am. Chem. Soc. 1997, 119, 12414–12415. DOI: 10.1021/ja973174y. (b) Ooi, T.; Kameda, M.; Maruoka, K. J. Am. Chem. Soc. 1999, 121, 6519–6520. DOI: 10.1021/ja991062w. (c) Gratzer, K.; Waser, M. Synthesis. (Stuttg) 2012, 44, 3661–3670.doi: 10.1055/s-0032-1316804