Abstract
This study covers the literature documents available on the chemistry of preparation of thiazoles. Additionally, we cover the biological activities of certain thiazoles. The information of several artificial paths and varied physico-chemical factors of such thiazols made especial consideration of medicinal chemists to yield combinatorial library and carry out thorough efforts in the search of thiazoles
Graphical Abstract
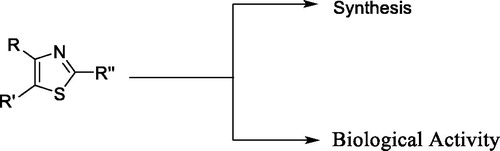
Keywords:
Introduction
Thiazoles are organic five-aromatic ring compounds with a general formula of C3H3NS. Free thiazole is a light-yellow liquid with an odor similar to pyridine.
Resonating structures of thiazole is considered as the following. Still, some resonating assemblies are similarly possible with the involvement of d-orbitals of sulfur ().
The thiazole nucleus is part of the vitamin B (thiamine) structure. Thiazoles are used to obtain free carbene particles and complexed with transition metals, while their salts are used as catalysts in the reaction of Stetter and benzoin condensation.[Citation1,Citation2]
Thiazoles occupy potent biological applications, described by Hantzsch and Weber for the first time in 1887.[Citation3] It is showed similar chemical and physical properties to pyridine and pyrimidine, although some of their derivatives behave similarly to the thiophene and furan. The molecular electrostatic potential (MEP) points out that nitrogen is the most negative atom compared to carbon and sulfur, which are neutral.[Citation4]
Thiazole and correlated are one of the supreme significant potential things in the mainly increasing chemical world of compounds having thiazoles showing notable pharmacological actions. The current survey highlights a wide sight on the preparation and biological actions of materials having thiazole moiety ().[Citation5]
One of the highest reasons for death in the world is due to cancer; and several materials have been prepared for expenditure in cancer therapy. Thiazoles are an adaptable heterocycle present in the several drugs are used in the cure of cancer.[Citation6]
Aryl thiazoles were prepared and evaluated for their anticancer actions.[Citation7] The (thiazol-2-yl)hydrazones were prepared and elucidated via elemental analytical.[Citation8] Thiophenylpyrazolyl-5-substituted-aryl-diazenylthiazoles were prepared from the reaction of 3-(2-Thienyl)-5-aryl-1-thiocarbamoyl-2-pyrazolines with hydrazonyol.[Citation9]
Derivatives of 4,5,6,7-tetrahydrobenzo[b]thiophene-3-carbohydrazides has been synthesized and elucidated.[Citation10]
Synthesis of thiazoles
Thiazole 8 itself can be obtained by condensing α-chloroacetaldehyde 5 and thioformamide 6 in excellent yield (Scheme 1).[Citation11,Citation12]
The reaction of a thioamide 10 with α-halocarbonyl 9 compounds has been used extensively, and several thiazoles 11 with alkyl, aryl, arylalkyl or heteroaryl functional groups at 2-, 4- or 5-positions have been reported (Scheme 2).[Citation11,Citation12]
The cyclization of two moles of 2-bromo-1-phenylethan-1-one 9 with benzene-1,4-bis(carbothioamide) 12 give rise to in 1,4-bis(4-phenylthiazol-2-yl)benzene 13 in excellent yield (Scheme 3).[Citation13]
Thiazolium salts 18 can be obtained successfully by a modification of the Hantzsch’s thiazole synthesis. This method is particularly valuable for those thiazolium compounds in which the substituent on the ring nitrogen cannot be introduced by direct alkylation, for example, aryl or heteroaryl thiazolium salts.
N-Monosubstituted thioamides 15 have been cyclized with α–halocarbonyl compounds 14 to give thiazolium salt in excellent yields (Scheme 4).[Citation14-18]
The most efficient involves the condensation of equimolar parts of thiourea 17 and α–haloketones or α–haloaldehydes 14 to yield the corresponding 2-aminothiazoles 18 with no by-products (Scheme 5).[Citation19]
The cyclization of N-substituted thioureas 9 with halocarbonyl compounds 19 gives 2-monosubstituted aminothiazoles 20 (Scheme 6).[Citation19]
N,N-disubstituted thioureas 21 reacted with α-halocarbonyl 14 to yield 2-disubstituted aminothiazoles 22 but in lower yields[Citation20-23] (30–70%) (Schemes 7 and 8).
Scheme 7. Synthesis of 2-disubstituted aminothiazoles 22 his method, initiated by Marchesini,[Citation23,Citation81] in 1893 based on the reaction of an 1-chloropropan-2-one 14 with O-methyl carbamothioate 23 gave 2-hydroxythiazole derivatives 24.
![Scheme 7. Synthesis of 2-disubstituted aminothiazoles 22 his method, initiated by Marchesini,[Citation23,Citation81] in 1893 based on the reaction of an 1-chloropropan-2-one 14 with O-methyl carbamothioate 23 gave 2-hydroxythiazole derivatives 24.](/cms/asset/fd952e49-7e34-4f27-bed6-68481630b153/lsyc_a_1854787_sch0007_c.jpg)
The cyclization of α-thiocyanatoketones 25 in aqueous acid medium, concentrated sulfuric acid in acetic acid and water or alkaline solution gave 2-hydroxy thiazoles 26 after dilution in water. These reactions can be carried out for some hours at room temperature or by heating at steam bath (Scheme 9).[Citation24-28]
Treatment of 2-chlorobenzothiazole 28 with thiourea 27 gave benzo[d]thiazol-2-yl carbamimidothioate 29 (Scheme 10).[Citation29]
Treatment carbon disulfide 31 with α-aminonitriles 30 to give 2-mercapto-5-amino thiazoles 32 (Scheme 11).[Citation30-33]
By condensing of dithioformic 10 with α-aminonitriles 33, 5-aminothiazoles 35 were obtained in good yields.Citation34]] These reactions were carried out in aqueous ethereal solution at room temperature (Scheme 12).
This reaction was first described by GabrielCitation35]] in 1910, when reacted with N-(2-oxo-2-phenylethyl)acetamide 36 with an equimolecular amount of phosphorus pentasulfide to yield 2-methyl-5-phenylthiazole 38 (Scheme 13).
4-Ethyl-5-methyl-2-phenylthiazole 41 prepared in fairly low yield (16 to 40%) by the action of 2-mercaptopentan-3-one 39 with benzonitrile 40 (Scheme 14).[Citation36]
The reaction of thiourea 17 with an 2-bromo-2-phenylacetonitrile 42 in alcohol yield 5-phenylthiazole-2,4-diamine 44 (Scheme 15).[Citation37–39]
5-Methyl-N-phenylthiazol-2-amine 46 can be organized by intramolecular nucleophilic substitutionreaction of 1-(2-bromoallyl)-3-phenylthiourea 45 (Scheme 16).[Citation40]
Treatment of methyl benzoate derivatives 47 and with phenylglycine diversity 49 of thiazoles 50 were synthesized in good to moderate yields using the following protocol (Scheme 17).[Citation41]
Hassan et al. synthesized a group of thiazole derivatives (53, 57 and 58) and studied their biological properties (Scheme 18).[Citation42]
The thiazoles 61 were obtained by the acylation of thiosemicarbazide 60 reacted with a series of α-halogenocarbonyls 59a–f () in acetone or DMF/acetone (Scheme 19).[Citation43,Citation44]
Table 1. α-Halogenocarbonyls compounds.
Bueno et al. used the Hantzsch method to construct the thiazole 65 (Scheme 20).[Citation45]
Filho et al. applied Hantzsch method to prepare thiazoles 68 and 70 (Schemea 21 and 22).[Citation46]
Arafa et al. prepared thiazoles by the reaction of 2,6-diformylphenol derivatives 73 which resulted from the reaction of 4-substituted phenols 71 with hexamethylenetetramine 72 in TFA as a solvent. Furthermore, dialdehyde 73 reacted with thiosemicarbazide 74 gave bis-thiosemicarbazones 75. Then, according to Hantzsch's reaction, condensation of bis-thiosemicarbazones 75 with phenacyl bromide derivatives 76 yield thiazoles 77 (Scheme 23).[Citation47]
Furthermore, refluxing of bis-thiosemicarbazones 78 with ethyl chloroacetate 79 in ethanol with the presence of potassium carbonate as a catalyst gave bis-thiazolidinone 82 derivatives. In addition, Treatment of bis-thiosemicarbazones 78 with chloroacetonitrile 80 in ethanol and a catalytic amount of triethylamine gave bisaminothiazolidines 83. Finally, the reaction of bis-thiosemicarbazones 78 with diethyl acetylenedicarboxylate 81 in ethyl acetate yield bis-thiazolones 84 (Scheme 24).[Citation47]
Laczkowski et al. prepared their compounds by the reaction of cyclopropane-carboxaldehyde 85 with thiosemicarbazide 74 to give 2-(Cyclopropylmethylidene)-hydrazinecarboxamide 86. The reaction was done in ethanol in the presence of acetic acid as a catalyst. Then, the reaction of 2-(Cyclopropylmethylidene)-hydrazinecarboxamide 86 with p-substituted bromoacetophenones yield the thiazole compounds 88 (Scheme 25).[Citation48]
In addition, thiazoline derivatives 92 were obtained from the reaction of thioacetamide 89 with dimethyl acetylenedicarboxylate 90 (Scheme 26). The final product was invistigated according to the literature survey[Citation49-52] and the spectral data
The reaction of nitro alkenes 93 with thiobenzamides 95 gave thiazoles derivatives 95 (Scheme 27).[Citation43]
Turan-Zitouni et al. synthesized bisthiazole derivatives 100 to study their properties as an anticancer. The preparation of these compounds was done in two steps, the first step involved the synthesis of 1,1′-(3,3′-dimethoxybiphenyl-4,4′-diyl)bis(thiourea) 98 from the reaction of 3,3′-dimethoxybiphenyl-4,4′-diamine 98 with potassium thiocyanate 97 with a catalytic amount of hydrochloric acid. The next step was the reaction of 1,1′-(3,3′-dimethoxy-[1,1′-biphenyl]-4,4′-diyl)bis(thiourea) 98 with phenacyl bromides 99 furnished the desired thiazoles 100 (Scheme 28).[Citation53]
Treatment of ethyl 2-phenylthiocarbamoyl acetate 101 with α-bromoketones 102 in ethanol yields thiazole derivatives 103 (Scheme 29).[Citation54]
Kim et al. used the Lawesson’s reagent (LR) to convert N-Boc-piperidine-4-carboxamide 104 to N-Boc-piperidine-4-carbothioamide 106 which was reacted with 1,3-dichloroacetone 107 to give the thiazole 108 (Scheme 30).[Citation55]
Thiazole derivatives 113 were obtained by the reaction of 4′-trifluoromethyl-benzaldehyde 109 with thiosemicarbazide 110 in the presence of hydrochloric acid as a catalyst. Then, reacted 111 with various α-halogenated ketones 112 and yield thiazole derivatives 113 (Scheme 31).[Citation56]
Steroid thiazoles 116 and 118 were synthesized from the reaction of steroidal ketones 114 or 117 with thiosemicarbazide 74 and then with phenacyl bromide 115 (Scheme 32).[Citation57]
Bis-thiazoles 122 and 125 were prepared by the reaction of 3-hexylthiophene 119 with lithium 2,2,6,6-tetramethylpiperidide 120 to give 4-hexylthiophene-2-carbaldehyde 121 then was reacted with dithiooxamide 51 and gave the desired product 1,1′-(thiazolo[5,4-d]thiazole-2,5-diylbis(thiophene-5,3-diyl))bis(hexan-1-one) 122. Similarly, 1,1′-(benzo[1,2-d:4,5-d']bis(thiazole)-2,6-diylbis(thiophene-5,3-diyl))bis(hexan-1-one) 125 was prepared using 2,5-diaminobenzene-1,4-dithiol bis-hydrochloride 124 instead of dithiooxamide 51 (Scheme 33).[Citation58]
Abu-Zaied et al. synthesized thiazole 131 by condensation of cyanamide 126 and carbon disulfide in a solution of ethanol and potassium hydroxide to give compound 127, which reacts easily with phenacyl bromide and ethyl bromoacetate to produce potassium 4-amino-5-substituted-thiazole-2-thiolates derivatives 128.[Citation59]
Adding acid on 128 gives 3-mercaptothiazole derivatives 129 while adding tetra-O-acetyl-α-D-glucopyranosyl bromide and tetra-O-acetyl-α-D-galactopyranosyl bromide 130 in DMF gives thiazole S-glycosides 131 in a good yield (Scheme 34).[Citation59]
Farag et al. used the potassium salts method to prepare thiazole compound 136. The initial materials were 3-oxo-N-(pyridin-2-yl)butanamide 132 and phenyl isothiocyanate, which reacted with KOH in DMF to give the corresponding salt 133. Following that, a reaction of the salt with 1-phenyl-2- bromoethanone produce the intermediate compound 134. The ring closure occurs via a nucleophilic attack from the amine group on the carbonyl group to give a compound 135 followed by losing of water molecule afforded the thiazole derivative 136 (Scheme 35).[Citation60]
Sayed prepared bis-thiazoles via a reaction of bis-hydrazonoyl chlorides 137 and 138 with thiosemicarbazone derivatives 139–143. The reactions were carried out in dioxane in the presence of triethylamine and heated to 105 0C to give the corresponding products with high yields 144–148 (Scheme 36).[Citation61] On the same methodology, in 2017 Gomha et al. introduced new bis-thiazoles 156–162 using different thiosemicarbazones 149–155 (Scheme 37).[Citation62]
3-(4-Dimethylaminophenyl)-1-phenylpropenone 163 was cured with thiosemicarbazide 74 in ethanol containing catalytically amount of triethylamine provided 5-(4-dimethylaminophenyl)-3-phenyl-4,5-dihydropyrazol-1-carbothioic acid amide 164.[Citation63] The compound 164 reacted with hydrazonoyl halides 165 gave 167
In alike manner, when compound 170 was allowable to react with the ethyl (N-arylhydrazono)-chloroacetates 165 in sutiable solvent and in the presence of triethylamine, it gave a single isolable produce, namely, 2-(3-(4-fluorophenyl)-1,3,4,5,6-pentahydrobenzo[6,7] cyclohepta[1,2-c]pyrazol-2-yl)-5-(arylhydrazono)thiazol-4(5H)-one 172 (Scheme 39).[Citation64]
Farghaly et al. prepared thiazole derivatives starting by preparing 175 from the reaction of thiochroman-4-one 173 and thiocarbohydrazide 174 in ethanol with hydrochloric acid as a catalyst. Reacting of thiochroman-4-thiocarbohydrazone 175 with hydrazonoyl chlorides derivatives 165 gaves thiazole compounds 177 (Scheme 40).[Citation65]
In the same way, using different types of hydrazonoyl chlorides 178, reacted with thiochroman-4-thiocarbohydrazone 175 to give the compounds 180 (Scheme 41).[Citation65]
3-(4-(Dimethylamino)benzylidene)thiochroman-4-one 181 was reacted with thiosemcarbazide 74 to give 3-(4-(dimethylamino)phenyl)-3,4-dihydrothiochromeno[4,3-c] pyrazole-2(1H)-carbothioamide 182. The last carbothioamide derivative 184 reacted with hydrazonoyl chlorides 165 and 178 to provide the thiazole derivatives 183 and 184, respectively (Scheme 42).[Citation66,Citation67]
Biological activities
Many organic compounds with biological activity contain thiazole fragments as a part of their structure. Thiazoles are found in numerous natural products such as the secondary metabolites in marine organisms. The biological activities of these NPs have been evaluated and studied as leading structures to manufacture new drugs. For example, latrunculin A derivatives are biologically measured as inhibitors for a prostate tumor and HIF-1 activators for a breast tumor.[Citation68,Citation69]
Secondary metabolites in marine organisms
Thiazoles are of great importance in pharmacology for their presence in most therapeutic agents. The biological activity of compounds containing thiazole is almost infinite, they are antineoplastic 188, 189, antibiotic 190, anti-inflammatory agents 191, 192, antiulcer 192, 193, anti-HIV 194, antimicrobial 195, antifungal drugs 196 and many more.[Citation70]
Most biologically active thiazole-containing compounds have substitutions at positions 2 and 4 or one of them. It was found that these substitutes give the compounds different biological properties depending on the difference of the substitutes themselves.[Citation70]
Antitumor activity
Marini et al. studied the effect of thiazoles implication on inactive compounds such as transplatin 197.[Citation71]
Ethyl 2-[3-(diethylamino)-propanamido]-thiazole-4-carboxylate 198 was tested against RPMI-8226 leukemia cell line and showed a noticeable activity in this filed.[Citation70]
Compound 199 and 200 were synthesized and evaluated biologically against cancerous tumors and found to be a good inhibitor for human cancer cell lines. These compounds are derivatives of 2-amino-4-ferrocenyl-5 (1H-1,2,4-triazole-1-yl)−1.3-thiazole.[Citation70]
Zaharia et al. synthesized several bis-thiazoles derivatives and studied their biological activity against the most common types of cancers, prostate and liver cancer (hepatocellular carcinoma). It was found that compounds 201–205 exhibited remarkable activity against previously mentioned types of cancers.[Citation44]
Turan-Zitouni et al. compared the biological activity of compound 206 and its analogue 207–216 to measure the effect of the presence of thiazole ring in the anticancer agents. It was found that the insertion of thiazole fragment into a bis-thiourea derivatives made it more active against A549 (human lung adenocarcinoma) and C6 (rat glioma) cell lines.[Citation53]
Havrylyuk et al. prepared several compounds from thiazolidinones and benzothiazoles. Their biological activities were evaluated on nine types of cancers; ovarian, lung, leukemia, melanoma, colon, renal, CNS, prostate and breast cancers. Compounds 217 and 218 were very active against the CNS cancer SF-295 cell line, compound 219 showed significant activity on renal cancer RXF 393 cell line, while compounds 220 and 221 exhibited wide, high activity that included all cancers under investigation.[Citation72]
Liu et al. studied anticancer activity of thiazole compounds. It was found that compounds 222 and 223 had a high activity against the non-solid human CEM cell line and a moderate with the solid human cancer cell lines. The researchers reviewed several factors influencing the effectiveness of the compounds and found that the presence of the 3,4,5-trimethoxy phenyl on the nitrogen atom, near the carbonyl group, enhance the efficiency. Moreover, the existence of an electron-donating group (3-amino) on the other ring also had a positive effect on the activity.[Citation73]
The compounds 224 and 225 were evaluated against different types of cancer cells. Compound (1-(4- substituted phenyl)-3-(thiazol-2-yl)urea) 224 was found to have an average inhibitory activity for leukemia P388 tumor in mice, while the compound (1-(thiazol-2-yl)-3-(1,1,1-trifluoro-2-(trifluoromethyl)butan-2-yl)urea) 225 exhibited excellent activity against prostate cancer (PC-3).[Citation74]
Anti-inflammatory activity
Kouatly et al. prepared compounds featuring thiazole 226 within its structure to measure its anti-inflammatory properties.[Citation75]
Mohareb et al. prepared a group of compounds containing thiazole ring and measured its activity against inflammation.[Citation76]
Also, Compounds 230–234 showed a significant anti-inflammatory activity.[Citation77]
Anticonvulsant activity
The compounds (235–237) exhibited high anticonvulsant activity when the activity was measured in mice using the maximal electroshock (MES) and pentylenetetrazole (PTZ) models.[Citation78]
The compound 238 was synthesized by Azam et al. screened for anticonvulsant activity. It exhibited high efficiency.[Citation79]
Evaluation of compounds (124a–j) using PTZ, MES and 6-Hz showed that the derivatives 124f and 124i exhibit the highest activity in the PTZ model. On the other hand, compounds 124c and 124e recorded higher efficiency in the MES method. Only compound 124i showed activity in the 6-Hz test.[Citation48]
Siddiqui et al. prepared several thiazole compounds and the most of them showed anticonvulsant activity in the MES and PTZ tests. However, compounds 239c and 239f were higher activity in MES with no toxicity, while 239b, 239k and 239f is the most active in the PTZ model.[Citation80]
Antibacterial activity
Compound 240 was studied for its biological properties and was found to inhibit the growth of two bacteria, Methicillin-resistant Staphylococci (MRSA) and Vancomycin-resistant Enterococci (VRE). Compound 241, which contain a benzenesulfonamide fragment, was prepared for biological purposes and tested against Gram-positive/negative bacteria. This compound was found to have potent properties against Gram-positive bacteria such as Staphylococci, Streptococci, and Bacillus. The hybrid compounds of two molecules known for their biological activity, thiazole and chalcone, were synthesized 242. These compounds have shown high activity against Gram-negative bacteria Enterococcus faecalis.[Citation68] In 2015, a nontoxic and nonhemolytic compound 243, which has the same active previous moieties, was prepared and assayed for its biological properties. It displayed inhibition activity against Gentamicin-resistant and Methicillin-resistant S. aureus.[Citation70]
Compound 244 was prepared and evaluated biologically. It recorded a significant activity against Escherichia coli, Aspergillus niger, Bacillus subtilis, Candida albicans, and Staphylococcus aureus. Compounds (245–247), which contain 2,5-dichlorothiophene or 2,4-dichlorophenyl substitutes, exhibit higher activity.[Citation70]
Biological evaluation of compounds (248a–e) against Gram-positive bacteria (B. subtilis and S. aureus) showed excellent activity.[Citation4] Compound 249 was synthesized and assayed against S. aureus and K. pneumonia. It showed noteworthy antibacterial activity.[Citation28] The compound 154a was active against E. coli.[Citation65]
Antifungal activity
2,4-Disubstituted thiazole derivatives 250 and 251 were prepared and measured for their biological activity with different microbes.[Citation70]
Phytophthora capsici causes damage to agricultural crops. It can be treated by compounds (252–256). These secondary metabolites isolated and evaluated for their biological activity and found to be an excellent antifungal.[Citation68]
Farghaly et al. studied the biological properties of compounds 154a and 159b. These showed an anti-fungal activity against Syncephalastrum racemosum (SR) higher than the well-known drug Amphotericin B and similar to Gentamicin.[Citation65]
Laczkowski et al. evaluated compounds 124a–j against fungi and found them very effective.[Citation48]
Conclusion
The foregoing literature survey[Citation1-93] indicates clearly those thiazoles easily to synthesis based on wide variety of starting materials. Thiazoles are biologically active and highly desirable.
Additional information
Funding
References
- Sayed, A. R.; Wiggins, J. S. Polymer 2008, 49, 2253–2259. DOI: 10.1016/j.polymer.2008.02.038.
- Siddiqui, N. S.; Arya, K.; Ahsanb, W.; Azad, B. IJDDR 2011, 3, 55–67.
- Hantzsch, A.; Weber, J. Ber. Dtsch. Chem. Ges. 1887, 20, 3118–3132. DOI: 10.1002/cber.188702002200.
- Moradi, A.; Peyghan, A.; Hashemian, S.; Baei, M. Bull. Korean Chem. Soc. 2012, 33, 3285–3292. DOI: 10.5012/bkcs.2012.33.10.3285.
- Nosrat, O. M.; Fateme, G. P. Phosphorus Sulf. Silicon. 2016, 191, 811–843.
- Xie, X.-X.; Li, H.; Wang, J.; Mao, S.; Xin, M.-H.; Lu, S.-M.; Mei, Q.-B.; Zhang, S.-Q. Bioorg. Med. Chem. 2015, 23, 6477–6485. DOI: 10.1016/j.bmc.2015.08.013.
- Omar, A. M.; Bajorath, J.; Ihmaid, S.; Mohamed, H. M.; El-Agrody, A. M.; Mora, A.; El-Araby, M. E.; Ahmed, H. E. A. Bioorg. Chem. 2020, 101, 103992 DOI: 10.1016/j.bioorg.2020.103992.
- Zou, X.-Z.; Feng, A.-S.; Liao, Y.-Z.; Xu, X.-Y.; Wen, H.-Y.; You, A.; Mei, M.; Li, Y. Inorg. Chem. Commu. 2020, 118, 108030. DOI: 10.1016/j.inoche.2020.108030.
- Safaa, I. E.; Mansour, E.; Nassar, I. F.; Mekawey, A. A. I. Russ. J. Bioorg. Chem. 2020, 46, 382–392. DOI: 10.1134/S1068162020030061.
- Mohareb, R. M.; Wardakhan, W. W.; Abbas, N. S. Anticancer. Agents Med. Chem. 2019, 19, 1737–1753. DOI: 10.2174/1871520619666190402153429.
- Willstatter, R.; Wirth, T. Berichte 1909, 42, 1908–1922.
- Process for the production of thiazoles, Otte Hrematka, Darmstadt, Germany, assignor to Merk & Co, Inc., Rahway, N. J., Corpoaration of new Jersey, Application September 23,1937, serial No.165413. In September 26, 1936, U.S. Patent No. 2160867; Chem. Abstr. 1939, 33, 7320
- Mulvaney, J. E.; Marvel, C. S. J. Org. Chem. 1961, 26, 95–97. Chem. Abstr. 1961, 55, 1902. DOI: 10.1021/jo01060a022.
- Clarke, H. T.; Gurin, S. J. Am. Chem. Soc. 1935, 57, 1876–1881. DOI: 10.1021/ja01313a035.
- Todd, A. R.; Bergel, F. Berichte 1936, 69B, 217–223. Chem. Abstr. 1936, 30, 3431.
- Karimullah, J. Chem. Soc. 1937, 1937, 961–962. Chem. Abstr. 1937, 31, 6231.
- Kiprianov, A. I.; Asnina, F. I.; Shako, I. K. J. Gen. Chem. U.S.S.R. 1948, 18, 165. Chem. Abstr. 1948, 42, 7293.
- Sych, E. D.; Mikitenko, E. K. Khim. Geterotsikl. Soedinenii. 1971, 7, 857. Chem. Abstr. 1972, 76, 25154k.
- Sarkis, G. Y.; Al-Azawe, S. J. Chem. Eng. Data 1973, 18, 99–102. Chem. Abstr. 1973, 78, 84310. DOI: 10.1021/je60056a029.
- Bonzom, A.; Metzger, J. Bull. Soc. Chim. France 1963, 11, 2582. Chem. Abstr. 1964, 60, 5474.
- Young, T. E.; Amstutz, E. D. J. Am. Chem. Soc. 1951, 73, 4773–4775. DOI: 10.1021/ja01154a088.
- Emanuel, W.; Stamford, conn., assignor to American Cyanamid Company, New York, N.Y. A Corporation of Maine, No Drawing. Application July 2, 1949, Serial No. 102, 926, U.S. Patent No. 1951, 2 547 677; Chem. Abstr. 1951, 45, 6351.
- Marchesini, G. Gazz. Chim. Ital. 1893, 23, 437.
- Tcherniac, J. Berichte. 1892, 25, 2067.
- Erlenmeyer, H.; Epprecht, A.; von Meyenburg, H. Hca. 1937, 20, 514–515. Chem. Abstr. 1937, 31, 6230. DOI: 10.1002/hlca.19370200177.
- Andersag, H.; Westphal, K. Wuppertal-Elberfeld, Germany, assignors to Winthrop Chemical Company, Inc., New York, N. Y., a corporation of New York, No Drawing. Application April 22, 1936, Serial No. 75865. In Germany May 2, 1935 U. S. Patent No. 2 139 570; Chem. Abstr. 1939, 33, 7819.
- Ganapathi, K.; Venkataraman, A. Proc. Indian Acad. Sci. 1945, 22, 343–358. Chem. Abstr. 1946, 40, 4056. DOI: 10.1007/BF03052641.
- Gibbs, E. M.; Robinson, F. A. J. Chem. Soc. 1945, 1945, 925. Chem. Abstr. 1946, 40, 1830. DOI: 10.1039/jr9450000925.
- Watt, G. W. J. Org. Chem. 1939, 4, 436–441. DOI: 10.1021/jo01216a010.
- Takahashi, T.; Nishigaki, S. J. Pharma. Soc. Japan 1953, 73, 1071–1075. Chem. Abstr. 1954, 48, 12081. DOI: 10.1248/yakushi1947.73.10_1071.
- Takahashi, T.; Nishigaki, S.; Sakamoto, S. J. Pharma. Soc. Jpn. 1953, 73, 1076–1078. Chem. Abstr. 1954, 48, 12082. DOI: 10.1248/yakushi1947.73.10_1076.
- Cook, A. H.; Heilbron, I. M.; Levy, A. L. J. Chem. Soc. 1947, 1, 1598–1609. DOI: 10.1039/JR9470001598.
- Cook, A. H.; Heilbron, I. M.; Stern, E. J. Chem. Soc. 1948, 1948, 2031. Chem. Abstr. 1949, 43, 3409. DOI: 10.1039/jr9480002031.
- Hoggarth, E. J. Chem. Soc. 1947, 1947, 431. Chem. Abstr. 1947, 41, 3458.
- Gabriel, S. Berichte 1910, 43, 1283.
- Asinger, F.; Thiel, M.; Pallas, E. Justus Liebigs Ann. Chem. 1957, 602, 37–49. Chem. Abstr. 1957, 51, 12074. DOI: 10.1002/jlac.19576020104.
- Davies, W.; MacLaren, J.; Wilkinson, L. J. J. Chem. Soc. 1950, 1950, 3491. Chem. Abstr. 1951, 45, 7110. DOI: 10.1039/jr9500003491.
- Chase, B.; Walker, J. J. Chem. Soc 1955, 1955, 4443. Chem. Abstr. 1956, 50, 10712.
- Ravizza, S. A. Netherlands Appl. 1966, 508, 138. Chem. Abstr. 1966, 64, 17611A.
- Koichi, N.; Shen, S. S.; Mao-Yi, L. Tetrahedron Lett. 2009, 50, 3161.
- Dominique, R.; Qiao, Q.; Goodnow, R. Jr. Tetrahedron Lett 2008, 49, 3682.
- Hassan, F.; Saeed, Z.; Ahmad, S. Jnus. 2011, 14, 1–6. DOI: 10.22401/JNUS.14.1.01.
- Frija, L.; Pombeiro, A.; Kopylovich, M. Coord. Chem. Rev. 2016, 308, 32–55. DOI: 10.1016/j.ccr.2015.10.003.
- Zaharia, V.; Ignat, A.; Palibroda, N.; Ngameni, B.; Kuete, V.; Fokunang, C.; Moungang, M.; Ngadjui, B. Eur. J. Med. Chem. 2010, 45, 5080–5085. DOI: 10.1016/j.ejmech.2010.08.017.
- Bueno, J.; Carda, M. M.; Crespo, B.; Cunat, A. C.; de Cozar, C.; Leon, M. L.; Marco, J. A.; Roda, N.; Sanz-Cervera, J. F. Bioorg. Med. Chem. Lett. 2016, 26, 3938–3944. DOI: 10.1016/j.bmcl.2016.07.010.
- de Oliveira Filho, G. B.; Cardoso, M. V. d O.; Espíndola, J. W. P.; Oliveira E Silva, D. A.; Ferreira, R. S.; Coelho, P. L.; Anjos, P. S. D.; Santos, E. d S.; Meira, C. S.; Moreira, D. R. M.; et al. Eur. J. Med. Chem. 2017, 141, 346–361.,DOI: 10.1016/j.ejmech.2017.09.047.
- Arafa, W.; Badry, M. J. Chem. Res. 2016, 40, 385–392. DOI: 10.3184/174751916X14639296902648.
- Łączkowski, K. Z.; Konklewska, N.; Biernasiuk, A.; Malm, A.; Sałat, K.; Furgała, A.; Dzitko, K.; Bekier, A.; Baranowska-Łączkowska, A.; Paneth, A. Med. Chem. Res. 2018, 27, 2125–2140. DOI: 10.1007/s00044-018-2221-x.
- Berseneva, V. S.; Tkachev, A. V.; Morzherin, Y. Y.; Dehaen, W.; Luyten, I.; Toppet, S.; Bakulev, V. A. J. Chem. Soc, Perkin Trans. 1. 1998, 1998, 2133–2136. DOI: 10.1039/a803543a.
- Darehkordi, A.; Saidi, K.; Islami, M. R. Arkivoc. 2007, 2007, 180–188. DOI: 10.3998/ark.5550190.0008.120.
- McKillop, A.; Bellinger, G. C. A.; Preston, P. N.; Davidson, A.; King, T. J. Tetrahedron Lett. 1978, 19, 2621–2624. DOI: 10.1016/S0040-4039(01)94845-2.
- Acheson, R. M.; Wallis, J. D. J. Chem. Soc, Perkin Trans. 1. 1981, 1, 415–422. DOI: 10.1039/p19810000415.
- Turan-Zitouni, G.; Altıntop, M. D.; Ozdemir, A.; Kaplancikli, Z. A.; Ciftci, G. A.; Temel, H. E. Eur. J. Med. Chem. 2016, 107, 288–294. DOI: 10.1016/j.ejmech.2015.11.002.
- Abdel‐Latif, E.; Bondock, S. Heteroat. Chem. 2006, 17, 299–305. DOI: 10.1002/hc.20206.
- Kim, H.; Cho, S.; Yoo, M.; Kang, S.; Kim, K.; Lee D, H.; Song, J.; Rhee, S.; Jung, W.; Ahn, J.; et al. Bioorg. Med. Chem. Lett. 2017, 27, 5213–5220. DOI: 10.1016/j.bmcl.2017.10.046.
- de Santana, T.; Barbosa, M.; Gomes, P.; da Cruz, A.; da Silva, T.; Leite, A. Eur. J. Med. Chem. 2018, 144, 874–886. DOI: 10.1016/j.ejmech.2017.12.040.
- Asif, M.; Ali, A.; Zafar, A.; Farhan, M.; Khanam, H.; Hadi, S. M.; Shamsuzzaman , J. Photochem. Photobiol. B Biol. 2017, 166, 104–115. DOI: 10.1016/j.jphotobiol.2016.11.010.
- Dessl, A.; Consiglio, G.; Calamante, M.; Reginato, G.; Mordini, A.; Peruzzini, M.; Taddei, M.; Sinicropi, A.; Parisi, M.; de Biani, F.; et al. Eur. J. Org. Chem. 2013, 2013, 1916–1928. DOI: 10.1002/ejoc.201201629.
- Abu-Zaied, M.; Elgemeie, G. Heteroat. Chem. 2017, 28, e21404. DOI: 10.1002/hc.21404.
- Farag, A.; Kheder, N.; Dawood, K. Amer. J. Org. Chem. 2015, 5, 73–77. DOI: 10.5923/j.ajoc.20150502.02.
- Sayed, A. R. Turk. J. Chem. 2015, 39, 600–609. DOI: 10.3906/kim-1412-77.
- Gomha, S. M.; Farghaly, T. A.; Sayed, A. R. J. Heterocycl. Chem. 2017, 54, 1537–1542. DOI: 10.1002/jhet.2741.
- Abdelhamid, A. O.; Sayed, A. R. Phosph. Sulf. Silicon Rel. Elem. 2007, 182, 1767–1777. DOI: 10.1080/10426500701313938.
- Gomha, S. M.; Farghaly, T. A.; Sayed, A. R. J. Heterocycl. Chem. 2016, 53, 1503–1509.
- Farghaly, T. A.; Abdallah, M. A.; Khedr, M. A.; Mahmoud, H. K. J. Heterocycl. Chem. 2017, 54, 2417–2425. DOI: 10.1002/jhet.2838.
- El-Hag, F.; Gomha, S.; Farghaly, T. J. Heterocycl. Chem. 2017, 54, 1172–1177. DOI: 10.1002/jhet.2688.
- Sayed, A. R.; Gomha, S. M.; Farghaly, T. A. J. Heterocycl. Chem. 2016, 53, 255–262. DOI: 10.1002/jhet.2320.
- Rouf, A.; Tanyeli, C. Eur. J. Med. Chem. 2015, 97, 911–927. DOI: 10.1016/j.ejmech.2014.10.058.
- Jin, Z. Nat. Prod. Rep. 2011, 28, 1143–1191. DOI: 10.1039/c0np00074d.
- Arora, P.; Narang, R.; Nayak, S.; Singh, S.; Judge, V. Med. Chem. Res. 2016, 25, 1717–1743. DOI: 10.1007/s00044-016-1610-2.
- Shawali, A. S. J. Adv. Res. 2016, 7, 873–907. DOI: 10.1016/j.jare.2016.09.001.
- Havrylyuk, D.; Mosula, L.; Zimenkovsky, B.; Vasylenko, O.; Gzella, A.; Lesyk, R. Eur. J. Med. Chem. 2010, 45, 5012–5021. DOI: 10.1016/j.ejmech.2010.08.008.
- Liu, Z. Y.; Wang, Y. M.; Li, Z. R.; Jiang, J. D.; Boykin, D. Bioorg. Med. Chem. Lett. 2009, 19, 5661–5664. DOI: 10.1016/j.bmcl.2009.08.025.
- Luzina, E.; Popov, A. Eur. J. Med. Chem. 2009, 44, 4944–4953. DOI: 10.1016/j.ejmech.2009.08.007.
- Kouatly, O.; Geronikaki, A.; Kamoutsis, C.; Hadjipavlou-Litina, D.; Eleftheriou, P. Eur. J. Med. Chem. 2009, 44, 1198–1204. DOI: 10.1016/j.ejmech.2008.05.029.
- Mohareb, R.; Zaki, M.; Abbas, N. Steroids 2015, 98, 80–91. DOI: 10.1016/j.steroids.2015.03.001.
- Mohareb, R.; Al-Omran, F.; Abdelaziz, M.; Ibrahim, R. Acta Chim. Slov. 2017, 64, 349–364. DOI: 10.17344/acsi.2017.3200.
- Ahangar, N.; Ayati, A.; Alipour, E.; Pashapour, A.; Foroumadi, A.; Emami, S. Chem. Biol. Drug Des. 2011, 78, 844–852. DOI: 10.1111/j.1747-0285.2011.01211.x.
- Azam, F.; Alkskas, I.; Lal Khokra, S.; Prakash, O. Eur. J. Med. Chem. 2009, 44, 203–211. DOI: 10.1016/j.ejmech.2008.02.007.
- Siddiqui, N.; Ahsan, W. Eur. J. Med. Chem. 2010, 45, 1536–1543. DOI: 10.1016/j.ejmech.2009.12.062.
- Marchesini, G. Gazz. Chim. Ital. 1894, 24, 65.
- Heilbron, I. J. Chem. Soc. 1949, 1949, 2099. DOI: 10.1039/jr9490002099.
- Rashdan, H. R. M.; Abdelmonse, A. H.; Shehadi, I. A.; Gomha, S. M.; Soliman, A. M.; Mahmoud, H. K. Molecules 2020, 25, 4997. DOI: 10.3390/molecules25214997.
- Sayed, A. R.; Gomha, S. M.; Taher, E. A.; Muhammad, Z. A.; El-Seedi, H. R.; Gaber, H. M.; Ahmed, M. M. Drug Des. Devel. Ther. 2020, 14, 1363–1375. DOI: 10.2147/DDDT.S221263.
- Abu-Melha, S.; Edrees, M. M.; Riyadh, S. M.; Abdelaziz, M. R.; Elfiky, A. A.; Gomha, S. M. Molecules 2020, 25, 4565. doi.org/ DOI: 10.3390/molecules25194565.
- Mahmoud, H. K.; Abbas, A. A.; Gomha, S. M. Polycyclic Aromat. Compd. 2020, DOI: 10.1080/10406638.2019.1709085..
- Gomha, S. M.; Abdelhamid, A. O.; Abdelrehem, N. A.; Kandeel, S. M. J. Heterocycl. Chem. 2018, 55, 995–1001. DOI: 10.1002/jhet.3131.
- Ouf, S. A.; Gomha, S. M.; Eweis, M.; Ouf, A. S.; Sharawy, I. A. Bioorg. Med. Chem. 2018, 26, 3287–3295. DOI: 10.1016/j.bmc.2018.04.056.
- Ouf, S. A.; Gomha, S. M.; Ewies, M. M.; Sharawy, I. A. A. J. Heterocycl. Chem. 2018, 55, 258–264. DOI: 10.1002/jhet.3040.
- Gomha, S. M.; Muhammad, Z. A.; Gaber, H. M.; Amin, M. M. J. Heterocycl. Chem. 2017, 54, 2708–2716. DOI: 10.1002/jhet.2872.
- Sayed, A. R.; Ali, S. H.; Gomha, S. M.; Al-Faiyz, Y. S. Synth. Commun. 2020, 50, 3175–3203. DOI: 10.1080/00397911.2020.1799016.
- Sayed, A. R.; Al-Faiyz, Y. S. Heterocycles 2020, 100, 955–963. DOI: 10.3987/COM-20-14254.
- Sayed, A. R.; Ali, S. H.; Al-Faiyz, Y. S. Synth. Commun. 2019, 49, 3210–3217. DOI: 10.1080/00397911.2019.1661482.