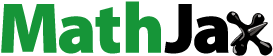
Abstract
This study investigates the long-term performance of a 52-kW on-grid solar PV plant in the Mechanical ‘C’ block, SRM Institute of Science and Technology (SRMIST). This article delivers a simple approach that would act as a pivot for PV system assessment. Therefore, methodologies like Energy yield analysis, Energy distribution, and Life cycle costing are implemented. This empowers the methods to facilitate pre-auditing, energy conservation, and economic analysis. The performance ratio and a capacity factor of the 52-kW PV plant in 2020 are determined as 60% and 12.8%, respectively. The study offers that the plant has a less simple payback period and energy pack time for 2020. From this study, the issues identified in the plant are highlighted with the solution. It also paves the way for the researchers to suggest the solutions for the underutilisation of the plant, especially in the situations like fault occurrence, pandemic conditions, etc.
KEYWORDS:
Nomenclature
YR | = | Reference yield (h/day) |
YA | = | Array yield (h/day) |
YF | = | Final yield (h/day) |
LAC | = | Array capture loss |
LS | = | System loss |
ηAL | = | Array efficiency (%) |
ηSYS | = | System efficiency (%) |
ηINV | = | Inverter efficiency (%) |
EP | = | Energy essential to produce the material essential for PV system (kWh/m2) |
EM | = | Energy essential to manufacture PV system (kWh/m2) |
ET | = | Energy essential to transport the materials vital during the lifespan (kWh/m2) |
EI | = | Energy essential to install the system (kWh/m2) |
ELM | = | Energy requirement for end-of-life management(kWh/m2) |
ESI | = | Annual solar radiation (kWh/m2) |
ETE | = | Total embodied energy (kWh/m2) |
LPV | = | Life of the PV system (years) |
η:LCC | = | Life cycle conversion efficiency (%) |
i | = | Inflation rate (%) |
d | = | Discount rate (%) |
DHI | = | Diffuse Horizontal Irradiation |
Abbreviations
PV | = | Photovoltaic |
MNRE | = | Ministry of new and renewable energy |
UP | = | Uttar Pradesh |
NREL | = | National Renewable Energy Laboratory |
SRMIST | = | SRM Institute of Science and Technology |
DC | = | Direct current |
AC | = | Alternating current |
STC | = | Standard testing condition |
IEC | = | International electrotechnical commission |
PR | = | Performance ratio |
CF | = | Capacity factor |
GHI | = | Global horizontal irradiation |
EPT | = | Energy payback time |
EPF | = | Electricity production factor |
MPP | = | Maximum power point |
LED | = | Light emitting diode |
LC | = | Lifecycle |
O & M | = | Operation and maintenance cost |
PWF | = | Present worth factor |
ALC | = | Annual lifecycle |
= | Present worth factor of future recurring investment |
1. Introduction
Recently, renewable energy sources have been a solution or alternative to the energy deficit in the countries due to the depletion of fossil fuels and the growth of population and technologies. Among the renewable energy sources, solar is the most promising and fast-growing technology due to the adverse research in this area. It does not create any destructive effect on the environment. In our country, India, a separate ministry, the Ministry of new and renewable energy (MNRE), is formed to concentrate on deploying non-conventional energy sources throughout the country, especially in the highly promising area. MNRE insisted many educational institutions install solar PV plants on the rooftop of the building for their use. This will benefit the institution with the energy yield and motivate the students to study the components of stand-alone and grid-connected PV systems. Currently, many institutes like the Indian Institute of Technology (IIT), Roorkee (1.8 MW), IIT, Kanpur (1.8 MW), IIT Bombay (1 MW), etc. are slowly deploying solar PV plants in the institute for harnessing solar energy for their utilisation.
Many researchers are concentrating on making a case study on the installed PV stations, which will help us to identify the problems raised in the plant. The inference from the analysis made on the performance parameters will also guide us in identifying the solutions to the real-time issues, which will enhance the performance of the PV system. Recently, the case studies on PV park in Sweden (Lindberg et al. Citation2021), construction on PV system in Turkey (Colak, Memisoglu, and Gercek Citation2019), PV facilities in South Korea (Jung et al. Citation2020), stand-alone PV system in Egypt for irrigation purpose (Rezk, Abdelkareem, and Ghenai Citation2019), grid-connected PV system in Iraq (Aziz et al. Citation2020), impact of optimal tilt angle and solar radiation in PV system in Beijing (Shen et al. Citation2018) etc. are carried out to highlight the significant inferences from the study. From these studies and the results, we get the information related to:
Factors to be considered for selecting PV sites and constructing PV plants using a geographical information system (Colak, Memisoglu, and Gercek Citation2019).
Utility-scale solar guide for establishing solar park (Lindberg et al. Citation2021).
Construction of PV system considering the variation in atmospheric conditions (Shen et al. Citation2018).
Modern algorithms can forecast long-term power facilities from PV systems (Jung et al. Citation2020).
Various software like Homer, hybrid pro, PVsyst, etc. is used to design the PV system before installation (Kumar et al. Citation2020).
In India, a detailed study on grid-tie rooftop solar PV for domestic application in Ujjain city is carried out using solar simulation software like SolarGIS, PVGIS, PVSOL SISIFO, etc. (Dondariya et al. Citation2018). The most highly populated state in India is Uttar Pradesh (UP), in which numerous houses lack access to electricity. To find a solution to this issue, the UP-state government issued a new mini-grid policy which attracted great attention. This UP-policy and solar PV-based mini-grid is explained by exploring a 1 MW grid-connected PV system (Bhattacharyya et al. Citation2019). The central government has announced several plans for promoting solar PV technologies. Exclusively, the reverse auction process is introduced, and policies are framed to bring down the rate of energy generated from PV Nisarg Shah (Citation2020). Hence, from these articles, we observe that developing countries like India still need a lot of studies to increase the feasibility and viability of performance-enhanced solar PV systems in the countryside and remote locations.
Similarly, in this paper, we took a grid-tied rooftop PV system in an academic institute in India to study its performance. The performance parameters of the plant are studied for one assessment period (Malvoni et al. Citation2017; Shiva Kumar and Sudhakar Citation2015; de Lima, Ferreira, and de Lima Morais Citation2017).
The main contribution of this work is: (1) a step-by-step procedure is proposed for analyzing the performance of the plant from real-time data, (2) significant results from the study are highlighted with the inference from the study and (3) notable issues from the commissioned power plant are identified, and the relevant solutions are proposed for the issues. The methodology chosen for the study is explained in the third section. In the subsequent sections, the 52-kW PV plant is explained with the derivation of all the performance parameters of the system. Finally, a comparative analysis is made to highlight the drawback of the plant’s performance in the year 2020.
2. Explanation of installed 52-kW PV system
A 52 kW grid-tie PV system consists of 160 polycrystalline silicon PV panels, each 325 kW LE24P325 with a single-cell size of 156.75 × 156.75 mm and a total weight of 21.5 kg. It has 72 cells with tempered glass and an anti-reflection coating. The thickness of the glass is 3.2 mm with the anodised aluminium alloy frame.
The solar PV module is arranged in 10 parallel strings, and each string has 16 PV modules to produce a 325 × 160 = 52-kW rating. These 160 numbers of PV modules are connected via an inbuilt DC disconnect switch to Delta RPI-M50A grid-tie inverter, directly fed into the low tension (LT) line. The input and output to the inverter are displayed in the screen in the front panel of the inverter. It gives the data such as DC and AC voltage and current, daily and monthly energy and power generated, and other required data. This grid-tie inverter is a central inverter where all the 10 strings are connected to the central inverter.
This PV plant was integrated on 30 August 2019 but commissioned on 30 September 2019, and the schematic diagram of the installed 52-kW PV system is depicted in (a–c). Tables and present the technical specifications of the solar PV module and Delta RPI-M50A grid-tie inverter, respectively.
Figure 1. (a) Illustrative diagram of 52-kW solar PV system, (b) picture of the 325 W PV array and (c) mechanical ‘C’ block where 52 kW PV plant installed.

Table 1. Description of 325 W PV module.
Table 2. Specification of Delta RPI-M50A Grid-tie inverter.
3. Methodology for this study
In this section, the methodology incorporated to study the performance of the 52-kW plant is presented. As a preliminary analysis, metrological data of the proposed site is obtained from NREL and observed the solar irradiance potential in the study area.
Step 1: The load details of the block where the 52-kW PV plant is installed on the rooftop are explained elaborately with lighting to high power loads. The study on the load details of the building will give us an overview of the usage of the energy in that building with the scope to design an alternative solution to replace the zero-fossil fuel consumption in that building.
Step 2: All data required for the study are obtained from the online monitoring system Delremo e-monitoring service. The complete study is performed from the data retrieved, and the system yield and losses analysis is performed.
Step 3: Principal cost of the installed 52-kW PV plant is obtained from the maintenance department of the institute, and the life cycle cost analysis is performed.
Step 4: Notable findings from the study are highlighted with the solution to the issues raised. The future scope for further investigation is observed, and the scope for the further enhancement of the 52-kW PV plant is finally listed in the article.
3.1. Study area
The study area is Potheri, Kattankulathur, Tamil Nadu 603203, India with a latitude of 12.4912° and a longitude of 80.02245°. Solar PV plant (52 kW) is installed on the rooftop of Mechanical ‘C’ block, Main campus, SRMIST, Kattankulathur, Chennai, India. (a) represents the satellite map of Mechanical ‘C’ block location where the 52-kW solar panel installed. The sun path of the location obtained from SunCalc is also presented in (b). Delremo, Delta Electronics online monitoring system is used to track the data and its dashboard is depicted in Figure (c).
3.2. Meteorological data of installed location
To begin this study, the solar radiation data with temperature and wind velocity of the area where 52-kW PV plant installed are obtained from PVsyst. This study is required in the preliminary stage for the installation of the PV system. Furthermore, the data are also essential for determining the healthiness of the installed PV system.
(a–d) illustrates the solar radiation data obtained for Kattankulathur, Tamil Nadu, India. From this data, it is noted that the average GHI for the year is 5.82 kWh/m2/day. Similarly, the average DHI for the year is 2.3 kWh/m2/day. The maximum temperature and wind velocity in a year are 31.5°C and 3.15 m/s. These data are obtained from the NREL through PVsyst 7.1software.
3.3. Load details in the proposed site
A comprehensive investigation of each room of Mechanical ‘C’ Block was done to specify the load details and the required solar PV system to make it a zero building. The total area of the building is 23,002.94 square feet. Tables afford the details of the number of rooms in the building with the loads.
Table 3. Number of rooms and loads in Mechanical ‘C’ block.
Table 4. AC load details in mechanical ‘C’ block.
Table 5. Load details with total wattage in mechanical ‘C’ block.
4. Energy yield analysis of 52-kW PV system
The healthiness of the solar PV system can be determined by calculating the PV functional constraints like YR, YA, YF and PR. represents the flow diagram which illustrates the parameters required to evaluate the function of the PV system according to IEC 61724. The functioning of the PV plant is commonly studied by analysing the system, which constitutes YR, YA and YF.
The YA is obtained by dividing the Direct Current energy output by rated PV power over the specified period of time. YR is determined by calculating the ratio of global solar radiation and reference PV radiation at STC. YF is obtained by determining the total energy yield obtained in proportion to deliver to the customer and the rated mounted PV system. PR of the mounted system is achieved by dividing YF and YR. ().
Table 6. Significant expression for performance analysis.
The performance of the installed PV system is depicted with the results obtained from the online monitoring system. (a–c) present the energy yield from the inverter. It is monitored for the entire 2020 and presented in (a). To present the energy yield for a month, everyday energy yield is obtained for the month June and November, and it is presented in (b,c), respectively. The hourly variation of the AC power output is also offered for 20 June 2020. This hourly monitoring of power output is done from 5 AM to 8 PM. From this observation, we noted that the peak power is obtained at 1:30 PM. AC, DC voltage and current for various days in a year are observed and illustrated in Figures and . (a–d) depicts the AC voltage and current on 30th May, 24th July, 27th October, 1st December, respectively. Likewise, DC voltage and current are presented for the same days in (a–d).
Figure 5. Energy yield and AC power output from inverter. (a) Energy yield in the year 2020 (month-wise), (b) energy yield in the month of June (day-wise), (c) energy yield in the month of November (day-wise) and (d) AC power output on 20 June 2020 (hourly-basis).

Figure 6. AC Voltage and current (Line 1) for various days in the year. (a) 30 May 2020, (b) 24 July 2020, (c) 27 October 2020 and (d) 1 December 2020.

Figure 7. DC voltage and current for various days in the year. (a) 30 May 2020, (b) 24 July 2020, (c) 27 October 2020 and (d) 1 December 2020.

presents the analysis performed on the system losses and efficiency of the plant for one month. It is noted that the energy harnessed from the plant is more during the month of February, March and June. Hence the analysis is presented for the month of June and presented in for further study. presents the performance parameters of 52-kW solar PV plant. The assessment is carried out for one year, 2020. Performance ratio (PR) and capacity factor (CF) are obtained in percentage for every month of the year 2020 and their average values are obtained and observed. The performance ratio mainly depends on
How the PV system is monitored regularly.
How fast the defects are detected.
Temperature of the PV module. Generally, the PR value recommended for roof-top PV system is 75%.
Table 7. System yield and loss analysis of 52-kW PV system.
Table 8. Functional parameters of 52 kW PV system for the assessment period (2020).
It is noted from , the performance of the plant droops for a certain period even though with the good solar radiation in the installed area.
5. Life cycle investigation of the plant
In this section, the life cycle analysis of 52-kW solar PV plant for 30 yrs is calculated and discussed.
5.1. Calculation of energy payback time (EPT)
To calculate the operating time required to recover the energy spent in making the PV system, the following expression is used.
(1)
(1)
Tiwari et al. (Citation2009) proposed the values of above-mentioned energy demand for the square metre area of the PV module. The assumptions considered in Tiwari et al. (Citation2009) is taken as such for the mathematical analysis of these energy metrics. In Phylipsen and Alsema (Citation1995); Wong, Royapoor, and Chan (Citation2016), the embodied energy considered for polycrystalline solar module is 1145 kWh/m2. depicts the values of various parameters accounted for the calculation of total embodied energy per m2. The value is taken as
(2)
(2)
Figure 8. Split-up for embodied energy for a solar PV system (Tiwari et al. Citation2009).

Total area of the 52-kW PV module is
(3)
(3)
(4)
(4)
Total encompassed energy from Equation (2)
(5)
(5)
(6)
(6)
Considering Equations (5) and (6) EPT are calculated as
(7)
(7)
The comprehensive performance of the system is considered by taking the reciprocal of EPT. Therefore, EPF is given by,
(8)
(8)
(9)
(9)
Yearly capacity utilisation factor is calculated by considering the yearly energy yield of the installed 52-kW PV plant from Equation (6), and it is given by
(10)
(10)
5.2. Calculation of life cycle conversion efficiency
This terminology is calculated for the lifespan of the solar PV plant. It gives the overall productivity of the PV plant in consideration of solar irradiation.
(11)
(11)
The lifespan of PV plant is taken as 30 yrs and the entire solar irradiation in one year is 1927 kWh/m2. The life cycle conversion efficiency is
The noted lifespan of the PV module is 30 yrs, however, the mounting structure has the same lifetime with 10% replacement. The other components (OC) of the plant require replacements which are discussed in Section 5.3.2.
5.3. Cost evaluation of the PV plant
Payback period is the period during which the invested money can be recovered. This can be estimated in two ways
Simple payback period
Lifecycle costing (LCC)
presents the capital cost and other lifespan cost of the 52-kW PV plant. It is observed that the estimation of LCC is quite cumbersome compared to a simple payback period where the system cost and annual electricity generated are considered for estimation.
Table 9. Cost distribution of 52-kW PV system.
5.3.1. Simple payback period
Simple payback just gives the time to get back the invested amount through solar energy saving. Depreciation, inflation rate, O & M cost and other lifetime cost of the PV system is not included.
(13)
(13)
The entire yearly energy yield in the year 2020 is 57.75 MWh. According to Tamil Nadu electricity regulatory commission (TNERC), the tariff (kWh) for the electricity consumed in private academic institutes and hostel is Rs.7.50/-.
(14)
(14)
Simple payback period of 52-kW PV plant is 5.6 years. This value gives the time required to raise the fund expended for the PV plant.
5.3.2. Lifecycle (LC) cost
LC cost gives the cost of the PV plant in its lifetime. It includes system cost, O & M cost and replacement cost. Inflation rate in India is 5.1% (FY-2020). The discount rate is considered as 10%. The lifespan of the PV plant is taken as 30 yrs.
PWF of the investment is obtained by
(15)
(15)
where i = inflation rate, d = discount rate.
The product cost decreases in the future since we assume that the discount rate greater than the inflation rate. The product cost decreases with the factor, PWF. The present worth of the components that need replacement after certain of period of time is obtained by estimating the value of PWF. To include the O & M cost of the PV plant for 30 yrs of lifespan, it is essential to calculate the present worth of future recurring investment. It is determined as
(16)
(16)
The value of f for d = 0.1 is 0.955. The value of f generally varies from 0.95 to 1.05. Annualised lifecycle (ALC) cost of the PV system is estimated to determine the annual cost of the operation of the system in terms of the current value of money. ALC cost is calculated as
(17)
(17)
shows the details of LC cost analysis of the PV system with PWF value for the required components to be replaced in the lifetime. It also depicts the ALC cost of the 52-kW PV plant.
Table 10. LC cost of 52-kW PV plant with 30 yrs lifespan.
6. Results and discussion
The photovoltaic system studied is installed in the Mechanical ‘C’ block of SRMIST, Kattankulathur, Tamil Nadu, India. The 52-kW plant is installed with 160 modules of 325 W each. It covers a total area of 310.46 square metres. The analysis is carried out for one assessment period (Jan-Dec 2020) to study the performance parameters of the 52-kW solar PV plant. To perform this study, meteorological data of the proposed site are obtained from PVsyst 7.1 software through NREL solar resource data. The global horizontal solar irradiance is received daily and monthly and presented to study the performance ratio.
The energy yield calculation of the plant with the performance ratio is presented in . From this, it is observed that the performance ratio varies from 85% in October to 61% in January. Similarly, the capacity factor is calculated for every month during the assessment period, and it is noted that it is high for March (19%) and less for December (12%). The average capacity factor for the year 2020 is calculated. It is 12.8%, representing that the installed PV system can produce full energy for only 47 days in a year.The global horizontal irradiation (GHI) is varied from197.6 kWh/m2/month in March to 118 kWh/m2/month inDecember. The average GHI and temperature of the site are about 161 kWh/m2 and 28.5°C, respectively. The average temperature per month varies between 31.5°C in May and 25.3°C in January. According to the highest GHI, the energy yield is also high in March, amounting to 6928 kWh, and it is less in December, amounting to 4545 kWh. The main factors that influence power generation in PV systems are soiling, shading, temperature, module orientation, parasitic resistance, fill factor, cable thickness, etc.
represents the total energy yield from the 52 kW PV plant from the beginning. The total income generated is obtained by taking into account the tariff rate of Rs. 7.50/- per unit (kWh). Likewise, the CO2 avoided is obtained by multiplying the energy yield by 0.8 Kg per kWh.
Table 11. Paybacks of installed 52-kW PV plant.
6.1. Notable findings from the study
This section highlights inferences from the study with the issues and solutions to the problems noticed. Energy yield in April and May is observed to be very low. This is due to the plant’s shutdown on holidays and during pandemic situations, i.e. covid-19. The most significant inference is that the 52-kW plant will be under-utilised in 2020. The plant is commissioned in September 2019. To study the underutilisation of the PV plant, we observed the daily energy yield from April 2020 to November 2020, tabulated in .
Table 12. Day-wise energy yield for the month (April-November 2020).
From , it is noted that the PR of the plant is reduced in April, May, and September 2020. The reason for reducing this performance ratio is tabulated in . This table elaborates on the inferences from the study and the solutions that need to be incorporated. Furthermore, the reference articles for implementing the solutions are also presented in . In India, we noticed that country observes around 15–20 holidays in a year. During these days, the 52-kW on-grid PV plant is shut down due to the low load in the institute. However, in the case of the weekend, the institute functions with part-time classes. Hence, it is required to get approval from the TNERC for the grid interaction with high tension line and to install the net metering equipment. This will eradicate the issue noted during the public holidays and the peculiar pandemic situation.
Table 13. Inferences from the study.
A comparative study is performed to highlight the actual performance of the PV plant. Figures and present the survey carried out with the calculated energy yield for the months where the generated PV power was not utilised correctly. (a,b) present the system yield and performance parameters of the PV plant under normal operating conditions. From this study, the performance ratio and a capacity factor of the 52-kW plant are high compared to the performance parameters noted in 2020. depicts the comparative research on other performance parameters like EPT, EPF, LCCE, average PR, average CF, and simple payback period. These analyses are carried out by considering the actual energy yield of the plant, as depicted in . The exact simple payback period of the plant is 4.5 years, whereas it gives 5.6 years with the energy yield obtained from the year 2020.
Figure 9. (a) System yield of the plant based on the actual performance and (b) actual PR and CF of the plant.

Figure 10. (a), (b) Comparative study on Performance parameters of 52-kW PV plant based on actual and year 2020 performance and (c) Comparison on simple payback period of PV plant.

Table 14. Actual energy yield (kWh) of 52-kW plant without any issue.
6.2. Comparison with other plants
The performance metrics of existing photovoltaic systems were compared to those of other solar plants using the most widely used factors, including capacity, CUF, and PR, in order to reinforce the study’s conclusions. summarises the important performance metrics for which the current plant is better than those in other regions. lists the key performance characteristics for which the 52-kW solar Photovoltaic plant’s results were nominal in range when compared to those in other regions. It’s significant to mention that all of the studies focused at a one-year observation period (Dahmoun et al. Citation2021). Several solar hotspots in India were identified, and their performance ratio is plotted and discussed (Saxena, Saxena, and Sudhakar Citation2021).
Table 15. Comparative study of 52-kW plant with other PV plants in literature.
6.3. Future scope
In this study, we also noted that, there is scope to extend the work in the 52-kW PV plant for further research work. The inferences from this study will motivate the under and postgraduate students in SRMIST to take the problem statements for their study and bring an alternative solution to the issues identified. Suggestion for extending the research work for the future in this area is presented in .
Table 16. Suggestions for performance improvement in 52-kW PV plant.
7. Conclusion and policy implications
In this study, an investigation and assessment of a 52-kW ON-grid solar PV plant mounted in the Mechanical ‘C’ block of SRMIST are carried out. This plant is monitored and analysed for one-year 2020, and the following observations are noted from this study.
The energy yielded from the commencement date to December 2020 is 39.7 MWh.
The total energy yielded from the plant for 2020 is 57.75 MWh.
In the assessment period, the highest energy yield was 6928 kWh in March 2020.
The average PR of the PV plant is 60%.
The average CF is 12.8% which illustrates the full energy output from the 52-kW plant for 47 days in the year 2020.
The energy payback time and electricity production factor of the system is 8.1 years and 0.122, respectively.
The annual capacity utilisation factor is 12.7%
The lifecycle conversion efficiency of the system by taking 30 years as the life of the PV plant is 7%.
The LCC and ALCC of the PV system are Rs.3,909,593/- and Rs.2,44,472/-, respectively. The energy generated from the system is fed to the low-tension line, and it is not appropriately monitored.
Delta Electronics established an online monitoring system limited to tracking the installed plant’s energy yield. However, the energy fed into the grid is not sensed and monitored regularly.
The amount of CO2 avoided due to the installation of this PV plant is 31.73 Ton.
The amount of diesel saved by using this PV system is 3.69 kL.
The revenue generated from this 52-kW PV plant from the date of commencement is three lakhs.
The observation made from the study of the 52-kW solar PV plant is the PV system is not utilised entirely in the year 2020 due to the lockdown imposed in April and May 2020 due to the spread of corona, public holidays in the country, and the unnoticed earth leakage issue in September 2020. The significant results from the comparative study are
The actual average final yield of the plant is 115%, whereas the average final yield in the year 2020 is 92%.
The actual average performance ratio of the 52-kW plant is 73%, whereas the average performance ratio in the year 2020 is 60%.
The actual simple payback period of the plant is 4.5 years, whereas it gives 5.6 years with the energy yield obtained from the year 2020.
The actual yearly energy yield of the plant is 72.27 MWh, whereas the annual energy yield in the year 2020 is 57.75 MWh.
The actual energy payback time of the plant is 6.6 years, whereas it gives 8.1 years with the energy yield obtained from the year 2020.
The solutions to the issues mentioned above are incorporating the net metering concept to the grid-connected plant and regular and proper monitoring of the plant. The average global solar irradiation potential and temperature in the study area are more suitable for solar PV systems. Hence, it is required to concentrate on the effective utilisation of the PV plant throughout the year. The future scope of this study will be extended by incorporating the suggestions proposed for the issues raised in this plant. The following are the recommendations and enhancements for further examination of the system. It would be good to measure the temperature of the cells in terms of understanding the influence of environmental factors on the operation of PV plants. A thorough examination of design optimisation and scalability, voltage fluctuations, associated harmonics, and appropriate filters for grid-connected PV systems can also be carried out to address the problems and uncertainties related to power quality issues.
Disclosure statement
No potential conflict of interest was reported by the author(s).
References
- Adaramola, Muyiwa S., and Emil E. T. Vågnes. 2015. “Preliminary Assessment of a Smallscale Rooftop PV-Grid Tied in Norwegian Climatic Conditions.” Energy Conversion and Management 90: 458–465.
- Ayompe, L. M., A. Duffy, S. J. McCormack, and M. Conlon. 2011. “Measured Performance of a 1.72 kW Rooftop Grid Connected Photovoltaic System in Ireland.” Energy Conversion and Management 52 (2): 816–825.
- Aziz, Ali Saleh, Mohammad Faridun Naim Tajuddin, Mohd Rafi Adzman, Mohd Fayzul Mohammed, and Makbul A. M. Ramli. 2020. “Feasibility Analysis of Grid-Connected and Islanded Operation of a Solar PV Microgrid System: A Case Study of Iraq.” Energy 191: 116591.
- Bhattacharyya, Subhes C., Debajit Palit, Gopal K. Sarangi, Vivek Srivastava, and Prerna Sharma. 2019. “Solar PV Mini-Grids versus Large-Scale Embedded PV Generation: A Case Study of Uttar Pradesh (India).” Energy Policy 128: 36–44.
- Buticchi, G., D. Barater, E. Lorenzani, and G. Franceschini. 2012. “Digital Control of Actual Grid-Connected Converters for Ground Leakage Current Reduction in PV Transformerless Systems.” IEEE Transactions on Industrial Informatics 8 (3): 563–572.
- Chakraborty, P., E. Baeyens, P. P. Khargonekar, K. Poolla, and P. Varaiya. 2019. “Analysis of Solar Energy Aggregation Under Various Billing Mechanisms.” IEEE Transactions on Smart Grid 10 (4): 4175–4187.
- Colak, H. Ebru, Tugba Memisoglu, and Yasin Gercek. 2020. “Optimal Site Selection for Solar Photovoltaic (PV) Power Plants Using GIS and AHP: A Case Study of Malatya Province, Turkey.” Renewable Energy 149: 565–576.
- Dahmoun, Mouhcen El-Hadi, Bennaissa Bekkouche, K. Sudhakar,Mohammed Guezgouz, Abdessalam Chenafi, and Abdellah Chaouch. 2021. “Performance Evaluation and Analysis of Grid-Tied Large Scale PV Plant in Algeria.” Energy for Sustainable Development 61: 181–195.
- de Lima, Lutero Carmo, Leonardo de Araújo Ferreira, and Francisco Hedler Barreto de Lima Morais. 2017. “Performance Analysis of a Grid Connected Photovoltaic System in Northeastern Brazil.” Energy for Sustainable Development 37: 79–85.
- Dey, Sumon, and Bala Pesala. 2020. “Solar Tree Design Framework for Maximized Power Generation with Minimized Structural Cost.” Renewable Energy 162: 1747–1762.
- Dondariya, Chandrakant, Deepak Porwal, Anshul Awasthi, Akash Kumar Shukla, K. Sudhakar, S. R. Murali Manohar, and Amit Bhimte. 2018. “Performance Simulation of Grid-Connected Rooftop Solar PV System for Small Households: A Case Study of Ujjain, India.” Energy Reports 4: 546–553.
- Edaris, Z. L., M. F. Mohammed, M. S. Saad, S. Yusoff, and M. F. Naim Tajuddin. 2018. “Experimental and Simulated Evaluation of Temperature Effect on Panel Efficiency Performance with Front Water Cooling.” International Conference on Computational Approach in Smart Systems Design and Applications (ICASSDA), Kuching, 1–5.
- Jung, Yoonhwa, Jaehoon Jung, Byungil Kim, and SangUk Han. 2020. “Long Short-Term Memory Recurrent Neural Network for Modeling Temporal Patterns in Long-Term Power Forecasting for Solar PV Facilities: Case Study of South Korea.” Journal of Cleaner Production 250: 119476.
- Khatri, Rahul. 2016. “Design and Assessment of Solar PV Plant for Girls Hostel (GARGI) of MNIT University, Jaipur City: A Case Study.” Energy Reports 2: 89–98.
- Kumar, Ravi, C. S. Rajoria, Amit Sharma, and Sathans Suhag. 2020. “Design and Simulation of Standalone Solar PV System Using PVsyst Software: A Case Study.” Materials Today: Proceedings 46 (11): 5322–5328.
- Lindberg, O., A. Birging, J. Widén, and D. Lingfors. 2021. “PV Park Site Selection for Utility-Scale Solar Guides Combining GIS and Power Flow Analysis: A Case Study on a Swedish Municipality.” Applied Energy 282: 116086.
- Lorenzo, G. D., R. Araneo, M. Mitolo, A. Niccolai, and F. Grimaccia. 2020. “Review of O&M Practices in PV Plants: Failures, Solutions, Remote Control, and Monitoring Tools.” IEEE Journal of Photovoltaics 10 (4): 914–926.
- Malvoni, M., A. Leggieri, G. Maggiotto, P. M. Congedo, and M. G. De Giorgi. 2017. “Long Term Performance, Losses and Efficiency Analysis of a 960kWP Photovoltaic System in the Mediterranean Climate.” Energy Conversion and Management 145: 169–181.
- Moharram, K. A., M. S. Abd-Elhady, H. A. Kandil, and H. El-Sherif. 2013. “Enhancing the Performance of Photovoltaic Panels by Water Cooling.” Ain Shams Engineering Journal 4: 869–877.
- Phylipsen, G. J. M., and E. A. Alsema. 1995. “Environmental Life-Cycle Assessment of Multicrystalline Silicon Solar Cell Modules.” Department of Science, Technology and Society, Utrecht University.
- Pritam Satsangi, K., D. Bhagwan Das, and A. K. Saxena. 2014. “Performance evaluation of 147.5 kWp Hybrid Solar Power Plant.” Conference Proceedings of 38th National Systems Conference, 128–133.
- Rezk, Hegazy, Mohammad Ali Abdelkareem, and Chaouki Ghenai. 2019. “Performance Evaluation and Optimal Design of Stand-Alone Solar PV-Battery System for Irrigation in Isolated Regions: A Case Study in Al Minya (Egypt).” Sustainable Energy Technologies and Assessments 36: 100556.
- Saxena, Anupama Khare, Seema Saxena, and K. Sudhakar. 2021. “Energy, Economic and Environmental Performance Assessment of a Grid-Tied Rooftop System in Different Cities of India Based on 3E Analysis.” Clean Energy 5 (2): 288–301.
- Shah, Nisarg. 2020. “Paradox between Perspectives of Reverse Auction Process for Allotment of Solar PV Projects in India.” Renewable Energy Focus 34: 91–98.
- Shen, Yanbo, Jun Zhang, Peng Guo, and Xinwen Wang. 2018. “Impact of Solar Radiation Variation on the Optimal Tilted Angle for Fixed Grid-Connected PV Array—Case Study in Beijing.” Global Energy Interconnection 1: 460–466.
- Shiva Kumar, B., and K. Sudhakar. 2015. “Performance Evaluation of 10 MW Grid Connected Solar Photovoltaic Power Plant in India.” Energy Reports 1: 184–192.
- Tiwari, Arvind, P. Barnwal, G. S. Sandhu, and M. S. Sodha. 2009. “Energy Metrics Analysis of Hybrid – Photovoltaic (PV) Modules.” Applied Energy 86: 2615–2625.
- Vega Díaz, J. J., M. Vlaminck, D. Lefkaditis, S. A. Orjuela Vargas, and H. Luong. 2020. “Solar Panel Detection within Complex Backgrounds Using Thermal Images Acquired by UAVs.” Sensors 20: 6219.
- Wong, J. H., M. Royapoor, and C. W. Chan. 2016. “Review of Life Cycle Analyses and Embodied Energy Requirements of Single-Crystalline and Multi-Crystalline Silicon Photovoltaic Systems.” Renewable and Sustainable Energy Reviews 58: 608–618.
- Yadav, Satish Kumar, and Usha Bajpai. 2018. “Performance Evaluation of a Rooftop Solar Photovoltaic Power Plant in Northern India.” Energy for Sustainable Development 43: 130–138.