Abstract
Background
The detection of foetal DNA and extravillus trophoblasts (EVTs) in early pregnancy in cervical and uterine samples offers a potential pathway for non-invasive prenatal diagnostics. However, the challenge lies in effectively quantifying these samples. This study introduces a novel approach using the Ras association domain family 1 A (RASSF1A), which exhibits hypermethylation in foetal cells and hypomethylation in maternal cells. The differential methylation pattern of RASSF1A provides a unique biomarker for quantifying foetal cells in cervical and intrauterine samples.
Methods
This study was conducted between September 2022 and May 2023. In total, 23 samples (12 cervical cell samples and 11 intrauterine samples) were collected from women in the Sichuan Jinxin Women & Children Hospital, Jingxiu District, Chengdu, China. The cervical cell samples were collected via lavage and brush techniques, and the intrauterine cell samples were obtained via uterine lavage. These samples were collected as part of a broader effort to advance our understanding of foetal cell dynamics during early pregnancy. The sampling methods were chosen for their minimally invasive nature and their potential in capturing a representative cell population from the respective sites. After digestion of the cell samples using a methylation-sensitive restriction enzyme cocktail, a critical step to differentiate between maternal and foetal DNA, the quantitative polymerase chain reaction (qPCR) of RASSF1A and β-actin (ACTB) were employed to measure foetal DNA and cell concentrations. Immunofluorescence techniques targeting histocompatibility complex, class I G (HLA-G) and GATA binding protein 3 (GATA-3) were employed to detect EVTs in the cell samples and in decidual tissue, with the latter providing an additional layer of confirmation for the presence of foetal cells.
Results
The results showed no hypermethylated RASSF1A was detected in any of the cervical samples, irrespective of whether the samples were obtained by brush or lavage. However, an average of 17,236 ± 7490 foetal cells per sample were detected in the uterine lavage samples. Foetal cells accounted for approximately 0.14% ± 0.10% of the total cell population in these samples. The presence of EVTs in these samples was confirmed by their expression of both HLA-G and GATA-3.
Conclusion
The detection of foetal cells in uterine cavity samples based on hypermethylation of RASSF1A and quantification of foetal cells can be used to prenatal screening. GATA-3 can be used to label EVTs.
PLAIN LANGUAGE SUMMARY
In the realm gestational foetal health, obtaining foetal cells or genetic information is important for detecting and managing potential genetic disorders. Although foetal cells can be obtained from the cervix or uterine cavity, methods to quantify the foetal cell and foetal DNA are lacking. We introduce an innovative technique that utilises DNA restriction endonucleases to selectively isolate foetal DNA and identify foetal cells. We used this technique to measure the number of foetal cells in a sample. Using this technique, we detected foetal cells collected via lavage in uterine but not cervical samples. This finding is significant as it paves the way towards early detection of chromosomal disorders in foetuses, potentially as early as the 10th week of pregnancy. Such early detection offers promising new screening options in early pregnancy, contributing to better prenatal care and outcomes.
1. Introduction
Prenatal diagnostics, including the detection of genetic disorders, depends on the acquisition and analysis of foetal cells. It plays a crucial role in determining whether a foetus might have a genetic disorder. Currently, there are four foetal cell sampling methods: amniocentesis, chorionic villus sampling, and non-invasive prenatal testing (NIPT) of maternal peripheral blood, and collection of foetal cells from the cervix and uterine cavity via brushing and lavage (Stranc et al. Citation1997, Steele and Breg Citation1966, Bianchi et al. Citation2015, Moser et al. Citation2018). There are advantages and disadvantages associated with each method. Among these methods, the collection of foetal cells from the cervix and uterine cavity is particularly valuable, as it can be performed as early as the fifth week of gestation, thereby opening up possibilities for early gestational foetal genetic period (Bolnick et al. Citation2014).
In the past, foetal genetic testing focused on syntrophoblast and extravillus trophoblast (EVTs) via magnetic activated cell sorting. However, recent studies have revealed the presence of foetal cells in cervical mucus. (Kliman et al. Citation1986, Griffith-Jones et al. Citation1992). The dynamics of these cells, including their shedding into the uterine cavity and replacement of epithelial cells in blood vessels and glands (Arutyunyan et al. Citation2023, Moser et al. Citation2010). Histocompatibility complex, class I, G (HLA-G) has emerged as a specific protein marker for EVTs, facilitating their identification and sorting (Apps et al. Citation2008, Bulmer et al. Citation2023). In male foetuses, the sex-determining region Y (SRY) gene can be analysed via the polymerase chain reaction (PCR) and fluorescent in situ hybridisation tests (Griffith-Jones et al. Citation1992, Massari et al. Citation1996), with the short tandem repeat gene aiding the analysis of foetal-derived DNA sequences (Adinolfi et al. Citation1995). Despite advancements foetal cell screening, a simple and effective method to quantify foetal cells in the cervix and uterine cavity remains elusive. The absence of such a method has limited the potential clinical application of cervical cell samples in prenatal diagnostics.
Our study addresses this gap by focusing on the hypermethylated promoter of Ras association domain family 1 A (RASSF1A) in foetal cells. Hypomethylated maternal DNA sequences were removed using a methylation-sensitive restriction enzyme cocktail, paving the way for accurate quantification of foetal RASSF1A via qPCR in samples contain both maternal and foetal DNA (Chan et al. Citation2006, Hromadnikova et al. Citation2010). The methodology offers a novel approach to overcome the challenges in quantifying foetal cells in maternal samples, potentially revolutionising early prenatal diagnostics.
2. Materials and methods
2.1. Ethics approval and sample collection
The study was conducted according to the ethical guidelines of the Medical Ethics Committee of Sichuan Jinxin Xinan Women & Children Hospital, Jingxiu District (2023KY-01), Chengdu, China. Informed consent was obtained from all the participants (N = 23) prior to sample collection. In total, 12 samples from the cervix (lavage), 12 samples from the cervix (brush), 11 samples from in uterine lavage (IUL), and 25 decidual tissue samples (S1 table) were obtained from women undergoing voluntary pregnancy termination (i.e., without medical indication) in the Sichuan Jinxin Xinan Women & Children Hospital (Jingxiu District). The lavage samples were collected using a sterile flexible insemination (Cat. No.1221300; PRODiMED, France) connected to a 20 ml syringe. Sterile saline (5 ml) was gently injected into the uterine cavity or cervix, and 3–5 ml of fluid was aspirated after an interval of 10 seconds. Intrauterine sampling was performed under ultrasound. The brush samples of the cervix were collected using a sterile cervical smear (#B2; HUALAISI, China). The cervical brush was rotated 360 degrees at the cervix and then slowly retracted. All procedures were performed by a senior obstetrician and gynaecologist. The brush head part was immediately placed in 4°C 0.01 M phosphate buffer solution (PBS).
2.2. Sample processing and digestion by methylation-sensitive restriction enzyme cocktail
The collected cell samples were washed three times using sterile PBS and centrifuged at 500 g for 5 minutes at 4°C. Genomic DNA (gDNA) was extracted from the samples using a QIAamp DNA Mini Kit (#51304; Qiagen, Germany), following the manufacturer’s instructions. Subsequently, 800 ng of gDNA were digested with 20 U BstUI (#R0518; New England BioLabs, USA), 10 U BstYI (#R0523; New England BioLabs, USA), and 40 U HhaI (#R0139; New England BioLabs, USA) in 50 μl of 1 × rCutSmart buffer (#B6004;New England BioLabs, USA) at 37° C for 30 minutes and 60° C for 3 hours.
2.3. Quantitative Real-Time PCR (qPCR) of RASSF1A and β-actin
Real-time PCR assays were used for the detection of RASSF1A and β-actin (ACTB-Y and ACTB-N) sequences (Chan et al. Citation2006). Standard curves were generated using known concentrations of human male gDNA (Lo et al. Citation1998). The sequences of the primers and probes are listed in . The contents and reaction conditions of the analysis were identical, except for differences in the primers and probes. Each reaction contained 1× AccuProbe qPCR Master Mix (#A602-01; EXONGEN, China), 6% dimethylsulphoxide, 300 nM PASSF1A primers, 300 nM ACTB-Y primers, 200 nM ACTB-N primers, 150 nM RASSF1A probe, 150 nM ACTB-Y probe, and 100 nM ACTB-N probe with 5 μl of methylated enzyme-digested gDNA. The qPCR conditions were as follows: 25°C for 2 minutes, 94°C for 10 minutes, 95°C for 1 minutes, and 45 cycles of 95 °C for 15 seconds and 60° C for 45 seconds.
Table 1. Primers and probes of qPCR.
2.4. Immunohistochemistry for in uterine lavage (IUL) sample
After collection, the IUL samples were immediately washed with sterile PBS, centrifuged at 500 × g for 5 minutes at 4° C, and diluted to an appropriate cell concentration. Cells (N = 1,000 approx.) were evenly coated on slides and air dried. The slides were soaked with 4% bovine serum albumin (BSA) (#900933; Sigma-Aldrich, USA) buffer for 10 minutes and endogenous peroxidase was closed by 3% hydrogen peroxide. The slides were incubated overnight with the anti-human HLA-G monoclonal antibody (1:1000) (#557577; BD Biosciences, Franklin Lakes, NJ, USA) at 4°C. The slides were then incubated with horseradish peroxidase labelled anti-mouse antibody (1:10000) (#ab205719; Abcam, Cambridge, UK). An aminoethyl carbazole substrate was then added for staining, and nuclei were counterstained with haematoxylin. The slides were covered by cover glass and photographed using a microscope (Nikon e400, Japan).
2.5. Immunofluorescence of decidua tissue and IUL sample
The decidual tissue was fixed with 4% paraformaldehyde for 48 hours, embedded in paraffin, and then sectioned into 4 μm sections. The slides were dewaxed and rehydrated. For antigen retrieval, the slides were pre-treated with Tris-EDTA buffer in a pressure cooker for 5 minutes. The slides were then blocked with 3% hydrogen peroxide for 15 minutes and 4% BSA for 30 minutes at 25 °C and subsequently incubated overnight with primary antibodies at 4°C. The primary antibodies included mouse anti-human HLA-G monoclonal antibody (1:1000) (#557577; BD Biosciences, Franklin Lakes, NJ, USA) and rabbit anti-GATA-3 monoclonal antibody (1:5000) (#5852; Cell Signalling Technology, Danvers, MA, USA). The slides were then incubated with anti-mouse Alexa 488 antibody (1:1000) (#4408; Cell Signalling Technology, Danvers, MA, USA) and anti-rabbit Alexa 555 antibody (1:1000) (#4413; Abcam, Cell Signalling Technology, Danvers, MA, USA). Nuclei were stained with 4′,6-diamidino-2-phenylindole (Cat. No.#D4080; US Everbright, Xiangcheng, China). Images of EVTs were then taken with an AXIO Observer 7 (Zeiss, Oberkochen, Germany).
2.6. Statistical analysis
Statistics were performed using SPSS 21.0 for Windows (SPSS INC., Chicago, IL, USA). A two-tailed probability value of p < 0.05 was considered statistically significant.
3. Results
3.1. Quantitative analysis of the foetal cells in IUL samples
Standard curves for RASSF1A and ACTB-N were generated from suspensions of single cells, providing a foundational framework for the quantitative analysis (, Fig. S1). The results revealed the absence of ACTB-Y amplification products in all the methylated enzyme-digested samples, indicating the successful removal of nonmethylated RASSF1A and confirming that all detected RASSF1A amplification products originated from foetal cells. The total cell number in each sample was calculated based on ACTB-N. There was no significant difference in the age (p = 0.932), body mass index (p = 0.745), or gestational weeks (p = 0.901) of the donors of the cervical or uterine samples (S1 Table). In the cervical samples, no RASSF1A amplification products were detected, either through the brushing or lavage methods. In contrast, foetal cells accounted for 0.14% of the total cell population in the IUL samples, with an average of 17,236 foetal cells detected per sample ().
Figure 1. The standard curves of RASSF1A.
Plot of the input target cell ntunbers (natural logaritlun) against the threshold cycle (Ct) of RASSF1A. Linear function is y = -0.7139 * x + 27. 891. The correlation coefficient is 0.9981.
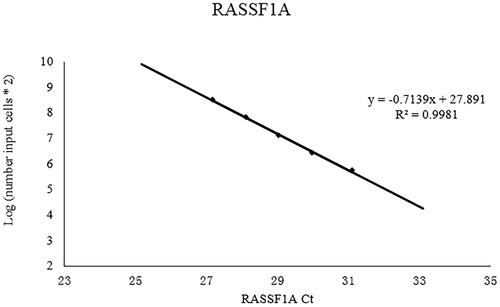
Table 2. Quantitation of the foetal cells in IUL samples.
3.2. Co-expression of HLA-G and GATA-3 in EVTs
To further elucidate the foetal cells obtained from the uterine cavity, we conducted a comprehensive cellular immunohistochemical analysis on the IUL samples. The presence of HLA-G positive signal cells, indicative of EVTs, was a critical finding (). Immunofluorescence analysis of the co-expression of HLA-G and GATA-3 in EVTs in the IUL and decidual tissue samples revealed the co-existence of these proteins (, Fig S2). Co-expression was evident in the nucleus of EVTs in the decidual tissue samples and in the cytoplasm of EVTs in the IUL samples.
Figure 2. EVTs express HLA-G and GATA-3 in IUL samples.
(A, B) HLA-G immunohistochemical staining in EVTs from IUL sample at gestational week 9.6. (C) The immunofluorescence merge figure of EVTs from IUL sample at gestational week 9.6. (D) DAPI staining for nucleus. (E) HLA-G and (F) GATA-3. Magnification: 100X, scale bar = 50 µm.
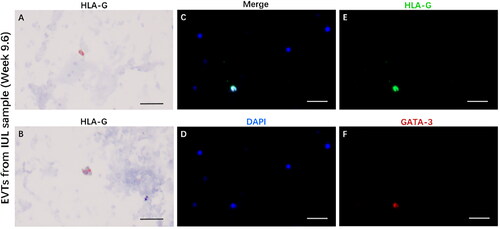
4. Discussion
RASSF1A is a tumour suppressor with a role in stabilising mitotic cyclins and inducing mitotic arrest at prometaphase in cells (Song et al. Citation2004). In this study, we describe the novel application of RASSF1A in prenatal screening. RASSF1A deficiency has been linked to the hypermethylation of a CpG island in its promoter. This hypermethylation characteristic makes RASSF1A an ideal marker for foetal cell detection in pregnant samples (García-Gutiérrez et al. Citation2020, Pan et al. Citation2013). Previous research has demonstrated the use of hypermethylated RASSF1A in maternal plasma in the diagnosis of pre-eclampsia (Mantzaris and Cram Citation2015). Other research has demonstrated the efficacy of using hypermethylated RASSF1A as a control marker for fatal RhD blood genotyping (Chan et al. Citation2006). The results of the present study underscore the sensitivity and versability of hypermethylated RASSF1A in the detection of foetal genetic information.
The methodology for trophoblast retrieval from the cervical and uterine lavage samples in the present study centred on the differential methylation status of maternal-derived versus foetal-derived RASSF1A (Saraswathy et al. Citation2017). This approach allows for the quantification of foetal trophoblasts using qPCR amplification. To enhance the accuracy of the foetal RASSF1A assay, we designed an additional pair of ACTB-Y primers and a probe to test the efficiency of methylation-sensitive enzymatic cleavage (Hromadnikova et al. Citation2010). This dual-primer approach ensured that non-detection of ACTB-Y amplification products indicated the clearance of maternal RASSF1A, leaving only trophoblast-derived RASSF1A amplification products.
The hypermethylated of RASSF1A is present not only with EVTs but also in trophoblast of chorionic villous in the first trimester (Chiu et al. Citation2007). However, as only EVTs express HLA-G, they can be differentiated from trophoblasts and syncytiotrophoblasts (Apps et al. Citation2008). If trophoblasts or syncytiotrophoblasts were present in the uterine cavity, the number of foetal cells quantified by RASSF1A qPCR would be higher than the count of HLA-G positive cells (EVTs).
As foetal cells in cervix or uterine cavity are extremely rare compared to maternal cells, foetal cells would be difficult to use in clinical practice without isolation () (Fritz et al. Citation2015). For instance, in isolating foetal cells from cervical samples, HLA-G protein of EVTs has been used as a specific antigen to bind with magnetic bead antibodies (Bolnick et al. Citation2014). However, HLA-G antigen alone may not be sufficient for identifying foetal cells after isolation. Conversely, beta human chorionic gonadotropin (β-HCG) or cytokeratin 7 (CK7) has also been used as an identification antigen for EVTs (Fritz et al. Citation2015). However, EVTs do not express β-HCG in decidua or chorionic villi, and only the syncytiotrophoblast expresses β-HCG (Wide et al. Citation1988). Moreover, as maternal endocervical epithelial cells are present in the cervix (Bulmer et al. Citation1995), using β-HCG or cytokeratin as an antigen to validate EVTs after isolation by HLA-G is inappropriate. It must be taken to avoid cross-reactivity between HLA-G antibody and secondary antibody of β-HCG or cytokeratin when using immunohistochemical methods to confirm EVTs (Bolnick et al. Citation2014, Hong et al. Citation2022). EVTs express GATA-3 in decidual tissue (Fig. S2) (Arutyunyan et al. Citation2023). In addition, trophoblasts of the chorionic villi express GATA-3 (Lee et al. Citation2016). Based on our results, GATA-3 is a more effective marker than β-HCG or cytokeratin of foetal cells in uterine cavity and cervical samples ().
An important aspect of our study was the comparison of foetal cell yields in cervical and uterine cavity samples. We detected a higher number of foetal cells in the uterine cavity samples than in the cervical samples (Cioni et al. Citation2005), suggesting that although foetal cells are readily obtainable from the uterine cavity, the collection of such cells from cervical samples remains a challenge. Some researchers have likened uterine lavage to a minimally invasive form of chorionic villus sampling, hypothesising regular shedding of trophoblastic cells into the uterine cavity, with only occasional migration to the cervix (Cioni et al. Citation2005). From a clinical application standpoint, uterine lavage may offer a more reliable method than cervical sampling for collecting foetal cells. However, considering potential risks associated with uterine lavage for ongoing pregnancies, it is imperative that such procedures be conducted under ultrasound guidance to ensure patient safety (ErgIn et al. Citation2001).
An important consideration in our study was the inclusion of patients with previous pregnancies. The potential impact of residual foetal cells from prior pregnancies on the measurement of current foetal cell counts in the uterine cavity remains an area for further investigation.
5. Conclusion
Our study provides a gender-independent method for quantifying foetal cells in the uterine cavity and identifies GATA-3 as a suitable marker for EVT immunohistochemistry. As we continue to explore the complexities of foetal cell dynamics, the methodologies and discoveries presented in this study pave the way for more accurate, non-invasive prenatal testing techniques, contributing to improved maternal and foetal health outcomes.
Authors’ contributions
Y. S.: Writing: original draft; conceptualisation. W. L.: Writing: original draft; conceptualisation. J. H.: Methodology. Y. Y.: Investigation. J. Y.: Project administration; Resources. X. F.: Writing: review & editing.
Supplemental Material
Download MS Word (18.2 KB)Disclosure statement
No potential conflict of interest was reported by the authors.
Data availability statement
The data that support the findings of this study are available on doi: 10.6084/m9.figshare.23633739
Additional information
Funding
References
- Adinolfi, M., et al., 1995. Molecular evidence of fetal-derived chromosome 21 markers (STRs) in transcervical samples. Prenatal Diagnosis, 15 (1), 35–39.
- Apps, R., Gardner, L. and Moffett, A., 2008. A critical look at HLA-G. Trends in Immunology, 29 (7), 313–321.
- Arutyunyan, A., et al., 2023. Spatial multiomics map of trophoblast development in early pregnancy. Nature, 616 (7955), 143–151.
- Bianchi, D.W., et al., 2015. Noninvasive prenatal testing and incidental detection of occult maternal malignancies. JAMA, 314 (2), 162–169.
- Bolnick, J.M., et al., 2014. Trophoblast retrieval and isolation from the cervix (TRIC) for noninvasive prenatal screening at 5 to 20 weeks of gestation. Fertility and Sterility, 102 (1), 135–142 e136.
- Bulmer, J.N., et al., 2023. HLA-G positive trophoblastic cells in transcervical samples and their isolation and analysis by laser microdissection and QF-PCR. Prenatal Diagnosis, 23 (1), 34–39.
- Bulmer, J.N., Rodeck, C. and Adinolfi, M., 1995. Immunohistochemical characterization of cells retrieved by transcervical sampling in early pregnancy. Prenatal Diagnosis, 15 (12), 1143–1153.
- Chan, K.C., et al., 2006. Hypermethylated RASSF1A in maternal plasma: a universal fetal DNA marker that improves the reliability of noninvasive prenatal diagnosis. Clinical Chemistry, 52 (12), 2211–2218.
- Chiu, R.W. K., et al., 2007. Hypermethylation of RASSF1A in human and rhesus placentas. The American Journal of Pathology, 170 (3), 941–950.
- Cioni, R., et al., 2005. Comparison of two techniques for transcervical cell sampling performed in the same study population. Prenatal Diagnosis, 25 (3), 198–202.
- ErgIn, T., et al., 2001. Non-invasive early prenatal diagnosis using fuorescent in situ hybridization on transcervical cells: comparison of two different methods for retrieval. European Journal of Obstetrics, Gynecology, and Reproductive Biology, 95 (1), 37–41.
- Fritz, R., et al., 2015. Trophoblast retrieval and isolation from the cervix (TRIC) is unaffected by early gestational age or maternal obesity. Prenatal Diagnosis, 35 (12), 1218–1222.
- García-Gutiérrez, L., et al., 2020. D. RASSF1A tumour suppressor: target the network for effective cancer therapy. Cancers, 12 (1), 229.
- Griffith-Jones, M. D., et al., 1992. Detection of fetal DNA in trans-cervical swabs from first trimester pregnancies by gene amplification: a new route to prenatal diagnosis? British Journal of Obstetrics and Gynaecology, 99 (6), 508–511.
- Hong, K., et al., 2022. Advanced strategy of trophoblasts retrieval and isolation from the cervix (TRIC): comparison of two HLA-G antibodies for immunomagnetic isolation of trophoblasts. Journal of Personalized Medicine, 13 (1), 22.
- Hromadnikova, I., et al., 2010. Quantification of extracellular DNA using hypermethylated RASSF1A, SRY, and GLO sequences—evaluation of diagnostic possibilities for predicting placental insufficiency. DNA and Cell Biology, 29 (6), 295–301.
- Kliman, H.J., et al., 1986. Purification, characterization, and in vitro differentiation of cytotrophoblasts from human term placentae. Endocrinology, 118 (4), 1567–1582.
- Lee, C.Q., et al., 2016. What is trophoblast? A combination of criteria define human first-trimester trophoblast. Stem Cell Reports, 6 (2), 257–272.
- Lo, Y.M., et al., 1998. Quantitative analysis of fetal DNA in maternal plasma and serum: implications for noninvasive prenatal diagnosis. American Journal of Human Genetics, 62 (4), 768–775.
- Mantzaris, D. and Cram, D.S., 2015. Potential of syncytiotrophoblasts isolated from the cervical mucus for early non-invasive prenatal diagnosis: evidence of a vanishing twin. Clinica Chimica Acta; International Journal of Clinical Chemistry, 438, 309–315.
- Massari, A., et al., 1996. Non-invasive early prenatal molecular diagnosis using retrieved transcervical trophoblast cells. Human Genetics, 97 (2), 150–155.
- Moser, G., et al., 2010. Endoglandular trophoblast, an alternative route of trophoblast invasion? Analysis with novel confrontation co-culture models. Human Reproduction (Oxford, England), 25 (5), 1127–1136.
- Moser, G., et al., 2018. Trophoblast retrieval and isolation from the cervix: origins of cervical trophoblasts and their potential value for risk assessment of ongoing pregnancies. Human Reproduction Update, 24 (4), 484–496.
- Pan, J., et al., 2013. Association between RASSF1A promoter methylation and prostate cancer: a systematic review and meta-analysis. PLoS One, 8 (9), e75283.
- Saraswathy, S., et al., 2017. Fetal-specific hypermethylated RASSF1A quantification in pregnancy. The Journal of Maternal-Fetal & Neonatal Medicine, 30 (7), 849–853.
- Song, M.S., et al., 2004. The tumour suppressor RASSF1A regulates mitosis by inhibiting the APC-Cdc20 complex. Nature Cell Biology, 6 (2), 129–137.
- Steele, M.W. and Breg, W.R., 1966. Chromosome analysis of human amniotic-fluid cells. Lancet (London, England), 1 (7434), 383–385.
- Stranc, L.C., Evans, J.A. and Hamerton, J.L., 1997. Chorionic villus sampling and amniocentesis for prenatal diagnosis. Lancet (London, England), 349 (9053), 711–714.
- Wide, M., et al., 1988. Localization of mRNA for the β-subunit of placental hCG by in situ hybridization. Acta Endocrinologica, 119 (1), 69–74.