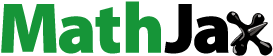
Abstract
Introduction: In human and experimentally induced asthma, a dysfunction of the intra-alveolar-surface active agent (surfactant) has been demonstrated. Type II alveolar epithelial cells (AEII) synthesize, secrete and recycle surfactant. Prior to secretion, intracellular surfactant is stored in specific secretory organelles of AEII. The lamellar bodies (Lb) represent its ultrastructural correlate. The aim of this study was to investigate whether disturbances of the intra-alveolar surfactant are accompanied by alterations in the intracellular surfactant.Material and Methods: Brown-Norway rats were sensitized twice with ovalbumin (OVA) and heat killed Bordetella pertussis bacilli. During airway challenge, an aerosol of 5% ovalbumin/saline solution (0.25 l/min) was nebulized. 24 h after airway challenge, lungs were fixed by vascular perfusion. AEII and their Lb were characterized stereologically by light and electron microscopy.Results: In both groups, AEII were structurally intact. The number of AEII per lung and their number-weighted mean volume did not differ (controls: 49 × 106, 393 µm3; asthmatics: 44 × 106, 390 µm3). A mean of 90 Lb in AEII of asthmatics and of 93 Lb in AEII of controls were evaluated. The Lb mean total volume was 59 µm in asthmatics and 68 µm in controls. Values of both parameters did not reach significance. Also, the size distribution and mean volume of Lb was not influenced by asthma induction, because the volume weighted mean volume of Lb (2.18 µm in asthmatics compared to 1.87 µm in controls) and the numerical weighted mean volume (0.96 µm in asthmatics and 0.75 µm in controls) were comparable in both groups.Conclusion: The obtained results suggest that asthma-induced surfactant dysfunction is not related to disturbances in the intracellular surfactant´s ultrastructural correlates.
Introduction
Pulmonary surfactant (surface active agent) stabilizes alveolar walls and prevents alveolar collapse during exhalation by a surface area depending reduction of alveolar surface tension.Citation1–3 Surfactant can be subdivided in an intra-alveolar pool and an intracellular compartment.Citation4,Citation5 The intra-alveolar pool is represented by the active and inactive surfactant subtypes in the alveolar hypophase of the lining layer covering the alveolar epithelium.Citation6–10 The intracellular surfactant is found in type II alveolar epithelial cells (AEII), which synthesize, store, secrete and recycle surfactant.Citation11 The phospholipids are synthesized in the endoplasmic reticulum and stored in so-called immature lamellar bodies (Lb).Citation12 Mature Lb are formed by fuzing immature Lb with SP-B and -C containing multivesicular bodies (mvb).Citation13,Citation14 SP-B and SP-C containing Lb are secreted by controlled exocytosis, SP-A and SP-D containing mvb by constitutive exocytosis.Citation15,Citation16
Human bronchial asthma is one of the most common diseases.Citation17 Animal models of asthma are widely used.Citation18–23 Drawing conclusions from animal models to human asthmatics depends highly on the animal species selected.Citation18,Citation21 Asthma induction with ovalbumin in rats leads to cellular infiltration of the lung, antigen-specific IgE production, and a predominant Th2 response.Citation21,Citation24 Furthermore, parenchymal infiltration was observed.Citation25–31 Different research groups could verify that surfactant dysfunction was associated with a reduction in minimum surface tension in asthma-induced animalsCitation10,Citation32,Citation33 as well as in patients with asthma.Citation20,Citation34–36 It could be shown that the different proteins passed or secreted into the alveolar hypophase,Citation33,Citation37 particularly the eosinophilic cationic protein (ECP) secreted from eosinophils,Citation38–41 are jointly responsible for surfactant dysfunction.
However, there is no information whether allergic inflammation affects the structural correlate of the intracellular surfactant pool. Therefore, we tested the hypothesis that asthma induced alterations also occur in the AEII and their storage organelles in the asthma model of the Brown Norway (BN) rat.
Material and methods
Male Brown Norway (BN) rats were kept under specific pathogen-free conditions. They had access to food and water ad libitum. Experiments were approved by the local government authority and met the NIH Guidelines for the Care and Use of Laboratory Animals (NIH Publication No. 85, reprint 2002).
Asthma model
Experimental asthma was induced according to a standard protocol for sensitization and challenge.Citation10,Citation42 Rats of the challenge group (n = 7) were sensitized twice both with 1 mg ovalbumin (OVA) (Sigma, Taufkirchen, Germany) and 200 mg Al(OH) 3 (Sigma, Taufkirchen, Germany) in 1 ml 0.9% NaCl solution by subcutaneous application and with 5 × 109 heat-killed Bordetella pertussis bacilli in 0.4 ml 0.9% NaCl solution (kindly donated by Chiron Behring, Marburg, Germany) given intraperitoneally. The sensitization was retried 6-7 days later. Airway challenge (provocation) was carried out another 6-7 days later by nebulization with 5% OVA in 0.9% NaCl as described earlier.Citation10 Rats without any treatment served as controls (home cage controls, n = 5).
Fixation, sampling and tissue processing
Animals were anesthetized and ventilated with a tidal volume of 5 ml and a respiration rate between 30 and 40 breaths per minute as described earlier.Citation10 After thoracotomy and opening of the pericardial cavity a cannula was engaged in the pulmonary artery via the right ventricle. Preperfusion with Krebs-Henseleit solution containing 0.25 g/1900 ml procaine-HCl and in situ perfusion fixation with 1.5 glutaraldehyde and 1.5% paraformaldehyde in 0.15 M HEPES buffer followed immediately after stopping ventilation and exsanguination as described earlier.Citation10 After perfusion fixation, the lungs were fixed by immersion for 24 h. After the determination of lung volume by the Archimedes principle,Citation43 lungs were embedded in 2% aqueous agar, and each organ was cut from apical to caudal into 3 mm slices using a tissue slicer as described in more detail earlier.Citation10 All further tissue processing of the specimens followed a routine protocol.Citation10,Citation44,Citation45
Stereology
Stereological analyses were carried out according to the guidelines for quantitative assessment of lung structure.Citation46 Subcellular volume densities (Vv/AEII) of intracellular surfactant component profiles such as Lb, composite bodies (cb), and multivesicular bodies (mvb) as well as of additional subcellular organelle profiles like nucleus, mitochondria, and cytoplasm were evaluated according to the point counting method.Citation47
We characterized AEII by determining their number, their mean volume and their subcellular composition. Furthermore, the Lb as surfactant storage organelles were characterized by their number and their mean volume. For sampling test fields in light and electron microscopical sections we used the systematic uniform random sampling method that covers all regions with equal probability.Citation46 We used the following parameters to verify possible asthma induced alterations of AEII in asthmatics compared to controls, which are described in detail in reviews of HsiaCitation46 and others.Citation48,Citation49
Using the disector principle, the number of AEII per lung was determined light microscopically and the number of Lb per AEII electron microscopically. Comparing paired sections from the same tissue block with known distance in z direction, AEII were counted if their nucleolus was visible in one section but not in the other section (). Lb were counted if Lb appeared in one section but not in the other (). The height (h) of the light microscopic disector was 2 µm, h of the electron microscopic disector 100 nm.
Figure 1. Disector method, counting of AEII. The number of AEII per lung was determined light microscopically using the disector principle. Comparing paired sections from the same tissue block with known distance in z direction (sampling section for counting, look-up section for comparison), AEII were counted if their nucleolus was visible in the sampling section but not in the look-up section. The section pairs were used for counting in both directions, i.e. using each section once for counting and once for comparison. The height (h) of the light microscopic disector was 2 µm. Scale bar = 25µm.
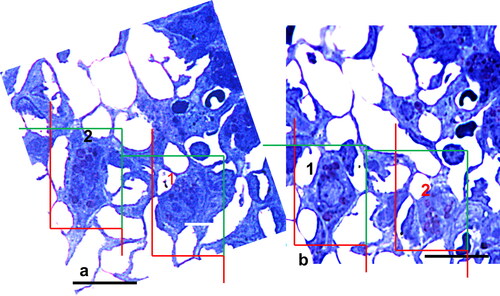
Figure 2. Disector method, counting of Lb. Cellular Lb were counted on paired sections if Lb appeared in one section but not in the other. As for counting of AEII, each section was used once for counting and once for comparison (i.e. counting in both directions). The height (h) of the electron microscopic disector was 100 nm. Scale bar = 2 µm.
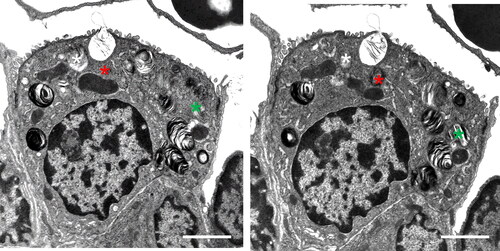
At first, the numerical density of AEII (NV (AEII/par)) and Lb (NV(Lb/AEII)) were evaluated according to the following formula:
where: h = disector height, a(f) = area per test frame, a(p) = area per test point, Q- = counting events
The following additional parameters were calculated:Citation48,Citation50
The number and the number-weighted mean volume (, AEII) of AEII were analyzed using an Axioscope light microscope (Zeiss, Oberkochen, Germany) equipped with a computer-assisted stereology tool box (Cast; Olympus, Ballerup, Denmark). The number-weighted mean volume was evaluated during counting of AEII using the rotator method. The subcellular volume densities of organelles as well as the surface and numerical densities in AEII were evaluated by means of an EM 10 (Zeiss, Oberkochen, Germany) or EM 900 (LEO, Oberkochen, Germany). The volume-weighted mean volume of Lb was evaluated using the point sampled intercepts and a ruler.Citation51 The numberweighted mean volume of Lb was determined by dividing VV(Lb/AEII) by NVLb.Citation50
Statistics
The entire graphics were created with the computer program “GraphPad Prism 6”. In the figures and tables, all data were plotted as arithmetic mean ± standard deviation (SD) if not indicated otherwise. The calculations were done with the statistics program “GraphPad InStat 6”. Stereologic analyses were carried out as evidence of statistical differences, starting at a p < 0.05. We used the non–parametric Mann-Whitney test because the data were not Gaussian distributed.
Results
We fixed the lungs by perfusion fixation in order to examine the distribution of the intra-alveolar and intracellular surfactant without changing their micro-organization. Therefore, we timely performed additional experiments using also the established asthma model of the Brown Norway rat under the same conditions in order to collect functional data and to carry out BAL to determine surfactant activity and numbers of inflammatory cells. The data of these experiments are already published. We summarized these data in the following to verify the allergic inflammation.
Sensitization and challenge with OVA lead to a significant increase of cell numbers and eosinophils in the BALF compared to controls.Citation42,Citation52 We found also a significant higher infiltration of eosinphils in lung parenchyma.Citation52,Citation53 Measuring lung function in intubated and spontaneously breathing animals show a significant increase of lung resistance by 500% in OVA challenged rats compared to controls.Citation10 The minimum surface tension increases significantly by 95% after OVA sensitization and challenge.Citation10
Moreover, sensitization and challenge with OVA led, on the structural level, to a significantly higher alveolar epithelial fraction covered with alveolar fluid as well as to a significantly increased fraction of inactive surfactant compared to controls.Citation10 These results were obtained by evaluating the same perfusion fixed lungs, which are also used for investigation of the intracellular surfactant in the present paper.
Animal weight and lung volume ()
There were no asthma dependent alterations in body weight and lung volume as shown in .
Table 1. Body weight and lung volume.
Table 2. Volume and number of alveolar epithelial cells type II (AEII).
Structural preservation
The alveolar surface was covered by more edematous fluid and more inactive components of the intra-alveolar surfactant in the asthmatics as described earlierCitation10 and documented in .
Figure 3. Alveolar space, alveolar lining layer with hypophase. (a) Control lung: surface active subtypes in the hypophase (tubular myelin, tm) covered by the partly seen surface film, visible also partly in asthma induced lungs. Scale bar = 1µm. (b) Controls: surface active subtypes (tm) and surface inactive subtypes (unilamellar vesicles, ulv (asterisk) and multilamellar vesicles, mlv, arrow), visible also partly in asthmatic lungs. Scale bar = 1µm. (c) OVA sensitized and challenged animals: surface active subtypes and surface film (arrows), which are lifted off by intra-alveolar protein rich fluid accumulation (asterisks), visible only in asthma induced lungs. Scale bar = 1µm. (d) OVA sensitized and challenged animals: Extensive accumulation of inactive surfactant components (ulv and mlv, arrows), visible only in asthma induced lungs. Scale bar = 1µm.
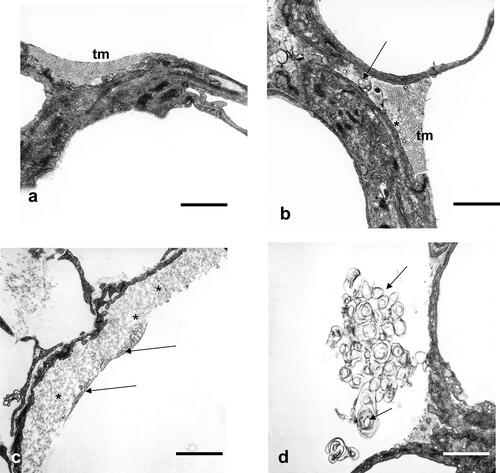
AEII appeared structurally intact in controls and asthma-induced rats (). Subcellular components appeared normally distributed and showed no signs of inflammatory dependent damage. No observable enlarged or very small Lb as possible signs of disturbances in secretion or synthesis were visible.
Figure 4. Type II alveolar epithelial cells (AEII). (a) Control lung: AEII, structurally intact, with intact organelles. Lb appear normal in number and size. Scale bar = 2µm. (b) OVA sensitized and challenged lung: structurally intact AEII. Distribution and size of Lb profiles are qualitatively comparable with controls. No giant or very small Lb profiles or unnatural accumulation as signs of disturbances of intracellular surfactant synthesis are seen. Scale bar = 2 µm.
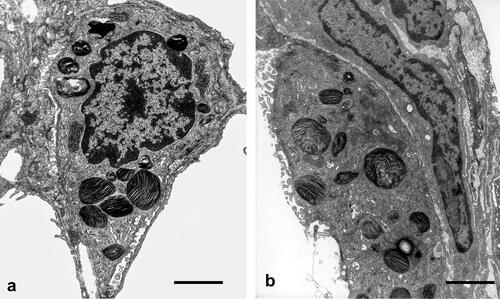
Number and volume of AEII were not affected in the BN rat asthma model ()
The total number of AEII did not differ in controls and OVA sensitized and challenged animals. The number-weighted mean volume of AEII was in the same range in both groups. Thus, a determination of subcellular densities in AEII was possible, without the risk of the reference trap leading to too high or too low densities.Citation50 Looking at the subcellular volume fractions of compartments presented in all animal cells (nucleus, cytoplasm, mitochondria), no alterations between both groups were evaluated (). Thus, quantitative data characterizing AEII were not influenced by induction of experimental asthma.
Table 3. Volume densities of subcellular organells of AEII.
Size distribution, volume and number of Lb in AEII of OVA sensitized and challenged rats are compared to controls ( and )
Table 4. Characterization of intracellular surfactant.
Table 5. Total number and volume of Lb.
To check whether there are possible disturbances in Lb, we determined different parameters characterizing their densities, size, volume and number.
Because the influence of the reference space is negligible, we listed the evaluated VV. The VV of profiles of intracellular surfactant storage organelles as well as the numerical density (NV(Lb,AE II)) of Lb did not differ ().
The volume-weighted mean volume and the numerically weighted mean volume of Lb were not influenced by OVA sensitization and challenge (). Multiplying the NV (Lb,AEII) with the volume of AEII of the same animal, the Lb number in AEII was determined (). AEII of controls contain 93 and of OVA sensitized and challenged rats 90 Lb without reaching significance. Values for total Lb volume and the total Lb surface did also not reach significance.
Discussion
Quantitative ultrastructural investigations by means of stereology of AEII and their Lb after allergen challenge, as presented here, have not been performed until now. Our results show that the allergic inflammation does not influence the intracellular surfactant pool, as assessed by morphological means. Numerically weighted mean cell volume and the number of AEII are comparable in controls and OVA sensitized and challenged animals. The lamellar bodies, as storage organelles of surfactant, represent the morphometric correlate of the intracellular surfactant pool per lung. The total volume of Lb as well as the number of Lb exhibit no differences in OVA sensitized and challenged animals when compared with controls. Furthermore, we found no increase in OVA sensitized and challenged animals of the volume-weighted mean volume of Lb, indicating that the there was no increased variability in Lb volume. The comparable values for the numerically weighted mean volume of Lb also indicate no alterations in the mean volume of single lamellar bodies, independent of the asthma induction. Thus, OVA challenge neither influences the mean variability nor the mean particle volume of Lb. The size distribution of Lb was comparable in both groups. Because of our data, we suggest that neither more or less Lb were synthesized at the smooth ER, nor increased or less amounts of Lb were secreted by exocytosis.
Our results show that there are no clearly visible structural changes of the intracellular surfactant in the asthma model of the Brown Norway rat, which may be responsible for the surfactant dysfunction, as documented by the increase of surface tension and airway resistance in the same asthma model.Citation10 Therefore, we suppose that the inflammatory reaction after allergen challenge induce mainly alterations in the intra-alveolar surfactant pool, as demonstrated by us and others.Citation10,Citation33,Citation34,Citation37,Citation39
It is already accepted that asthma induced inflammation spreads not only into small airways but also into the gas-exchanging alveolar region of lungs.Citation25,Citation27,Citation29,Citation42 Up to now, numerous authors verified that the inflammation is accompanied by an surfactant dysfunction using animal models of asthma or BALF of patients suffering from asthma. They proved an increase of surface tension and an increase of inactive surfactant components in BALF harvested from asthma induced lungs found as one responsible cause.Citation10,Citation34,Citation35,Citation37,Citation54,Citation55
Surfactant dysfunction as a result of augmented inactivation of intra-alveolar surfactant in OVA sensitized and challenged rats may be caused by 1) an IL-4-driven SP-B deficiency after allergen provocation,Citation54 2) secretion products of invaded eosinophils, such as ECP,Citation39 3) inflammatory proteins and or plasma-derived, such as albumin and fibrinogen,Citation37,Citation56,Citation57 4) and by developing inflammatory dependent alveolar edema.Citation58,Citation59 The accumulation of infiltrating mediators of different origins and their interaction with aggregates of alveolar surfactantCitation60 may accelerate the conversion from active to inactive surfactant components and increase the surfactant dysfunction.Citation32
Alterations in the composition of phospholipids, which are not visible microscopically, may also influence the intra-alveolar surfactant activity. e.g., a diminution in phosphatidylglycerol (PG) correlated with increased surface tension combined with an increase of inactive surfactant components.Citation33 Furthermore, it was suggested that the loss of PG by, for instance. increased phospholipase activity, may contribute more to surfactant dysfunction than alterations of surfactant proteins, loss of posphatidylcholine or increased levels of lysophospholipids, or the presence of increased numbers of eosinophils.Citation61 In asthmatic animals compared to controls, decreased surfactant pool sizes and saturated phosphatidylcholine to protein ratios in BALF may predominantly be induced by leaked serum proteins.Citation32 Summarizing the results of other authors, asthma induced inflammation predominantly disturbs the intra-alveolar surfactant pool in different ways.
Lb secretion is regulated by chemical stimuli, such as β-agonists, cholinergic agonists and adenosine, or physical stimuli, such as ventilation.Citation16,Citation62 Furthermore, components of the allergic inflammatory metabolism, such as IL-1 or TNF-α,Citation30,Citation34,Citation63 are also able to influence the secretion of surfactant.Citation16 Thus, disturbances of synthesis, storage and secretion are conceivable. Liu et al. proved a decreased synthesis, storage and activity of phospholipids, measured on isolated AEII.Citation64 They found a significant decrease in cellular phospholipids and phosphatidylcholine synthesis in AEII isolated from immunized and challenged guinea-pigs. The phospholipid content was 33% of control values and the rate of phosphatidylcholine synthesis less than 35%.Citation64 However, these biochemical alterations are not accompanied by clearly visible changes in the morphological correlates of the intracellular surfactant pool.
Taken our results together, the present study has shown that in the asthma model of the Brown Norway rat neither the AEII nor the storage organelles of surfactant exhibit any damage or significant quantitative alterations, as determined by stereological methods. However, a limiting factor of this study is that we used only male rats for our experiments.
Author contributions
AS designed the study, performed the light microscopical evaluation and wrote the manuscript, SF performed the electron microscopical evaluation, TT performed the instillation fixation and JMH performed the experiments. All authors reviewed the manuscript.
Acknowledgements
The authors gratefully acknowledge the expert technical assistance of A. Gerken, S. Freese, H. Hühn (Göttingen), M. Hanke (Hannover), M. Fathollahy (Hannover) and S. Elki (Hannover) and thank S. Schmiedl (M.Ed.) for the linguistic review of the manuscript. Finally, the authors thank Prof. Dr. M. Ochs (Berlin) very much for his support in sample processing as well as for critical reading and for his constructive suggestions improving the manuscript.
Disclosure statement
In accordance with Taylor & Francis policy and our ethical obligation as researchers, the authors declare that they have no known competing financial interests or business interests or personal relationships that could have appeared to influence the work reported in this paper.
Additional information
Funding
References
- Agudelo CW, Samaha G, Garcia-Arcos I. Alveolar lipids in pulmonary disease. A review. Lipids Health Dis. 2020;19(1):122. doi:10.1186/s12944-020-01278-8.
- Avery ME, Mead J. Surface properties in relation to atelectasis and hyaline membrane disease. AMA J Dis Child. 1959;97(5, Part 1):517–523. doi:10.1001/archpedi.1959.02070010519001.
- Clements JA, Avery ME. Lung surfactant and neonatal respiratory distress syndrome. Am J Respir Crit Care Med. 1998;157(4 Pt 2):S59–S66. doi:10.1164/ajrccm.157.4.nhlb1-1.
- Wright JR, Clements JA. Metabolism and turnover of lung surfactant. Am Rev Respir Dis. 1987;136(2):426–444. doi:10.1164/ajrccm/136.2.426.
- Olmeda B, Martinez-Calle M, Perez-Gil J. Pulmonary surfactant metabolism in the alveolar airspace: biogenesis, extracellular conversions, recycling. Ann Anat. 2017;209:78–92. doi:10.1016/j.aanat.2016.09.008.
- Weibel ER, Gil J. Electron microscopic demonstration of an extracellular duplex lining layer of alveoli. Respir Physiol. 1968;4(1):42–57. doi:10.1016/0034-5687(68)90006-6.
- Veldhuizen RA, Inchley K, Hearn SA, Lewis JF, Possmayer F. Degradation of surfactant-associated protein B (SP-B) during in vitro conversion of large to small surfactant aggregates. Biochem J. 1993;295(Pt 1):141–147. doi:10.1042/bj2950141.
- Ochs M, Nenadic I, Fehrenbach A, et al. Ultrastructural alterations in intraalveolar surfactant subtypes after experimental ischemia and reperfusion. Am J Respir Crit Care Med. 1999;160(2):718–724. doi:10.1164/ajrccm.160.2.9809060.
- Fehrenbach A, Ochs M, Warnecke T, et al. Beneficial effect of lung preservation is related to ultrastructural integrity of tubular myelin after experimental ischemia and reperfusion. Am J Respir Crit Care Med. 2000;161(6):2058–2065. doi:10.1164/ajrccm.161.6.9908014.
- Schmiedl A, Hoymann HG, Ochs M, et al. Increase of inactive intra-alveolar surfactant subtypes in lungs of asthmatic Brown Norway rats. Virchows Arch. 2003;442(1):56–65. doi:10.1007/s00428-002-0720-z.
- Canadas O, Olmeda B, Alonso A, Perez-Gil J. Lipid-protein and protein-protein interactions in the pulmonary surfactant system and their role in lung homeostasis. Int J Mol Sci. 2020;21(10):3708.
- Chevalier G, Collet AJ. In vivo incorporation of choline- 3 H, leucine- 3 H and galactose- 3 H in alveolar type II pneumocytes in relation to surfactant synthesis. A quantitative radoautographic study in mouse by electron microscopy. Anat Rec. 1972;174(3):289–310. doi:10.1002/ar.1091740303.
- Weaver TE, Na CL, Stahlman M. Biogenesis of lamellar bodies, lysosome-related organelles involved in storage and secretion of pulmonary surfactant. Semin Cell Dev Biol. 2002;13(4):263–270. doi:10.1016/s1084952102000551.
- Brasch F, Johnen G, Winn-Brasch A, et al. Surfactant protein B in type II pneumocytes and intra-alveolar surfactant forms of human lungs. Am J Respir Cell Mol Biol. 2004;30(4):449–458. doi:10.1165/rcmb.2003-0262OC.
- Rooney SA, Young SL, Mendelson CR. Molecular and cellular processing of lung surfactant. Faseb J. 1994;8(12):957–967. doi:10.1096/fasebj.8.12.8088461.
- Andreeva AV, Kutuzov MA, Voyno-Yasenetskaya TA. Regulation of surfactant secretion in alveolar type II cells. Am J Physiol Lung Cell Mol Physiol. 2007;293(2):L259–L271. doi:10.1152/ajplung.00112.2007.
- Hashmi MF, Tariq M, Cataletto ME, Hoover EL, Asthma (Nursing). 2023. StatPearls Publishing. StatPearls [Internet]. Ref Type: Edited Book
- Pabst R. Animal models for asthma: controversial aspects and unsolved problems. Pathobiology. 2002;70(5):252–254. doi:10.1159/000070737.
- Coffman RL, Hessel EM. Nonhuman primate models of asthma. J Exp Med. 2005;201(12):1875–1879. doi:10.1084/jem.20050901.
- Krug N, Rabe KF. Animal models for human asthma: the perspective of a clinician. Curr Drug Targets. 2008;9(6):438–442. doi:10.2174/138945008784533598.
- Kucharewicz I, Bodzenta-Lukaszyk A, Buczko W. Experimental asthma in rats. Pharmacol Rep. 2008;60(6):783–788.
- Gorska MM. Mouse Models of Asthma. Methods Mol Biol. 2018;1809:351–362.
- Akkoc T, O’Mahony L, Ferstl R, Akdis C, Akkoc T. Mouse models of asthma: characteristics, limitations and future perspectives on clinical translation. Adv Exp Med Biol. 2022;1376:119–133. doi:10.1007/5584_2021_654.
- Skripuletz T, Schmiedl A, Schade J, et al. Dose-dependent recruitment of CD25+ and CD26+ T cells in a novel F344 rat model of asthma. Am J Physiol Lung Cell Mol Physiol. 2007;292(6):L1564–L1571. doi:10.1152/ajplung.00273.2006.
- Kraft M, Djukanovic R, Wilson S, Holgate ST, Martin RJ. Alveolar tissue inflammation in asthma. Am J Respir Crit Care Med. 1996;154(5):1505–1510. doi:10.1164/ajrccm.154.5.8912772.
- Martin RJ. Small airway and alveolar tissue changes in nocturnal asthma. Am J Respir Crit Care Med. 1998;157(5 Pt 2):S188–S190. doi:10.1164/ajrccm.157.5.rsaa-4.
- Sutherland ER, Martin RJ. Distal lung inflammation in asthma. Ann Allergy Asthma Immunol. 2002;89(2):119–124. doi:10.1016/S1081-1206(10)61926-6.
- Kraft M. Part III: Location of asthma inflammation and the distal airways: clinical implications. Curr Med Res Opin. 2007;23 Suppl 3:S21–S27. doi:10.1185/030079907X226177.
- Hamid Q. Pathogenesis of small airways in asthma. Respiration. 2012;84(1):4–11. doi:10.1159/000339550.
- McVey MJ, Steinberg BE, Goldenberg NM. Inflammasome activation in acute lung injury. Am J Physiol Lung Cell Mol Physiol. 2021;320(2):L165–L178. doi:10.1152/ajplung.00303.2020.
- Shimizu K, Tanabe N, Oguma A, et al. Parenchymal destruction in asthma: fixed airflow obstruction and lung function trajectory. J Allergy Clin Immunol. 2022;149(3):934–942.e8. doi:10.1016/j.jaci.2021.07.042.
- Cheng G, Ueda T, Sugiyama K, Toda M, Fukuda T. Compositional and functional changes of pulmonary surfactant in a guinea-pig model of chronic asthma. Respir Med. 2001;95(3):180–186. doi:10.1053/rmed.2000.1012.
- Hite RD, Seeds MC, Bowton DL, et al. Surfactant phospholipid changes after antigen challenge: a role for phosphatidylglycerol in dysfunction. Am J Physiol Lung Cell Mol Physiol. 2005;288(4):L610–L617. doi:10.1152/ajplung.00273.2004.
- Hohlfeld JM, Ahlf K, Enhorning G, et al. Dysfunction of pulmonary surfactant in asthmatics after segmental allergen challenge. Am J Respir Crit Care Med. 1999;159(6):1803–1809. doi:10.1164/ajrccm.159.6.9806145.
- Meyer KC, Zimmerman JJ. Inflammation and surfactant. Paediatr Respir Rev. 2002;3(4):308–314. doi:10.1016/s1043-6618(02)00212-8.
- Erpenbeck VJ, Fischer I, Wiese K, et al. Therapeutic surfactants modulate the viability of eosinophils and induce inflammatory mediator release. Int Arch Allergy Immunol. 2009;149(4):333–342. doi:10.1159/000205579.
- Liu M, Wang L, Enhorning G. Surfactant dysfunction develops when the immunized guinea-pig is challenged with ovalbumin aerosol. Clin Exp Allergy. 1995;25(11):1053–1060. doi:10.1111/j.1365-2222.1995.tb03251.x.
- Badar A, Saeed W, Hussain MM, Aslam M. Correlation of eosinophil cationic protein with severity of asthma. J Ayub Med Coll Abbottabad. 2004;16(3):66–71.
- Hohlfeld JM, Schmiedl A, Erpenbeck VJ, Venge P, Krug N. Eosinophil cationic protein alters pulmonary surfactant structure and function in asthma. J Allergy Clin Immunol. 2004;113(3):496–502. doi:10.1016/j.jaci.2003.12.008.
- Schmiedl A, Krug N, Hohlfeld JM. Influence of plasma and inflammatory proteins on the ultrastructure of exogenous surfactant. J Electron Microsc (Tokyo). 2004;53(4):407–416. doi:10.1093/jmicro/dfh056.
- Górska K, Paplińska-Goryca M, Nejman-Gryz P, Goryca K, Krenke R. Eosinophilic and Neutrophilic Airway Inflammation in the Phenotyping of Mild-to-Moderate Asthma and Chronic Obstructive Pulmonary Disease. COPD. 2017;14(2):181–189. doi:10.1080/15412555.2016.1260539.
- Schuster M, Tschernig T, Krug N, Pabst R. Lymphocytes migrate from the blood into the bronchoalveolar lavage and lung parenchyma in the asthma model of the brown Norway rat. Am J Respir Crit Care Med. 2000;161(2 Pt 1):558–566. doi:10.1164/ajrccm.161.2.9812021.
- Scherle W. A simple method for volumetry of organs in quantitative stereology. Mikroskopie. 1970;26(1):57–60.
- Fehrenbach H, Ochs M. Studying lung ultrastructure. In: Uhlig S, Taylor AE, eds. Methods in Pulmonary Research. Basel: Birkhäuser; 1998:429–454.
- Fehrenbach H, Schmiedl A, Wahlers T, et al. Morphometric characterisation of the fine structure of human type II pneumocytes. Anat Rec. 1995;243(1):49–62. doi:10.1002/ar.1092430107.
- Hsia CC, Hyde DM, Ochs M, Weibel ER, ATS/ERS Joint Task Force on Quantitative Assessment of Lung Structure An official research policy statement of the American Thoracic Society/European Respiratory Society: standards for quantitative assessment of lung structure. Am J Respir Crit Care Med. 2010;181(4):394–418. doi:10.1164/rccm.200809-1522ST.
- Weibel ER, Hsia CC, Ochs M. How much is there really? Why stereology is essential in lung morphometry. J Appl Physiol (1985). 2007;102(1):459–467. doi:10.1152/japplphysiol.00808.2006.
- Muhlfeld C, Ochs M. Quantitative microscopy of the lung: a problem-based approach. Part 2: stereological parameters and study designs in various diseases of the respiratory tract. Am J Physiol Lung Cell Mol Physiol. 2013;305(3):L205–L221. doi:10.1152/ajplung.00427.2012.
- Ochs M, Muhlfeld C. Quantitative microscopy of the lung: a problem-based approach. Part 1: basic principles of lung stereology. Am J Physiol Lung Cell Mol Physiol. 2013;305(1):L15–L22. doi:10.1152/ajplung.00429.2012.
- Ochs M. A brief update on lung stereology. J Microsc. 2006;222(Pt 3):188–200. doi:10.1111/j.1365-2818.2006.01587.x.
- Gundersen HJ, Jensen EB. Stereological estimation of the volume-weighted mean volume of arbitrary particles observed on random sections. J Microsc. 1985;138(Pt 2):127–142. doi:10.1111/j.1365-2818.1985.tb02607.x.
- Schmiedl A, Luhrmann A, Pabst R, Koslowski R. Increased surfactant protein a and d expression in acute ovalbumin-induced allergic airway inflammation in brown norway rats. Int Arch Allergy Immunol. 2009;148(2):118–126. doi:10.1159/000155742.
- Schmiedl A, Tschernig T, Luhrmann A, Pabst R. Leukocyte infiltration of the periarterial space of the lung after allergen provocation in a rat asthma model. Pathobiology. 2005;72(6):308–315. doi:10.1159/000091328.
- Haczku A, Atochina EN, Tomer Y, et al. The late asthmatic response is linked with increased surface tension and reduced surfactant protein B in mice. Am J Physiol Lung Cell Mol Physiol. 2002;283(4):L755–L765. doi:10.1152/ajplung.00062.2002.
- Winkler C, Hohlfeld JM. Surfactant and allergic airway inflammation. Swiss Med Wkly. 2013;143:w13818. doi:10.4414/smw.2013.13818.
- Seeger W, Grube C, Gunther A, Schmidt R. Surfactant inhibition by plasma proteins: differential sensitivity of various surfactant preparations. Eur Respir J. 1993;6(7):971–977. doi:10.1183/09031936.93.06070971.
- Park J, Bae CW, Choi YM. In vitro inhibition of biophysical surface properties and change in ultrastructures of exogenous pulmonary surfactant by albumin or fibrinogen. J Korean Med Sci. 1998;13(2):123–130. doi:10.3346/jkms.1998.13.2.123.
- Babu KS, Woodcock DA, Smith SE, Staniforth JN, Holgate ST, Conway JH. Inhaled synthetic surfactant abolishes the early allergen-induced response in asthma. Eur Respir J. 2003;21(6):1046–1049. doi:10.1183/09031936.03.00069202.
- Blanco O, Ramirez W, Lugones Y, et al. Protective effects of Surfacen(R) in allergen-induced asthma mice model. Int Immunopharmacol. 2022;102:108391. doi:10.1016/j.intimp.2021.108391.
- Wright SM, Hockey PM, Enhorning G, et al. Altered airway surfactant phospholipid composition and reduced lung function in asthma. J Appl Physiol (1985). 2000;89(4):1283–1292. doi:10.1152/jappl.2000.89.4.1283.
- Cochrane CG. Pulmonary surfactant in allergic inflammation: new insights into the molecular mechanisms of surfactant function. Am J Physiol Lung Cell Mol Physiol. 2005;288(4):L608–L609. doi:10.1152/ajplung.00434.2004.
- Rooney SA. Regulation of surfactant secretion. Comp Biochem Physiol A Mol Integr Physiol. 2001;129(1):233–243. doi:10.1016/s1095-6433(01)00320-8.
- Lappalainen U, Whitsett JA, Wert SE, Tichelaar JW, Bry K. Interleukin-1beta causes pulmonary inflammation, emphysema, and airway remodeling in the adult murine lung. Am J Respir Cell Mol Biol. 2005;32(4):311–318. doi:10.1165/rcmb.2004-0309OC.
- Liu M, Wang L, Holm BA, Enhorning G. Dysfunction of guinea-pig pulmonary surfactant and type II pneumocytes after repetitive challenge with aerosolized ovalbumin. Clin Exp Allergy. 1997;27(7):802–807. doi:10.1046/j.1365-2222.1997.420885.x.