Abstract
Oral microbiome research has gained significant interest in recent years due to its potential impact on overall health. Smoking has been identified as a significant modulator of the oral microbiome composition, leading to dysbiosis and possible health consequences. Research has primarily focused on the association between smoking and oral microbiome, as well as smoking’s association with cardiometabolic syndrome (CMS). This narrative review presents an overview of the recent findings and current knowledge on the oral microbiome and its role in CMS, including the effects of smoking and ethnicity. We discussed the development and composition of the oral microbiome and the association of periodontitis with diabetes and cardiovascular diseases. Furthermore, we highlighted the correlations between oral microbiome and CMS factors, such as diabetes, hypertension, dyslipidemia, and obesity. There is a need for further research in this area to better understand the mechanisms underlying the impact of smoking on oral microbiome dysbiosis and the development of CMS. Interestingly, geographic location and ethnicity have been shown to impact the oral microbiome profiles across populations. This knowledge will help develop personalized disease prevention and treatment approaches considering individual differences in oral microbiome composition. Understanding the complex interplay between oral microbiome, smoking, and CMS is essential for developing effective prevention and treatment strategies for a wide range of diseases.
Background
The human microbiome is a complex ecosystem comprising 10 to 100 trillion symbiotic microbial cells, including bacteria, viruses, fungi, and protozoa.Citation1 Microbiome projects worldwide have been dedicated to understanding the role of microorganisms in health and disease. The human microbiome project, for instance, was committed from 2007 to 2016 and focused on determining the microorganisms that make up the microbiome in five body sites, including the skin, oral cavity, nasal cavity, gastrointestinal tract, and urogenital tract.Citation2 One key area of interest is the oral microbiome, which is critical in maintaining oral health and preventing diseases.
Recent studies have indicated that the oral microbiome is highly dynamic and can be affected by several factors, such as cardiometabolic syndrome (CMS). CMS describes a cluster of metabolic disorders that includes insulin resistance (IR), dyslipidemia, hypertension, and obesity, which could lead to the development of type 2 diabetes (T2D) and cardiovascular disease (CVD). CMS is mostly preventable and treatable; however, it is one of the highest causes of mortality worldwide, with 22.4 million deaths.Citation3,Citation4 Alarmingly, almost half of these deaths were categorized as “premature deaths,” occurring before the age of 60 and often leading to a significant reduction in the quality of life for affected individuals.Citation3,Citation4
There has been emerging evidence that the oral microbiome may be involved in the pathogenesis of CMS factors and may be influenced by lifestyle factors such as smoking. Smoking is one of the habits worldwide that has significantly impacted the oral microbiome. Several studies have studied the role of smoking on the oral microbiome in different populations.Citation5–7 However, the mechanisms between oral microbiome, smoking, and CMS are largely unexplored. The physiological effects of numerous chemicals found in smoking have not received sufficient research attention, and there is even less understanding of how the oral microbiome might influence the interactions between cigarette smoke and human physiology. The circulatory system serves as a pathway through which oral microbiome bacteria can potentially impact the risk of CMS. The blood supply associated with each tooth allows metabolites or endotoxins produced during oral bacterial metabolism to enter the bloodstream.Citation8 Consequently, this process can lead to systemic inflammation, affecting various other parts of the body.Citation8 A recent study conducted in China found that smoking was associated with enrichment of four bacterial genera in the oral microbiome (Anaeroglobus, Megasphaera, Actinomyces, and Rothia), which were linked to elevated triglyceride levels.Citation9 Additionally, Anaerglobus was negatively associated with HDL-C levels.Citation9 Overall, there is still a lack of evidence on the role of oral microbiome in CMS and the effects of smoking in different populations. This narrative review aims to provide an overview of the current knowledge.
Oral microbiome overview
Microorganisms residing in the oral cavity are referred to as the oral microbiome. It includes various distinct habitats, which include teeth, gums, tongue, hard palate, soft palate, cheeks, and lips. Adjacent anatomical structures such as tonsils, pharynx, middle ear, trachea, esophagus, eustachian tube, nasal passages, and sinuses are included in the human oral microbiome. The human oral microbiome is defined as all microorganisms present on or within the oral cavity and its contagious extension, except for the distal esophagus. However, most studies and samples have been from the oral cavity itself.Citation10
The healthy oral microbiome harbors approximately 50–100 million bacteria belonging to 700 species and is the second most abundant and diverse microbiome in the human body, following the gut microbiome Citation11–13 The oral cavity region provides an optimal environment for microorganism growth with an average temperature of 37 °C and a saliva pH consistently between 6.5–7.5, allowing bacteria to thrive in a stable environment.Citation14 Moreover, saliva serves as a source of hydration for microorganisms and functions as a transport medium for nutrients to microorganisms. The oral microbiome and its host have a mutually dependent and evolutionary relationship characterized by continuous communication.Citation15 The oral microbiome plays diverse roles, including physiological, immunological, metabolic, mucosal protection, nutritional, and detoxifying functions.Citation15,Citation16
The development of the oral microbiome starts at a very early stage of life. During delivery, the newborn encounters the microflora of the mother’s uterus and vagina and later with the other microorganisms in the atmosphere during delivery.Citation13 Initially, the newborn’s oral cavity is usually sterile, but it becomes inoculated with microorganisms from the first feeding, and the residential oral microflora acquisition process starts.Citation17 Streptococcus salivarius and Streptococcus mitis are pioneer species that inhabit the oral cavity at the early stages after birth.Citation18 In the first year after birth, the oral cavity is mainly invaded by aerobic bacteria, including Streptococcus, Lactobacillus, Actinomyces, Neisseria, and Veillonella.Citation19 Later, after teeth eruption, anaerobic microorganisms, such as Prevotella, Fusarium, and more, dominate the environment that exists between the gums and teeth.Citation18 Streptococcus species, including Streptococcus parasanguis and Streptococcus mutans, can grow on enamel and colonize gingival epithelial surfaces.Citation19
The Human Microbiome Project (HMP) was initiated in 2007 as a collaborative effort between the National Institute of Health (NIH) and the International Human Microbiota Consortium (IHMC).Citation20 The main objective was to comprehensively characterize the human microbiota on a large scale and explore their significance in relation to human health and disease.Citation20 According to the expanded Human Oral Microbiome Database (eHOMD) (https://homd.org/, accessed on 16 April 2023), there are 774 oral bacterial species, 58% are named, 16% have been cultivated but unnamed, and 26% have been identified through DNA analysis but yet to be cultivated; 96% of the total oral microbiome belongs to six broad phyla: Firmicutes, Actinobacteria, Proteobacteria, Fusobacteria, Bacteroidetes, and Spirochetes.Citation21 These oral microorganisms significantly impact various aspects of human health, ranging from the host metabolic process to immune responses.Citation22
Microbial dysbiosis is an imbalance in the microorganisms of the host. Indeed, oral microbiome dysbiosis has a high chance of spreading into adjacent epithelial surfaces, leading to potentially infectious diseases. Moreover, studies have linked oral bacteria dysbiosis to several systematic diseases, including CVD and diabetes.Citation23–28 By understanding the role of the oral microbiome in health and disease, researchers can develop better treatments and strategies to promote oral health.
Oral microbiome and periodontitis
Periodontitis is a bacterially induced chronic inflammatory disease.Citation29 The disease is characterized by bone and connective tissue loss, tooth mobility, and, ultimately, tooth loss.Citation30 The host’s immunological response is responsible for 80% of tissue destruction, while oral bacteria account for the remaining 20%.Citation29 Poor oral hygiene practices lead to plaque accumulation, triggering a chronic inflammatory response and affecting the gingival tissues. Gingivitis is the earliest periodontal disease stage and is reversible with simple oral hygiene practices.Citation29,Citation31 However, if left untreated, it can proceed into periodontitis due to persistent plaque accumulation and the patient’s response to the bacterial challenge.Citation29 Periodontitis is commonly associated with anaerobic Gram-negative bacteria such as Porphyromonas gingivalis, Prevotella intermedia, and Spirochetes such as Treponema denticola.Citation32–34
Oral bacteria can enter the bloodstream through various activities, including eating, flossing, and tooth brushing, leading to bacteremia.Citation35 Studies have shown that periodontitis is linked to CVD due to the pathogenic oral bacteria found in atherothrombotic mice tissue.Citation36,Citation37 The local inflammatory response triggers pro-inflammatory cytokines, which can also enter the bloodstream and initiate an acute inflammatory response.Citation38 Eventually, it results in a local inflammatory response and triggers pro-inflammatory cytokines such as interleukin-1 beta (IL-1β), interleukin-6 (IL-6), and tumor necrosis factor-alpha (TNFα), which can also enter the bloodstream and initiate an acute inflammatory response.Citation38 Chronic systemic inflammation caused by these cytokines can increase the risk of developing CVD, hypertension, and T2D.Citation35,Citation38 Additionally, the release of reactive protein can activate cytokines and oxidative stress response, further exacerbating chronic systemic inflammation.Citation38
Inflammation in periodontitis also drives dysbiosis by promoting the growth of pathogenic bacteria that can utilize nutrients released from the destruction of periodontal tissues, such as collagen fragments and heme-containing compounds.Citation39,Citation40 These bacteria, including Porphyromonas gingivalis, can thrive in low-oxygen environments created by inflammation and have an increased ability to cause inflammation.Citation41 They are known as “inflammophilic pathobionts” and can upregulate virulence-associated genes in response to certain nutrients.Citation40 The overgrowth of these bacteria is a major contributor to the development of periodontitis.Citation42
A meta-analysis has identified individuals with periodontitis to have a moderate to increased risk of developing coronary heart disease, ischemic stroke, and CVD.Citation43–46 Another meta-analysis has also resulted in the correlation of periodontitis increasing the risk of hypertension.Citation47 Evidence shows periodontitis treatment decreased CVD markers, consisting of C-reactive protein and circulating lipid.Citation36,Citation48 Moreover, diabetic patients had a 24% risk of developing cardiometabolic syndrome (CMS); periodontitis patients had a 26% risk of developing diabetes.Citation49
Porphyromonas gingivalis, Treponema denticola, and Tannerella forsythia are common bacteria found in the subgingival biofilms that contribute to the development of periodontitis.Citation50 Recent studies have found an association between Treponema and Corynebacterium bacteria for the development of periodontitis and CMS in subgingival plaque and saliva samples.Citation51 Furthermore, Filifactor alocis and Fretibacterium fastidiosum were found to be dominant in subgingival plaque of periodontitis patients who smoke.Citation52
Treatment for periodontitis has been shown to improve CVD markers, such as C-reactive protein and circulating lipids.Citation53 Furthermore, diabetic and periodontitis patients are at an increased risk of developing CMS and diabetes. Therefore, early detection and management of periodontitis is essential and can help in reducing the risk of developing CMS.
Oral microbiome influence on cardiometabolic syndrome
CMS is also known as metabolic syndrome x. As previously mentioned, it is a combination of metabolic diseases that includes a combination of diabetes mellitus (DM), hypertension, central obesity, and dyslipidemia. Studies have shown a strong link of CMS in developing atherosclerotic CVD, peripheral vascular disease, coronary artery disease, myocardial infarction, cerebrovascular Diabetes Mellitus disease, stroke, and DM.Citation54–56 An essential factor of CMS is obesity, which is on the rise globally where it is estimated that 1.1 billion adults are overweight and 312 million are obese.Citation57 Although obesity is well-recognized risk of developing CMS, a study revealed middle-aged men with CMS are at an increased risk of developing CVD and related deaths regardless of their Body mass index (BMI).Citation58
There are various internationally recognized definitions of CMS used. According to the National Cholesterol Education Program Adult Treatment Panel III (NCEP-ATP III), CMS is defined as the presence of 3 or more clinical abnormalities, which include: dyslipidemia, central obesity, systemic arterial hypertension, and hyperglycemia.Citation59 European Group for Study of Insulin Resistance (EGIR), the American Association for Clinical Endocrinology (AACE), and the International Diabetes Federation (IDF) are international organizations and have slightly different criteria for CMS; however, all include visceral obesity and insulin resistance.Citation60,Citation61 However, the World Health Organization (WHO) and ATPIII diagnostic criteria are more widely used. The WHO defines CMS as the presence of DM and IR as the primary factors and other risk factors such as obesity, high triglycerides, reduced high-density lipoprotein (HDL), hypertension, or micro-albuminuria.Citation61 EGIR and ATPIII defined obesity in CMS as visceral obesity rather than total obesity or overweight.Citation57 The effects of hyperglycemia, hypertension, dyslipidemia, and obesity on the oral microbiome will be discussed below. summarizes the oral microbiome of the different CMS.
Figure 1. Oral microbiome bacterial enrichment or depletion in cardiometabolic syndrome (CMS). Cardiovascular disease (CVD), type 2 diabetes (T2D), and obesity.
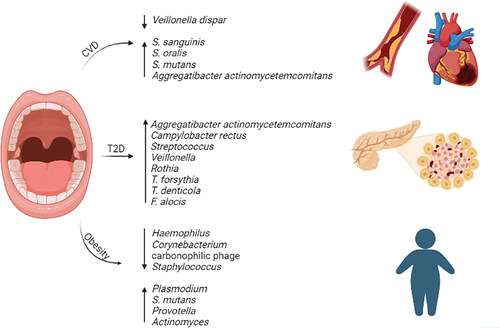
Hyperglycemia
Hyperglycemia is the increase of glucose in the bloodstream. The hemoglobin A1c (HbA1c) test is used to evaluate the percentage of the hemoglobin glycosylation and a person’s level of glucose control.Citation62 A level below 5.7% HbA1c is considered normal, 5.7 to 6.4% HbA1c is considered as pre-diabetic and a level higher than 6.7% HbA1c is considered diabetic.Citation62 Diabetes is a chronic metabolic disorder, it is one of the top 10 causes of mortality worldwide; in 2019, 463 million individuals were living with diabetes, which is expected to increase by 2045 to 700 million.Citation63
Studies have revealed a strong association between diabetes and both the gut and oral microbiome. In particular, diabetes is bidirectionally linked to periodontitis.Citation31,Citation64–66 This association is believed to be due to several factors, including increased glucose levels in gingival crevicular fluid and saliva, which provides a favorable environment for the growth of pathogenic bacteria. In addition, pH changes and reduced saliva flow associated with diabetes can cause alterations in the oral microbiome.
Interestingly, studies have suggested that individuals with periodontitis are at a higher risk of developing diabetes, and individuals with diabetes are more likely to develop periodontitis.Citation67 For example, a study conducted on Hispanic patients with T2D and periodontitis found that the most frequently isolated microorganisms from periodontitis sites were the red-complex bacteria, such as Aggregatibacter actinomycetemcomitans and Campylobacter rectus.Citation67 Miranda et al. (2017) investigated the impact of glycemic control on the abundance of periodontal pathogens (Treponema denticola, Porphyromonas. gingivalis, Tannerella forsythia, Eubacterium nodatum, Parvimonas micra, Fusobacterium nucleatum ssp., and Prevotella intermedia) in T2D who presented generalized chronic periodontitis.Citation68 They collected subgingival biofilm samples from patients with poor and good glycemic control.Citation68 The analysis revealed individuals with poor glycemic control had significantly higher levels of Fusobacterium. nucleatum ssp. and detection frequencies of Tannerella forsythia, Eubacterium nodatum, Parvimonas micra, and Fusobacterium nucleatum ssp, indicating poor glycemic control is associated with elevated levels and frequencies of periodontal pathogens.Citation68 In another study, researchers found an increase in aciduric species, including Streptococcus, Veillonella, and Rothia, in T2D patients who adhere to a Mediterranean diet.Citation69
Furthermore, specific oral microbiome profiles have been associated with the development of insulin resistance. Recent studies investigated the subgingival plaque of periodontitis patients with and without diabetes and found that periodontitis diabetic patients had significantly higher levels of Tannerella forsythia, Treponema denticola, and Filifactor alocis.Citation70 Notably, nondiabetic patients also showed a correlation between red complex species and Filifactor alocis and Fretibacterium fastidiosum.Citation70 These findings suggest that the oral microbiome may play a role in the development and progression of diabetes and periodontitis.
Hypertension
Hypertension is a major contributor to global morbidity and mortality.Citation71 It is associated with various metabolic and cardiovascular complications, posing a significant burden on global health and leading to years of life lost as a result of disability.Citation71,Citation72 The current diagnostic parameter for hypertension is based on systolic pressure values above 130 mmHg and diastolic pressure above 80 mmHg.Citation73
The salivary microbiome has emerged as a potential factor associated with hypertension. A 2015 study revealed a reciprocal correlation between a chlorhexidine-based mouthwash and Veillonella dispar, a nitrate-reducing bacteria, along with an increase in systolic blood pressure.Citation74 However, our earlier study demonstrated an increase in nitrate-reducing microbes in hypertensive patients,Citation71 indicating possible variations in microbial profiles among different hypertensive populations.
A recent study conducted on 1190 Qatari individuals investigated the differences in the salivary microbiome of hypertensive and normotensive individuals.Citation75 The analysis of differential abundance revealed that Bacteroides and Atopobium were significant members associated with hypertensive groups.Citation75 Normotensive individuals exhibited higher alpha diversity compared to hypertensive individuals and with beta diversity the normotensive individuals were significantly different from hypertensive individuals.Citation75
Studies exploring the subgingival microbiome in relation to hypertension have shown intriguing findings. In one study, in subgingival plaque of hypertensive individuals exhibited an increased abundance of Actinobacillus actinomycetemcomitans, while Streptococcus was significantly more abundant in normotensive individuals.Citation76 Additionally, Treponema denticule was more prevalent in supragingival region and prosthetic materials of hypertensive patients.Citation76 Furthermore, salivary nitric oxide levels were inversely associated with hypertension.Citation76
Elevated relative abundance of Fusobacterium in subgingival samples of hypertensive individuals has been reported.Citation77 Moreover, a decrease in the relative abundance of Actinomyces and increase in Selenomonas in subgingival plaque specimens was correlated with elevated blood pressure.Citation77 Furthermore, the relative abundance of Streptococcus and several of its species were decreased in saliva and oral samples of hypertensive and ischemic stroke compared to individuals with no CVD.Citation77–79
Dyslipidemia
Dyslipidemia, is defined as elevated concentrations of plasma triglycerides, reduced level of HDL concentration, and an increase in the levels of Low-Density Lipoprotein (LDL) concentrations. Dyslipidemia and pro-inflammatory cytokines play a crucial role in the development of atherosclerosis, a major underlying factor contributing to CVD.
Furthermore, the role of dyslipidemia in CVD is significant and is causing a health problem worldwide, with an increased mortality rate from 12.1 million in 1990 to 18.6 million in 2019. Research has identified that the microbiome may play a crucial role in CVD development. Oral microbiome dysbiosis can lead to gut dysbiosis by traveling through saliva, which can result in the release of endotoxins into the circulation, promoting CVD, heart failure, and left ventricular dysfunction.Citation80 Heart failure is also associated with lipopolysaccharide, a gram-negative cell wall product that activates dysregulated systematic inflammation.Citation81
Elevated levels of Aggregatibacter actinomycetemcomitans were detected in saliva samples and subgingival plaque, and have been associated with ischemic stroke and various cardiovascular conditions, including coronary artery disease, acute coronary symptoms, and valvular heart disease.Citation82–85 Furthermore, it has been found in samples of coronary artery atherosclerotic tissue, suggesting its potential role in atherosclerosis development.Citation83,Citation86 The effect of periodontitis has been associated with the development of CVD. Individuals with periodontitis experience endotoxins in their bloodstream, leading to low-level inflammation.Citation84,Citation87 Chronic inflammation can accelerate atherosclerotic plaques, increase inflammation permeability of the blood vessels, and increase the risk of thrombosis.Citation84,Citation87 Interestingly, a study found an inverse relationship between the levels of IgG antibodies to Tannerella forsythia and CVD mortality risk, specifically in men who had previously had heart attacks.Citation88 These findings suggest a complex relationship between the oral microbiome and CVD, which requires further investigation.
Multiple species of Fusobacterium were detected in both subgingival plaque and samples of coronary artery atherosclerotic plaques.Citation89 Porphyromonas gingivalis was identified in both subgingival and coronary artery atherosclerotic plaque samples either independently or in conjunction with several other species, such as Eikenella corrodens, Tannerella forsythia, Tannerella denticola, and Campylobacter rectus.Citation89,Citation90
Studies have explored the association between the oral microbiome and heart valve defects. One study sampled the heart valve during heart valve replacement surgery and cut it into two halves; each part was cultivated in different conditions (aerobic and nonaerobic).Citation91 Seven gram-positive bacteria were identified; three were typical oral bacteria (Streptococcus sanguinis, Streptococcus oralis, and Streptococcus sp.), while Cutibacterium acnes, which was the most abundant species and is also part of the skin normal flora.Citation91,Citation92 A prior study also found that cardiac valve samples exhibiting high rates of gingivitis and/or periodontitis contained oral bacteria, specifically Streptococcus mutans.Citation93 This confirms a previous study where Streptococcus mutans and Aggregatibacter actinomycetemcomitans were the abundant oral bacteria in cardiovascular specimens.Citation94 In addition, Veillonella was detected in whole mouth samples and in samples of carotid artery atherosclerotic plaque.Citation95
Obesity
Obesity is a complex metabolic disorder characterized by excessive adipose tissue accumulation that increases the risk of chronic disease, including T2D, CVD, and certain types of cancer. Recent research has focused on the role of oral microbiome in the pathogenesis of obesity. Studies have reported alterations in the composition and diversity of obese oral microbiome compared to lean individuals. Tam et al. (2018) conducted a study to explore whether obesity influences the composition and diversity of the oral microbiome.Citation96 They collected subgingival and saliva samples from 18 patients with T2D, including 6 in which are obese (BMI ≥ 30 Kg/m2) and 12 non-obese (BMI ˂ 30) most of them had periodontitis.Citation96 The study revealed in the subgingival of normal weight individuals there was a higher abundance of Bacteroidetes, Spirochetes, Firmicutes, Treponema spp., Selenomonas spp., and Filifactor spp.Citation96 On the other hand, obese individuals had a greater abundance of Proteobacteria, Firmicutes, Chloroflexi spp., and Campylobacter spp., with Bacteroidetes being nearly absent.Citation96 Similar patterns were observed in saliva with normal weight individuals; normal weight individuals had a higher abundance of Bacteroidetes and Firmicutes, while obese individuals had a higher abundance of Firmicutes.Citation96 The differences in microbial composition and diversity between obese and normal weight individuals were statistically significant indicating reduced species diversity in the obese group.Citation96
Certain bacterial species, such as Provotella and Actinomyces, have been shown to increase the salivary microbiome of obese individuals without periodontitis.Citation97 However, recent studies have found that obese individuals have a significantly low abundance of specific bacteria, including Haemophilus, Corynebacterium, Carbonophilic phage, and Staphylococcus, but an increase in the abundance of Plasmodium, Streptococcus genus, and Streptococcus mutans.Citation98,Citation99 In our previous study, we observed a higher abundance of proteobacteria and Firmicutes/Bacteroidetes ratio (a recognized obesogenic microbiome trait) in obese insulin-resistant and nondiabetic adults.Citation100
In another study, 647 obese and 969 non-obese individuals’ mouth rinse samples were analyzed.Citation101 Five taxa in Firmicutes and two each in Proteobacteria and Actinobacteria were significantly associated with increased obesity.Citation101 Bifidobacterium and Lactobacillus were associated with decreased obesity prevalence, lower weight gain and BMI.Citation101 A recent study on saliva samples on 3–4 years of age using shotgun metagenomics. Firmicutes, Actinobacteria, and Bacteroidetes phylum were linked to weight gain during the initial two years of life.Citation102 As BMI increased, the diversity of the oral microbiome decreased.Citation102 This suggests that alterations in body composition influenced the diversity of the oral microbiome, potentially contributing to an elevated risk of developing diseases in adulthood.Citation102,Citation103
Impact of smoking on cardiometabolic syndrome
The impact of smoking on the oral microbiome is significant, and it is also considered as an underlying cause and a significant risk for CMS. Meta-analysis studies have shown that smokers have a 1.26 times higher chance of developing CMS than nonsmokers.Citation104 It has also been indicated that smokers and passive smokers are associated with increased susceptibility to metabolic disturbances among adolescents.Citation105
Smoking has been associated in promoting insulin resistance leading to diabetes as well as hypertension and dyslipidemia.Citation106,Citation107 Notably, smoking is associated with visceral obesity, which plays a role in CMS. Multiple studies have shown a positive correlation between smoking and visceral obesity due to its harmful effects on adipose tissue, altering its secretion pattern, lipolysis, and differentiation.
However, smoking cessation can reduce the risk of developing CMS and CVD and subsequently lower mortality rates.Citation108,Citation109 Nevertheless, it should be emphasized that lifestyle modification should occur alongside smoking cessation, as it may lead to weight gain if not managed correctly.Citation110 A recent study conducted found smokers had increased levels of dyslipidemia, body mass index, and central obesity, in addition to higher von Willebrand factor (vWF) protein functional activity increased troponin I levels in smokers, indicating a higher susceptibility to cardiovascular mortality among smokers.Citation4
Overall, there is a lack of studies on the impact of smoking on CMS and cardiovascular health is multifaceted, involving various physiological factors, and understanding its influence on the oral microbiome adds to the complexity of its effects on overall health.
The impact of smoking on the oral microbiome
Smoking has a profound impact on the oral microbiome in various populations. Cigarette smoke contains several toxic compounds that affect the gut and oral microbiota and induce dysbiosis.Citation111 The toxic compounds include nicotine, heavy metals, aldehydes, nitrosamines, polycyclic aromatic hydrocarbons, and more, which are inhaled into the lungs as aerosol particles or free in a gaseous state.Citation112–115 The toxic compounds reduce endogenous antioxidants, increase pro-inflammatory factors concentration in the blood, and increase lipid peroxidation and oxidative stress.Citation112,Citation116–119
When smoking, the oral cavity is the first that comes into direct contact with these toxic compounds making it the most affected part of the body. Toxic compounds found in cigarettes disrupt the oral microbiome’s ecological balance through the formation of unstable bacterial growth in biofilms, increasing saliva acidity and reducing oxygen levels, altering bacterial attachment to mucosal surfaces, inducing antibiotic resistance, and affecting the host’s immune cells.Citation120–122
The salivary microbiome may be influenced by various factors, including host genetics, diet, and environmental factors.Citation123 In an American adult study using 16S rRNA oral wash samples, smokers had a lower abundance of the proteobacteria phyla and Neisseria, Porphyromonas, and Capnocytophaga compared to never smokers.Citation5 Meanwhile, Atopobium, Veillonella, and Streptococcus were enriched.Citation5 The functional analysis from inferred metagenomics revealed that the depleted bacterial genera were involved in carbohydrate, energy metabolism, and xenobiotic metabolism.Citation5 In contrast, the increased bacterial genera were anaerobes, thus supporting the oxygen depletion hypothesis.Citation5
A Puerto Rican study using 16S rRNA, chemokines, and cytokine analysis revealed taxonomic differences between smokers and nonsmokers, which was correlated with enhanced inflammatory responses.Citation124 These factors have been linked with carcinogenesis and inflammation in the oral cavity.Citation124 Proteobacteria was highly enriched in smokers and has been associated with CVD and metabolic conditions.Citation125
A Chinese 16S rRNA study found that alpha diversity differed between smokers and never smokers. Actinomyces and Veillonella were enriched in smokers, which are nitrite-producing bacteria that increase acidity.Citation7 Moryella, Bulleidia, and Moraxella were significantly enriched in the smoking status.Citation7 Acid production pathways were enriched in smokers.Citation7 In a Qatari salivary microbiome study, smoking increased the Bacteroidetes at the phylum level and Prevotella at the genus level. Proteobacteria and Synergistetes at the phylum level, Lactococcus, Corynebacterium, Gemella, Capnocytophaga, and Streptococcus at the genus level were significantly higher in the nonsmokers.Citation7 In another study of low-income African Americans participants, mouth rinse samples were collected and analyzed using 16S rRNA sequencing and showed higher levels of the probiotic genera Bifidobacterium and Lactobacillus, as well as the phylum Actinobacteria in smokers compared to never-smokers. In contrast, the phylum Proteobacteria was depleted in current smokers (Yang et al., 2019).Citation126 A Jordanian study aimed to investigate the salivary microbiome using high throughput next-generation sequencing found Streptococcus, Prevotella, and Veillonella showed significantly elevated levels among smokers at the expense of Neisseria in nonsmokers.Citation127
Tongue samples have also been studied to learn the effects of smoking on the tongue microbiome. Tongue microbiomes of East Asian subjects who were current, former, or never smokers using 16S rRNA amplicon sequencing.Citation128 Their results showed significant differences in microbiome composition and metagenomic functions between current and never smokers but not between former and never smokers.Citation128 Several genera, such as Streptococcus and Megasphaera, showed increase abundance in current smokers, while others, such as Neisseria and Capnocytophaga, were less abundant.Citation128 The same group also conducted metagenomic sequencing on the tongue microbiome and single-nucleotide variant (SNV) profiles and gene content.Citation129 They found that beta diversity between never and current smokers was significantly different, and the SNV profiles of Actinomyces graevenitzii, Megasphaera micronuciformis, Rothia mucilaginosa, Veillonella dispar, and one Veillonella sp. were significantly different between never and current smokers. Furthermore, genes related to the lipopolysaccharide biosynthesis pathway in Veillonella dispar were more frequently present in current smokers.Citation129 Suzuki et al. (2022) used barcoded pyrosequencing analysis to identify bacterial composition in resting saliva and tongue coating; the study demonstrated a significant difference in microbiome richness and diversity between saliva and tongue but not between smokers and nonsmokers.Citation130 Saliva samples of smokers were enriched with the genera Treponema and Selenomonas; however, tongue samples from smokers were enriched with the genera Dialister and Atopobium. The study also found that the genera associated with periodontitis and oral malodor were more abundant in smokers’ saliva and tongue and were positively associated with lifetime exposure to smoking.Citation130
Al-Bataineh et al. (2020) aimed to investigate the oral microbiota of 105 adults using shotgun metagenomics and compared the functional capabilities of the oral microbiome in smokers and nonsmokers. There was an increase in the relative abundance of Veillonella dispar, Leptotrichia spp., and Prevotella pleuritidis in smokers’ buccal swap samples.Citation131 Functional profiling showed that smokers had an enrichment of tricarballylate utilization and lactate racemization, while smokers with high nicotine dependence had an enrichment of xanthosine utilization, p-Aminobenzoyl-Glutamate utilization, and multidrug efflux pump in Campylobacter jejuni biosynthesis modules.Citation131 A Hungarian study also used shotgun analysis and found an increase of Prevotella and Megasphaera genera in smokers, which has been associated with facilitating disease development.Citation6 In contrast, the overall diversity and composition did not differ significantly between smokers and nonsmokers.
It is worth mentioning studies that have categorized their sample size into three groups, current, former, and never smokers, revealed that there is no significant difference in the oral bacteria abundance between former and never smokers, indicating that significant bacteria depletion caused by smoking may be reversed following smoking cessation.Citation5,Citation7 Furthermore, these studies have shown that the oral microbiome of smokers is enriched with anaerobic and facultative anaerobe bacteria while being depleted of aerobic bacteria, indicating smoking alters oxygen availability. A summary of the microbiome profile is presented in .
Table 1. Studies of smoking effects on oral microbiome across diverse populations.
Variability in the oral microbiome among different populations
Research suggests there is a significant variation in the oral microbiome among different populations. Some studies have found no apparent geographical distribution,Citation132,Citation133 while others have reported a significant association between salivary microbiome and geographical location.Citation134,Citation135 For instance, Nasidze et al. (2009) group sequenced 16s rRNA from 10 individuals from 12 different countries and found that the variation in microbiome might be influenced with the distance of each country from the equator.Citation135 However, despite the geographical diversity the study did not show any significant bacterial clustering depending on the geographical location, the bacterial composition of individuals differed from one individual to another, and it is not uniform across all individuals.Citation135 In another study, Li et al. (2014) conducted a study that explored oral microbiome variation from three different groups from different climates and regions (Alaska, Germany, and Africa). They found significant differences in alpha and beta diversity among the different groups, with Germans having the highest alpha diversity and the lowest beta diversity and Africans having the lowest alpha diversity and highest beta diversity. Using UniFrac, network, ANOSIM, and correlation analyses, they found similarities between the Germans’ and Alaskans’ salivary microbiome.Citation136
Similarly, Clarke et al. (2022) found significant differences in oral microbial diversity from four different geographical areas: Thailand, Chile, South Africa, and Barbados. Bacteroidetes and Proteobacteria were the two most abundant phyla, but there were significant differences in their prevalence between countries.Citation137 A recent Qatari study compared the salivary microbiome of the Qatari population to that of various other populations, including Bangladesh, British, Brazilian, Japanese, South Korean, American, and German.Citation134 The data was retrieved from the National Center for Biotechnology Information/Sequence Read Archive (NCBI/SRA) bioprojects.Citation134 The study found differences in microbial composition at the phylum and genus level, with the Qatari population resembling the German population in the abundance of Bacteroidetes, while the other countries had a predominance of the Firmicutes genus.Citation134 A recent study compared the oral and skin microbiome from two regions in Italy and found no significant difference in the oral microbial beta diversity.Citation138 However, the study identified trends in the abundance of specific bacteria depending on age and smoking habits.Citation138 It has been reported the abundant phyla in the salivary microbiome, in general, are Actinobacteria, Bacteroides, Firmicutes, Fusobacteria, Proteobacteria, Spirochetes, and Saccharibacteria.Citation139 Additionally, a study conducted on supragingival plaque samples from children residing in the same geographical location and representing four ethnic groups (Caucasian, Hispanic, Burmese, and African American)Citation140 revealed significant differences in alpha and beta diversity among the ethnic groups, with Burmese children exhibiting the most complex microbial community.Citation140 Burmese and Caucasian children had a higher microbial similarity compared to other ethnic groups.Citation140 Therefore, these findings highlighted the significant variations of the microbiome in supragingival samples among children.Citation140 Similarly, a study conducted in the Kingdom of Saudi Arabia, in Jeddah city, on four different families from different ethnicities (Saudi, Sudanese, Yemeni, and Indian) showed a variation in the abundance of bacteria among the families.Citation141
Furthermore, saliva samples were analyzed from eight different ethnic groups from southern Africa, suggested that ethnicity did not shape the oral microbial profiles of the population, but socioeconomic status could.Citation142 In contrast, a study in Gansu Province, China, where dental plaque samples were sequenced, showed geographic location had a significant influence on the composition of the oral microbiome in the same ethnic group.Citation143
Understanding the interplay between smoking, ethnic-related differences in the oral microbiome, and the pathogenesis of CMS could hold crucial implications for tailored treatment strategies. The oral microbiome’s role as a potential mediator in the association between smoking and CMS warrants further investigation, as it may offer new insights into preventive and therapeutic approaches for individuals at risk. Ultimately, elucidating the complex relationship between the oral microbiome, smoking, and CMS could pave the way for personalized interventions and improved management of cardiometabolic health.
Future direction
The predominant existing studies are correlational rather than causational relationships between oral dysbiosis and CMS. Therefore, there is a need for causation studies to understand the direct impact of oral microbiome on CMS development and progression. Furthermore, to our knowledge, most studies focused on hyperglycemia, hypertension, dyslipidemia, and obesity rather than the syndrome itself. It is crucial to comprehensively study the mechanisms through which smoking induced changes in microbial composition impacts immune, metabolic, and physiological functions. Additionally, exploring variations in the oral microbiome across geographical locations and ethnicities while considering environmental factors such as diet, host genotype, socioeconomic status, lifestyle could lead to valuable insights. This could provide valuable insights into the association between ethnicity, regional location, and disease susceptibility, thus, establishing a foundation for personalized approaches to disease prevention and treatment strategies. Moreover, future research should investigate oral microbial dysbiosis’s impact on the metagenomic content to identify specific microbial taxa and functional pathways influenced by smoking and contributing to CMS. Conducting larger and longitudinal studies can improve statistical power and enhance generalizability, providing crucial insights into the underlying pathogenesis of smoking-related diseases and help in the development of novel targeted therapies.
Conclusion
In conclusion, this narrative review explored the impact of oral microbiome dysbiosis in periodontitis and CMS. Smoking exerts a significant influence on the oral microbiome, leading to dysbiosis that disrupts the ecological balance of the oral microbiome and is linked to the development of oral and systematic diseases. However, the reviewed studies had certain limitations, including variation in sample size, methodologies employed, and inconsistent results from different populations, which made it challenging to establish a direct correlation between smoking-induced dysbiosis and CMS in this review. Nevertheless, it is crucial to acknowledge that smoking has detrimental effects on the oral microbiome and has the potential to contribute to the development of CMS. To gain deeper understanding of the specific effects of smoking on the oral microbiome and its role in disease development, further studies with larger sample sizes with a specific age group is warranted. By addressing these knowledge gaps, we can enhance our comprehension of the complex relationship between smoking, oral microbiome dysbiosis, and the pathogenesis of CMS, paving the way for more effective preventive and therapeutic strategies in the future.
Authors’ contributions
LIM: Manuscript draft writing. ZZZ, FMB: reviewing the manuscript. M.A: conception and reviewing, MA and FMB; Funding acquisition. All authors read and reviewed the final manuscript.
Abbreviations | ||
CMS | = | cardiometabolic syndrome |
T2D | = | type 2 diabetes |
CVD | = | cardiovascular disease |
HMP | = | human microbiome project |
NIH | = | National Institute of Health |
IHMC | = | International Human Microbiota Consortium |
DM | = | diabetes mellitus |
BMI | = | body mass index |
HDL | = | high density lipoprotein |
LDL | = | low density lipoprotein |
IR | = | Insulin resistance |
Disclosure statement
The authors declare that they have no competing interests
Additional information
Funding
References
- Ursell LK, Metcalf JL, Parfrey LW, Knight R. Defining the human microbiome. Nutr Rev. 2012;70 Suppl 1(Suppl 1):S38–S44. doi:10.1111/j.1753-4887.2012.00493.x.
- A review of 10 years of human microbiome research activities at the US National Institutes of Health, Fiscal Years 2007-2016. Microbiome. 2019;7(1):31. doi:10.1186/s40168-019-0620-y.
- Ezzati M, Lopez AD. Regional, disease specific patterns of smoking-attributable mortality in 2000. Tob Control. 2004;13(4):388–395. doi:10.1136/tc.2003.005215.
- Sultan S, Lesloom F. A prospective analysis of the association of smoking with cardiometabolic risk. 2023.
- Wu J, Peters BA, Dominianni C, et al. Cigarette smoking and the oral microbiome in a large study of American adults. ISME J. 2016;10(10):2435–2446. doi:10.1038/ismej.2016.37.
- Wirth R, Maróti G, Mihók R, et al. A case study of salivary microbiome in smokers and non-smokers in Hungary: analysis by shotgun metagenome sequencing. J Oral Microbiol. 2020;12(1):1773067. doi:10.1080/20002297.2020.1773067.
- Jia YJ, Liao Y, He YQ, et al. Association between oral microbiota and cigarette smoking in the Chinese population. Front Cell Infect Microbiol. 2021;11:658203. doi:10.3389/fcimb.2021.658203.
- Bui FQ, Almeida-da-Silva CLC, Huynh B, et al. Association between periodontal pathogens and systemic disease. Biomed J. 2019;42(1):27–35. doi:10.1016/j.bj.2018.12.001.
- Huang Q, Wu X, Zhou X, et al. Association of cigarette smoking with oral bacterial microbiota and cardiometabolic health in Chinese adults. BMC Microbiol. 2023;23(1):346. doi:10.1186/s12866-023-03061-y.
- Dewhirst FE, Chen T, Izard J, et al. The human oral microbiome. J Bacteriol. 2010;192(19):5002–5017. doi:10.1128/JB.00542-10.
- Lamont RJ, Koo H, Hajishengallis G. The oral microbiota: dynamic communities and host interactions. Nat Rev Microbiol. 2018;16(12):745–759. doi:10.1038/s41579-018-0089-x.
- Structure, function and diversity of the healthy human microbiome. Nature. 2012;486(7402):207–214. doi:10.1038/nature11234.
- Deo P, Deshmukh R. Oral microbiome: unveiling the fundamentals. J Oral Maxillofac Pathol. 2019;23(1):122–128. doi:10.4103/jomfp.JOMFP_304_18.
- Lim Y, Totsika M, Morrison M, Punyadeera C. Oral microbiome: a new biomarker reservoir for oral and oropharyngeal cancers. Theranostics. 2017;7(17):4313–4321. doi:10.7150/thno.21804.
- Bacali C, Vulturar R, Buduru S, et al. Oral microbiome: getting to know and befriend neighbors, a biological approach. Biomedicines. 2022;10(3):671. doi:10.3390/biomedicines10030671.
- Kilian M, Chapple ILC, Hannig M, et al. The oral microbiome – an update for oral healthcare professionals. Br Dent J. 2016;221(10):657–666. doi:10.1038/sj.bdj.2016.865.
- Marsh PD. Role of the oral microflora in health. Microb Ecol Health Dis. 2009;12(3):130–137. doi:10.1080/089106000750051800.
- Marsh PD, Takahashi N, Nyvad B. Dental Caries the Disease and Its Clinical Management. 3rd ed. (Fejerskov O, Nyvad B, Kidd E, eds.). John Wiley & Sons; Hoboken, New Jersey, USA; 2016.
- Willey JM, Sherwood L, Woolverton CJ, Prescott LM. Prescott, Harley, and Klein’s Microbiology. 7th ed. McGraw-Hill Higher Education; New York, NY, USA; 2008.
- Gao L, Xu T, Huang G, Jiang S, Gu Y, Chen F. Oral microbiomes: more and more importance in oral cavity and whole body. Protein Cell. 2018;9(5):488–500. doi:10.1007/s13238-018-0548-1.
- Zhang Y, Wang X, Li H, Ni C, Du Z, Yan F. Human oral microbiota and its modulation for oral health. Biomed Pharmacother. 2018;99:883–893. doi:10.1016/j.biopha.2018.01.146.
- Verma D, Garg PK, Dubey AK. Insights into the human oral microbiome. Arch Microbiol. 2018;200(4):525–540. doi:10.1007/s00203-018-1505-3.
- Seymour GJ, Ford PJ, Cullinan MP, Leishman S, Yamazaki K. Relationship between periodontal infections and systemic disease. Clin Microbiol Infect. 2007;13 Suppl 4:3–10. doi:10.1111/j.1469-0691.2007.01798.x.
- Offenbacher S, Jared HL, O’Reilly PG, et al. Potential pathogenic mechanisms of periodontitis-associated pregnancy complications. Ann Periodontol. 1998;3(1):233–250. doi:10.1902/annals.1998.3.1.233.
- Joshipura KJ, Rimm EB, Douglass CW, Trichopoulos D, Ascherio A, Willett WC. Poor oral health and coronary heart disease. J Dent Res. 1996;75(9):1631–1636. doi:10.1177/00220345960750090301.
- Joshipura KJ, Hung HC, Rimm EB, Willett WC, Ascherio A. Periodontal disease, tooth loss, and incidence of ischemic stroke. Stroke. 2003;34(1):47–52. doi:10.1161/01.STR.0000052974.79428.0C.
- Genco RJ, Grossi SG, Ho A, Nishimura F, Murayama Y. A proposed model linking inflammation to obesity, diabetes, and periodontal infections. J Periodontol. 2005;76(11 Suppl):2075–2084. doi:10.1902/jop.2005.76.11-S.2075.
- Beck JD, Offenbacher S. Systemic effects of periodontitis: epidemiology of periodontal disease and cardiovascular disease. J Periodontol. 2005;76(11 Suppl):2089–2100. doi:10.1902/jop.2005.76.11-S.2089.
- Meyle J, Chapple I. Molecular aspects of the pathogenesis of periodontitis. Periodontol 2000. 2015;69(1):7–17. doi:10.1111/prd.12104.
- Ramseier CA, Anerud A, Dulac M, et al. Natural history of periodontitis: disease progression and tooth loss over 40 years. J Clin Periodontol. 2017;44(12):1182–1191. doi:10.1111/jcpe.12782.
- King S, Chow CK, Eberhard J. Oral health and cardiometabolic disease: understanding the relationship. Intern Med J. 2022;52(2):198–205. doi:10.1111/imj.15685.
- Loesche WJ, Grossman NS. Periodontal disease as a specific, albeit chronic, infection: diagnosis and treatment. Clin Microbiol Rev. 2001;14(4):727–752. doi:10.1128/CMR.14.4.727-752.2001.
- Holmstrup P, Damgaard C, Olsen I, et al. Comorbidity of periodontal disease: two sides of the same coin? An introduction for the clinician. J Oral Microbiol. 2017;9(1):1332710. doi:10.1080/20002297.2017.1332710.
- Contaldo M, Itro A, Lajolo C, Gioco G, Inchingolo F, Serpico R. Overview on osteoporosis, periodontitis and oral dysbiosis: the emerging role of oral microbiota. Appl Sci. 2020;10(17):6000. doi:10.3390/app10176000.
- Polak D, Shapira L. An update on the evidence for pathogenic mechanisms that may link periodontitis and diabetes. J Clin Periodontol. 2018;45(2):150–166. doi:10.1111/jcpe.12803.
- Sanz M, Marco del Castillo A, Jepsen S, et al. Periodontitis and cardiovascular diseases: consensus report. J Clin Periodontol. 2020;47(3):268–288. doi:10.1111/jcpe.13189.
- Parvaneh M, Witting PK, Ku J, et al. Periodontitis induces endothelial dysfunction in mice. Sci Rep. 2021;11(1):14993. doi:10.1038/s41598-021-94418-8.
- Hasturk H, Kantarci A. Activation and resolution of periodontal inflammation and its systemic impact. Periodontol 2000. 2015;69(1):255–273. doi:10.1111/prd.12105.
- Rosier BT, Marsh PD, Mira A. Resilience of the oral microbiota in health: mechanisms that prevent dysbiosis. J Dent Res. 2018;97(4):371–380. doi:10.1177/0022034517742139.
- Hajishengallis G. The inflammophilic character of the periodontitis-associated microbiota. Mol Oral Microbiol. 2014;29(6):248–257. doi:10.1111/omi.12065.
- Maekawa T, Krauss JL, Abe T, et al. Porphyromonas gingivalis manipulates complement and TLR signaling to uncouple bacterial clearance from inflammation and promote dysbiosis. Cell Host Microbe. 2014;15(6):768–778. doi:10.1016/j.chom.2014.05.012.
- Duran-Pinedo AE, Chen T, Teles R, et al. Community-wide transcriptome of the oral microbiome in subjects with and without periodontitis. ISME J. 2014;8(8):1659–1672. doi:10.1038/ismej.2014.23.
- Humphrey LL, Fu R, Buckley DI, Freeman M, Helfand M. Periodontal disease and coronary heart disease incidence: a systematic review and meta-analysis. J Gen Intern Med. 2008;23(12):2079–2086. doi:10.1007/s11606-008-0787-6.
- Larvin H, Kang J, Aggarwal VR, Pavitt S, Wu J. Risk of incident cardiovascular disease in people with periodontal disease: a systematic review and meta‐analysis. Clin Exp Dent Res. 2021;7(1):109–122. doi:10.1002/cre2.336.
- Leng WD, Zeng XT, Kwong JSW, Hua XP. Periodontal disease and risk of coronary heart disease: an updated meta-analysis of prospective cohort studies. Int J Cardiol. 2015;201:469–472. doi:10.1016/j.ijcard.2015.07.087.
- Leira Y, Seoane J, Blanco M, et al. Association between periodontitis and ischemic stroke: a systematic review and meta-analysis. Eur J Epidemiol. 2017;32(1):43–53. doi:10.1007/s10654-016-0170-6.
- Muñoz Aguilera E, Suvan J, Buti J, et al. Periodontitis is associated with hypertension: a systematic review and meta-analysis. Cardiovasc Res. 2020;116(1):28–39. doi:10.1093/cvr/cvz201.
- Garde S, Akhter R, Nguyen MA, Chow CK, Eberhard J. Periodontal therapy for improving lipid profiles in patients with type 2 diabetes mellitus: a systematic review and meta-analysis. Int J Mol Sci. 2019;20(15):3826. doi:10.3390/ijms20153826.
- Stöhr J, Barbaresko J, Neuenschwander M, Schlesinger S. Bidirectional association between periodontal disease and diabetes mellitus: a systematic review and meta-analysis of cohort studies. Sci Rep. 2021;11(1):13686. doi:10.1038/s41598-021-93062-6.
- Fontana CR, Grecco C, Bagnato VS, de Freitas LM, Boussios CI, Soukos NS. Molecular analyses of two bacterial sampling methods in ligature-induced periodontitis in rats. Clin Exp Dent Res. 2018;4(1):19–24. doi:10.1002/cre2.98.
- Marotz C, Molinsky R, Martino C, et al. Early microbial markers of periodontal and cardiometabolic diseases in ORIGINS. NPJ Biofilms Microbiomes. 2022;8(1):30. doi:10.1038/s41522-022-00289-w.
- Moon JH, Lee JH, Lee JY. Subgingival microbiome in smokers and non-smokers in Korean chronic periodontitis patients. Mol Oral Microbiol. 2015;30(3):227–241. doi:10.1111/omi.12086.
- Schenkein HA, Loos BG. Inflammatory mechanisms linking periodontal diseases to cardiovascular diseases. J Clin Periodontol. 2013;40 Suppl 14 (0 14):S51–S69. doi:10.1111/jcpe.12060.
- Grundy SM, Cleeman JI, Daniels SR, et al. Diagnosis and management of the metabolic syndrome: An American Heart Association/National Heart, Lung, and Blood Institute scientific statement. Circulation. 2005;112(17):2735–2752. doi:10.1161/CIRCULATIONAHA.105.169404.
- Hossain P, Kawar B, El Nahas M. Obesity and diabetes in the developing world—a growing challenge. N Engl J Med. 2007;356(3):213–215. doi:10.1056/NEJMp068177.
- Wilson PWF, D’Agostino RB, Parise H, Sullivan L, Meigs JB. Metabolic syndrome as a precursor of cardiovascular disease and type 2 diabetes mellitus. Circulation. 2005;112(20):3066–3072. doi:10.1161/CIRCULATIONAHA.105.539528.
- Kelli HM, Kassas I. Cardio metabolic syndrome: a global epidemic. J Diabetes Metab. 2016;6:513. doi:10.4172/2155-6156.1000513.
- Arnlöv J, Ingelsson E, Sundström J, Lind L. Impact of Body Mass Index and the metabolic syndrome on the risk of cardiovascular disease and death in middle-aged men. Circulation. 2010;121(2):230–236. doi:10.1161/CIRCULATIONAHA.109.887521.
- Irace C, Cortese C, Fiaschi E, et al. Components of the metabolic syndrome and carotid atherosclerosis. Hypertension. 2005;45(4):597–601. doi:10.1161/01.HYP.0000158945.52283.c2.
- Misra A, Khurana L. Obesity and the metabolic syndrome in developing countries. J Clin Endocrinol Metab. 2008;93(11 SUPPL. 1):S9–S30. doi:10.1210/jc.2008-1595.
- Grundy SM. Metabolic syndrome pandemic. Arterioscler Thromb Vasc Biol. 2008;28(4):629–636. doi:10.1161/ATVBAHA.107.151092.
- Eyth E, Naik R. Hemoglobin A1C. StatPearls Publishing; St. Petersburg, Florida, United States; 2023.
- Saeedi P, Petersohn I, Salpea P, et al. Global and regional diabetes prevalence estimates for 2019 and projections for 2030 and 2045: Results from the International Diabetes Federation Diabetes Atlas, 9th edition. Diabetes Res Clin Pract. 2019; November; 157:107843. doi:10.1016/j.diabres.2019.107843.
- Lockhart PB, Bolger AF, Papapanou PN, et al. Periodontal disease and atherosclerotic vascular disease: does the evidence support an independent association? Circulation. 2012;125(20):2520–2544. doi:10.1161/CIR.0b013e31825719f3.
- Lalla E, Papapanou PN. Diabetes mellitus and periodontitis: a tale of two common interrelated diseases. Nat Rev Endocrinol. 2011;7(12):738–748. doi:10.1038/nrendo.2011.106.
- Kebschull M, Demmer RT, Papapanou PN. Gum bug, leave my heart alone !”—Epidemiologic and mechanistic evidence linking periodontal infections and atherosclerosis. J Dent Res. 2010;89(9):879–902. doi:10.1177/0022034510375281.
- Ebersole JL, Holt SC, Hansard R, Novak MJ. Microbiologic and immunologic characteristics of periodontal disease in Hispanic Americans with type 2 diabetes. J Periodontol. 2008;79(4):637–646. doi:10.1902/jop.2008.070455.
- Miranda TS, Feres M, Retamal-Valdés B, Perez-Chaparro PJ, Maciel SS, Duarte PM. Influence of glycemic control on the levels of subgingival periodontal pathogens in patients with generalized chronic periodontitis and type 2 diabetes. J Appl Oral Sci. 2017;25(1):82–89. doi:10.1590/1678-77572016-0302.
- Shaalan A, Lee S, Feart C, et al. Alterations in the oral microbiome associated with diabetes, overweight, and dietary components. Front Nutr. 2022;9:914715. doi:10.3389/fnut.2022.914715.
- Pandian DS, Victor DJ, Cholan P, Prakash P, Subramanian S, Shankar SP. Comparative analysis of the red-complex organisms and recently identified periodontal pathogens in the subgingival plaque of diabetic and nondiabetic patients with severe chronic periodontitis. J Indian Soc Periodontol. 2023;27(1):51–56. doi:10.4103/jisp.jisp_136_21.
- Sohail MU, Hedin L, Al-Asmakh M. Dysbiosis of the salivary microbiome is associated with hypertension and correlated with metabolic syndrome biomarkers. Diabetes Metab Syndr Obes. 2021;14:4641–4653. doi:10.2147/DMSO.S325073.
- Lim SS, Vos T, Flaxman AD, et al. A comparative risk assessment of burden of disease and injury attributable to 67 risk factors and risk factor clusters in 21 regions, 1990–2010: a systematic analysis for the Global Burden of Disease Study 2010. Lancet. 2012;380(9859):2224–2260. doi:10.1016/S0140-6736(12)61766-8.
- Iqbal AM, Jamal SF. Essential Hypertension. StatPearls Publishing; St. Petersburg, Florida, United States; 2022.
- Bondonno CP, Liu AH, Croft KD, et al. Antibacterial mouthwash blunts oral nitrate reduction and increases blood pressure in treated hypertensive men and women. Am J Hypertens. 2015;28(5):572–575. doi:10.1093/ajh/hpu192.
- Murugesan S, Al Khodor S. Salivary microbiome and hypertension in the Qatari population. J Transl Med. 2023;21(1):454. doi:10.1186/s12967-023-04247-8.
- Barbadoro P, Ponzio E, Coccia E, et al. Association between hypertension, oral microbiome and salivary nitric oxide: A case-control study. Nitric Oxide. 2021;106:66–71. doi:10.1016/j.niox.2020.11.002.
- Gordon JH, LaMonte MJ, Genco RJ, et al. Is the oral microbiome associated with blood pressure in older women? High Blood Press Cardiovasc Prev. 2019;26(3):217–225. doi:10.1007/s40292-019-00322-8.
- Perry SE, Huckabee M, Tompkins G, Milne T. The association between oral bacteria, the cough reflex and pneumonia in patients with acute stroke and suspected dysphagia. J Oral Rehabil. 2020;47(3):386–394. doi:10.1111/joor.12903.
- Boaden E, Lyons M, Singhrao SK, et al. Oral flora in acute stroke patients: a prospective exploratory observational study. Gerodontology. 2017;34(3):343–356. doi:10.1111/ger.12271.
- Kobayashi R, Ogawa Y, Hashizume-Takizawa T, Kurita-Ochiai T. Oral bacteria affect the gut microbiome and intestinal immunity. Pathog Dis. 2020;78(3) doi:10.1093/femspd/ftaa024.
- Pasini E, Aquilani R, Testa C, et al. Pathogenic gut flora in patients with chronic heart failure. JACC Heart Fail. 2016;4(3):220–227. doi:10.1016/j.jchf.2015.10.009.
- Leskelä J, Pietiäinen M, Safer A, et al. Serum lipopolysaccharide neutralizing capacity in ischemic stroke. PLoS One. 2020;15(2):e0228806. doi:10.1371/journal.pone.0228806.
- Maki KA, Ganesan SM, Meeks B, et al. The role of the oral microbiome in smoking-related cardiovascular risk: a review of the literature exploring mechanisms and pathways. J Transl Med. 2022;20(1):584. doi:10.1186/s12967-022-03785-x.
- Liljestrand JM, Paju S, Pietiäinen M, et al. Immunologic burden links periodontitis to acute coronary syndrome. Atherosclerosis. 2018;268:177–184. doi:10.1016/j.atherosclerosis.2017.12.007.
- Ziebolz D, Rost C, Schmidt J, et al. Periodontal bacterial DNA and their link to human cardiac tissue: findings of a pilot study. Thorac Cardiovasc Surg. 2018;66(1):83–90. doi:10.1055/s-0035-1564689.
- Ziebolz D, Jahn C, Pegel J, et al. Periodontal bacteria DNA findings in human cardiac tissue – is there a link of periodontitis to heart valve disease? Int J Cardiol. 2018;251:74–79. doi:10.1016/j.ijcard.2017.09.001.
- Neves AL, Coelho J, Couto L, Leite-Moreira A, Roncon-Albuquerque R. Metabolic endotoxemia: a molecular link between obesity and cardiovascular risk. J Mol Endocrinol. 2013;51(2):R51–R64. doi:10.1530/JME-13-0079.
- Lund Håheim L, Schwarze PE, Thelle DS, Nafstad P, Rønningen KS, Olsen I. Low levels of antibodies for the oral bacterium Tannerella forsythia predict cardiovascular disease mortality in men with myocardial infarction: a prospective cohort study. Med Hypotheses. 2020;138:109575. doi:10.1016/j.mehy.2020.109575.
- Serra e Silva Filho W, Casarin RCV, Nicolela EL, Passos HM, Sallum AW, Gonçalves RB. Microbial diversity similarities in periodontal pockets and atheromatous plaques of cardiovascular disease patients. PLoS One. 2014;9(10):e109761. doi:10.1371/journal.pone.0109761.
- Mahalakshmi K, Krishnan P, Arumugam SB. Association of periodontopathic anaerobic bacterial co-occurrence to atherosclerosis” – a cross-sectional study. Anaerobe. 2017;44:66–72. doi:10.1016/j.anaerobe.2017.02.003.
- Chalupova M, Skalova A, Hajek T, et al. Bacterial DNA detected on pathologically changed heart valves using 16S rRNA gene amplification. Folia Microbiol (Praha). 2018;63(6):707–711. doi:10.1007/s12223-018-0611-6.
- Moreno S, Parra B, Botero JE, et al. Periodontal microbiota and microorganisms isolated from heart valves in patients undergoing valve replacement surgery in a clinic in Cali, Colombia. Biomedica. 2017;37(4):516–525. doi:10.7705/biomedica.v37i4.3232.
- Oliveira FAF, Forte CPF, Silva Pg de B, et al. Molecular analysis of oral bacteria in heart valve of patients with cardiovascular disease by real-time polymerase chain reaction. Medicine (Baltimore). 2015;94(47):e2067. doi:10.1097/MD.0000000000002067.
- Nakano K, Nemoto H, Nomura R, et al. Detection of oral bacteria in cardiovascular specimens. Oral Microbiol Immunol. 2009;24(1):64–68. doi:10.1111/j.1399-302X.2008.00479.x.
- Koren O, Spor A, Felin J, et al. Human oral, gut, and plaque microbiota in patients with atherosclerosis. Proc Natl Acad Sci USA. 2011;108 Suppl 1 (Suppl 1):4592–4598. doi:10.1073/pnas.1011383107.
- Tam J, Hoffmann T, Fischer S, Bornstein S, Gräßler J, Noack B. Obesity alters composition and diversity of the oral microbiota in patients with type 2 diabetes mellitus independently of glycemic control. PLoS One. 2018;13(10):e0204724. doi:10.1371/journal.pone.0204724.
- de Andrade DR, Silva PA, Colombo AV, Silva‐Boghossian CM. Subgingival microbiota in overweight and obese young adults with no destructive periodontal disease. J Periodontol. 2021;92(10):1410–1419. doi:10.1002/JPER.20-0187.
- Lu M, Xuan S, Wang Z. Oral microbiota: A new view of body health. Food Sci Hum Wellness. 2019;8(1):8–15. doi:10.1016/j.fshw.2018.12.001.
- Kamalul Aripin NF, Idayu Zahid N, Abdul Rahim MA, et al. A review of salivary composition changes induced by fasting and its impact on health. Food Sci Human Wellness. 2023;13(1):50–64. doi:10.26599/FSHW.2022.9250004.
- Sohail MU, Elrayess MA, Al Thani AA, Al-Asmakh M, Yassine HM. Profiling the oral microbiome and plasma biochemistry of obese hyperglycemic subjects in Qatar. Microorganisms. 2019;7(12):645. doi:10.3390/microorganisms7120645.
- Yang Y, Cai Q, Zheng W, et al. Oral microbiome and obesity in a large study of low-income and African-American populations. J Oral Microbiol. 2019;11(1):1650597. doi:10.1080/20002297.2019.1650597.
- Coker MO, Lebeaux RM, Hoen AG, et al. Metagenomic analysis reveals associations between salivary microbiota and body composition in early childhood. Sci Rep. 2022;12(1):13075. doi:10.1038/s41598-022-14668-y.
- Iyer P. Oral cavity is the gateway to the body: role of oral health professionals: a narrative review. J Calif Dent Assoc. 2023;51(1). doi:10.1080/19424396.2023.2193372.
- Sun K, Liu J, Ning G. Active smoking and risk of metabolic syndrome: a meta-analysis of prospective studies. PLoS One. 2012;7(10):e47791. doi:10.1371/journal.pone.0047791.
- Kelishadi R, Noori A, Qorbani M, et al. Are active and passive smoking associated with cardiometabolic risk factors in adolescents? The CASPIAN-III study. Paediatr Int Child Health. 2016;36(3):181–188. doi:10.1179/2046905515Y.0000000039.
- Gastaldelli A, Folli F, Maffei S. Impact of tobacco smoking on lipid metabolism, body weight and cardiometabolic risk. Curr Pharm Des. 2010;16(23):2526–2530. doi:10.2174/138161210792062858.
- Azevedo PS, Polegato BF, Paiva S, et al. The role of glucose metabolism and insulin resistance in cardiac remodelling induced by cigarette smoke exposure. J Cell Mol Med. 2021;25(2):1314–1318. doi:10.1111/jcmm.16053.
- Rigotti NA, Clair C. Managing tobacco use: The neglected cardiovascular disease risk factor. Eur Heart J. 2013;34(42):3259–3267. doi:10.1093/eurheartj/eht352.
- Harris KK, Zopey M, Friedman TC. Erratum: metabolic effects of smoking cessation. Nat Rev Endocrinol. 2016;12(11):684–684. doi:10.1038/nrendo.2016.171.
- Byung JK, Bum SK, Ki CS, Jin HK, Man HL, Jung RP. Association of smoking status, weight change, and incident metabolic syndrome in men: A 3-year follow-up study. Diabetes Care. 2009;32(7):1314–1316. doi:10.2337/dc09-0060.
- Berkowitz L, Pardo-Roa C, Salazar GA, et al. Mucosal exposure to cigarette components induces intestinal inflammation and alters antimicrobial response in mice. Front Immunol. 2019;10:2289. doi:10.3389/fimmu.2019.02289.
- Lee SH, Yun Y, Kim SJ, et al. Association between cigarette smoking status and composition of gut microbiota: population-based cross-sectional study. J Clin Med. 2018;7(9):282. doi:10.3390/jcm7090282.
- Tintinger Gr TA. Harmful interactions of non-essential heavy metals with cells of the innate immune system. J Clin Toxicol. 2011;s3(01). doi:10.4172/2161-0495.S3-005.
- Centers for Disease Control and Prevention [CDC]. How Tobacco smoke causes disease: the biology and behavioral basis for smoking-attributable disease: a report of the surgeon general. 2010
- Ghio AJ, Hilborn ED, Stonehuerner JG, et al. Particulate matter in cigarette smoke alters iron homeostasis to produce a biological effect. Am J Respir Crit Care Med. 2008;178(11):1130–1138. doi:10.1164/rccm.200802-334OC.
- van der Vaart H, Postma DS, Timens W, ten Hacken NHT. Acute effects of cigarette smoke on inflammation and oxidative stress: a review. Thorax. 2004;59(8):713–721. doi:10.1136/thx.2003.012468.
- Roy J, Pallepati P, Bettaieb A, Averill-Bates DA. Acrolein induces apoptosis through the death receptor pathway in A549 lung cells: role of p53. Can J Physiol Pharmacol. 2010;88(3):353–368. doi:10.1139/Y09-134.
- Yoshida M, Tomitori H, Machi Y, et al. Acrolein toxicity: comparison with reactive oxygen species. Biochem Biophys Res Commun. 2009;378(2):313–318. doi:10.1016/j.bbrc.2008.11.054.
- Jamal QMS, Alharbi AH, Dhasmana A, Saxena A, Albejaidi F, Sajid M. Deciphering the influence of cigarette smoke carcinogens on CNS associated biomolecules: a computational synergistic approach. CNS Neurol Disord Drug Targets. 2021;20(6):540–555. doi:10.2174/1871527320666210309142714.
- McEachern EK, Hwang JH, Sladewski KM, et al. Analysis of the effects of cigarette smoke on staphylococcal virulence phenotypes. Infect Immun. 2015;83(6):2443–2452. doi:10.1128/IAI.00303-15.
- Chien J, Hwang JH, Nilaad S, et al. Cigarette smoke exposure promotes virulence of pseudomonas aeruginosa and induces resistance to neutrophil killing. Infect Immun. 2020;88(11) doi:10.1128/IAI.00527-20.
- Brook I. The impact of smoking on oral and nasopharyngeal bacterial flora. J Dent Res. 2011;90(6):704–710. doi:10.1177/0022034510391794.
- Shaw L, Ribeiro ALR, Levine AP, et al. The human salivary microbiome is shaped by shared environment rather than genetics: evidence from a large family of closely related individuals. mBio. 2017;8(5). doi:10.1128/mBio.01237-17.
- Rodríguez-Rabassa M, López P, Rodríguez-Santiago R, et al. Cigarette smoking modulation of saliva microbial composition and cytokine levels. Int J Environ Res Public Health. 2018;15(11):2479. doi:10.3390/ijerph15112479.
- Rizzatti G, Lopetuso LR, Gibiino G, Binda C, Gasbarrini A. Proteobacteria: a common factor in human diseases. Biomed Res Int. 2017;2017:9351507–9351507. doi:10.1155/2017/9351507.
- Yang Y, Zheng W, Cai QY, et al. Cigarette smoking and oral microbiota in low-income and African-American populations. J Epidemiol Community Health. 2019;73(12):1108–1115. doi:10.1136/jech-2019-212474.
- Al-Zyoud W, Hajjo R, Abu-Siniyeh A, Hajjaj S. Salivary microbiome and cigarette smoking: a first of its kind investigation in Jordan. Int J Environ Res Public Health. 2019;17(1):256. doi:10.3390/ijerph17010256.
- Sato N, Kakuta M, Uchino E, et al. The relationship between cigarette smoking and the tongue microbiome in an East Asian population. J Oral Microbiol. 2020;12(1):1742527. doi:10.1080/20002297.2020.1742527.
- Sato N, Kakuta M, Hasegawa T, et al. Metagenomic analysis of bacterial species in tongue microbiome of current and never smokers. NPJ Biofilms Microbiomes. 2020;6(1):11. doi:10.1038/s41522-020-0121-6.
- Suzuki N, Nakano Y, Yoneda M, Hirofuji T, Hanioka T. The effects of cigarette smoking on the salivary and tongue microbiome. Clin Exp Dent Res. 2022;8(1):449–456. doi:10.1002/cre2.489.
- Al Bataineh MT, Dash NR, Elkhazendar M, et al. Revealing oral microbiota composition and functionality associated with heavy cigarette smoking. J Transl Med. 2020;18(1):421. doi:10.1186/s12967-020-02579-3.
- Turnbaugh PJ, Ley RE, Hamady M, Fraser-Liggett CM, Knight R, Gordon JI. The human microbiome project. Nature. 2007;449(7164):804–810. doi:10.1038/nature06244.
- Lynge Pedersen AM, Belstrøm D. The role of natural salivary defences in maintaining a healthy oral microbiota. J Dent. 2019;80 Suppl 1:S3–S12. doi:10.1016/j.jdent.2018.08.010.
- Murugesan S, Al Ahmad SF, Singh P, Saadaoui M, Kumar M, Al Khodor S. Profiling the salivary microbiome of the Qatari population. J Transl Med. 2020;18(1):127. doi:10.1186/s12967-020-02291-2.
- Nasidze I, Li J, Quinque D, Tang K, Stoneking M. Global diversity in the human salivary microbiome. Genome Res. 2009;19(4):636–643. doi:10.1101/gr.084616.108.
- Li J, Quinque D, Horz HP, et al. Comparative analysis of the human saliva microbiome from different climate zones: Alaska, Germany, and Africa. BMC Microbiol. 2014;14(1):316. doi:10.1186/s12866-014-0316-1.
- Clarke T, Brinkac L, Greco C, et al. Sampling from four geographically divergent young female populations demonstrates forensic geolocation potential in microbiomes. Sci Rep. 2022;12(1):18547. doi:10.1038/s41598-022-21779-z.
- Ogbanga N, Nelson A, Ghignone S, et al. The oral microbiome for geographic origin: an Italian study. Forensic Sci Int Genet. 2023;64:102841. doi:10.1016/j.fsigen.2023.102841.
- Neculae E, Gosav EM, Valasciuc E, Dima N, Floria M, Tanase DM. The oral microbiota in valvular heart disease: current knowledge and future directions. Life (Basel). 2023;13(1):182. doi:10.3390/life13010182.
- Premaraj TS, Vella R, Chung J, et al. Ethnic variation of oral microbiota in children. Sci Rep. 2020;10(1):14788. doi:10.1038/s41598-020-71422-y.
- Altayb HN, Chaieb K, Baothman O, Alzahrani FA, Zamzami MA, Almugadam BS. Study of oral microbiota diversity among groups of families originally from different countries. Saudi J Biol Sci. 2022;29(7):103317. doi:10.1016/j.sjbs.2022.103317.
- Araújo V, Fehn AM, Phiri A, Wills J, Rocha J, Gayà-Vidal M. Oral microbiome homogeneity across diverse human groups from southern Africa: first results from southwestern Angola and Zimbabwe. BMC Microbiol. 2023;23(1):226. doi:10.1186/s12866-023-02970-2.
- Ma G, Qiao Y, Shi H, Zhou J, Li Y. Comparison of the oral microbiota structure among people from the same ethnic group living in different environments. Biomed Res Int. 2022;2022:6544497–13. doi:10.1155/2022/6544497.