Abstract
Aim
Treatment options for viral lung infections are currently limited. We aimed to explore the safety and efficacy of inhaled ethanol in an influenza-infection mouse model.
Materials and methods
In a safety and tolerability experiment, 80 healthy female BALB/c mice (20 per group) were exposed to nebulized saline (control) or three concentrations of ethanol (40/60/80% ethanol v/v in water) for 3x30-minute periods, with a two-hour break between exposures. In a separate subsequent experiment, 40 Female BALB/c mice were nasally inoculated with 104.5 plaque-forming units of immediate virulence “Mem71” influenza. Infection was established for 48-h before commencing treatment in 4 groups of 10 mice with either nebulized saline (control) or one of 3 different concentrations of ethanol (40/60/80% ethanol v/v in water) for 3x30-minute periods daily over three consecutive days. In both experiments, mouse behavior, clinical scores, weight change, bronchoalveolar lavage cell viability, cellular composition, and cytokine levels, were assessed 24-h following the final exposure, with viral load also assessed after the second experiment.
Results
In uninfected BALB/c mice, 3x30-minute exposures to nebulized 40%, 60%, and 80% ethanol resulted in no significant differences in mouse weights, cell counts/viability, cytokines, or morphometry measures. In Mem71-influenza infected mice, we observed a dose-dependent reduction in viral load in the 80%-treated group and potentiation of macrophage numbers in the 60%- and 80%-treated groups, with no safety concerns.
Conclusions
Our data provides support for inhaled ethanol as a candidate treatment for respiratory infections.
Introduction
The current management of respiratory viral infections is usually restricted to supportive care, with limited available antiviral and immunomodulatory treatment options.Citation1–5 Ethanol, delivered by inhalation, has several properties that make it a promising candidate treatment for acute respiratory infections.Citation6
Ethanol has well-characterised antimicrobial activity, with concentration-dependent activity against most viruses.Citation7 Ethanol also has potent immunomodulatory properties, described across different contexts in infection, inflammation, allergy, and autoimmunity.Citation6 While negative effects following chronic alcohol misuse are well described,Citation6 short-duration, inhaled ethanol treatment may prove efficacious in the context of the dysregulated immune response seen in severe infections, comparable to other immunomodulatory treatments (e.g. corticosteroids/biologics).Citation3 Finally, ethanol has surfactant-like properties, with demonstrated efficacy in cardiogenic pulmonary edema in humans,Citation8 with this property potentially useful for treating the acute respiratory distress syndrome-related pulmonary edema seen in critical infection.
In general, the primary benefit of the inhaled route of administration is the ability to deliver large doses directly to the target site of infection, while potentially reducing systemic absorption and side effects. Indeed, ethanol delivered by inhalation has been shown in humans to result in significantly lower blood alcohol levels than an equivalent dose delivered orally or intravenously.Citation9,Citation10
While previously published work has supported the potential safety/efficacy of inhaled ethanol in influenza infection when delivered prophylactically before infection,Citation11 ethanol utility as a treatment in established infection has not been explored. We hypothesized that inhaled ethanol would be safe and reduce viral replication in mild established influenza infection in mice.
Materials and methods
Animals
Eight-week-old female BALB/c mice (Animal Resources Center; Murdoch, Western Australia) were housed in individually ventilated cages at the Telethon Kids Institute on non-allergic, dust free bedding (ALPHA-dri TM, Shepherd Specialty Papers, MI, USA). Water and food (Rat and Mouse Cubes, Specialty Feeds, Glen Forrest, Western Australia) were supplied ad libitum. Daily weights and behavior/severity monitoring were performed according to standard operating procedures. All procedures were approved by the Institutional Animal Ethics Committee (AEC # 364) and conformed to National Health and Medical Research Council of Australia guidelines. Mice were monitored during all exposures for signs of distress and clinically scored after each exposure.
Ethanol exposure
For all nebulized exposure studies, individual mice, in groups of ten, were placed in separate compartments of a “pie-box” Perspex exposure chamber. Solutions of 0.9% saline (control) or ethanol at 40%, 60%, and 80% concentrations v/v in water were then nebulized into each chamber using an ultrasonic nebulizer (UltraNeb®, DeVilbiss, Somerset, Pennsylvania). Lignocaine 1% was added to all saline and ethanol solutions to minimize any potential for discomfort during inhalation.
In the baseline safety experiments, 20 healthy naïve mice per treatment group (saline, 40%, 60%, and 80% ethanol) were whole-body exposed for three, 30-minute periods, with a two-hour break in their home cage in between exposures. In the influenza infection model experiments, 10 mice per treatment group were exposed daily for three, 30-minute periods over three consecutive days in the influenza infection model experiments described below.
Influenza infection model
40 mice (n = 10 per exposure group) were inoculated under isoflurane anesthesia by pipetting drops of inoculum onto the nostrils. Mice received 104.5 plaque-forming units (pfu) of immediate virulence “Mem71” (H3N1) diluted in 50 μl phosphate buffered saline. Mem 71 is a reassortment strain of the Influenza A family, consisting of neuraminidase of A/Bellamy/42 and hemagglutinin of A/Memphis/1/71, as previously described.Citation12,Citation13 Mem71 was chosen to assess the impact of inhaled ethanol on established influenza infection in a model of moderate severity where infected mice do not show dramatic weight loss or overt signs of infection.Citation12,Citation13 Infection was established for 48-h (days 1-2) prior to commencing the three days of saline or ethanol exposure (days 3-5) to coincide with the known peak of Mem71 viral replication and cellular immune responses occurring between infection days 4-7.Citation12–14
Sample processing
24-h following their final exposure (day 6), mice were terminally anesthetized via intraperitoneal injection of a solution containing ketamine (40 mg/mL; Troy Laboratories, New South Wales, Australia) and xylazine (2 mg/mL; Troy Laboratories, New South Wales, Australia) at a dose of ∼0.10 mL/10 g body weight. Serum ethanol was not measured as all the inhaled ethanol would have been metabolized and cleared by this timepoint.Citation15
Bronchoalveolar lavage cell and mediator measurements were performed on a random subsample of mice (n = 10 per treatment) in the baseline safety exposure experiment and all mice (n = 10 per treatment) in the influenza infection experiment. Airway morphometry measurements were performed on the other half of mice (n = 10 per treatment) from control and 80% ethanol treatments in the baseline exposure experiment.
Bronchoalveolar lavage fluid (BAL) was collected by washing 0.5 mL of chilled saline (Pfizer Pty Ltd., WA, Australia) in and out of the lungs 3 times. To separate the cells and supernatant, BAL was centrifuged at 350 g for 4 min. A proportion of re-suspended cell pellet was stained with trypan blue and total cell counts obtained using a hemocytometer. Remaining cells were cytospun, stained with Rapid-Stain (Amber Scientific, Traralgon, Victoria, AUS) and differential cell counts conducted using light microscopy. The supernatant was analyzed for interleukin-6 (IL-6) and tumor necrosis factor-alpha (TNF-a) by ELISA as per the manufacturers’ instructions (BD Biosciences, North Ryde, NSW, Australia and R&D Systems, Gymea, NSW, Australia).
For airway morphometry measurements, lungs were inflation fixed at a trans-respiratory pressure of 10 cm H2O by intra-tracheal instillation of 4% formaldehyde prior to removal en bloc. The left lung was embedded in paraffin and 5 µm thick sections were taken from the proximal region. These were stained with Masson’s trichrome, and then analyzed for epithelial thickness, airway smooth muscle mass, and airway lumen diameter using the stereological software (newCAST; Visiopharm, Hørsholm, Denmark) as previously described.Citation16 Airway morphometry measurements were only performed on mice in the control and 80% ethanol treated groups to assess the maximal effect of ethanol treatment.
Viral load
For the viral load assessments, lungs were removed en bloc from mice euthanised as described above. Lungs were immediately flash-frozen in liquid nitrogen and stored at −80 °C until shipped to the School of Chemistry and Molecular Biosciences, University of Queensland for analysis. RNA was extracted and qPCR was performed as previously described.Citation17 Viral copy number was determined using 10-fold dilutions of a plasmid containing the MEM Matrix gene.
Statistics
All statistical analyses were performed in R. Differences between groups were assessed using pairwise t-tests (normal distribution) or Mann-Whitney U-tests (non-normal), with the control group as reference for pairwise group comparisons, or ANOVA for multiple group comparisons. The relationship between variables was assessed using Pearson correlation. A p-value <0.05 was considered statistically significant. P-values were adjusted for multiple comparisons using the Holm method.
Results
Baseline safety
No significant differences in clinical scores or pre-/post-exposure weights were observed between BALB/c mice exposed to saline (control) or three different concentrations (40%,60%,80%) of ethanol (). Ethanol exposed mice displayed unsteady gait for approximately 5 min after exposure, with complete normalization of behavior within 10 min in all animals. BAL and lung samples for morphometric analyses were collected 24-h after exposure and compared between groups. No significant differences in total BAL cell viability (p = 0.71) or lung epithelial layer thickness (p = 0.23), airway smooth muscle mass (p = 0.056), or airway lumen diameter (p = 0.27) were observed (). There was a statistically significant reduction in the number of neutrophils in mice exposed to 80% ethanol as compared to control (median cells/ml 0 vs. 1105, p = 0.044; ). Ethanol exposure had no other significant effects on BAL immune cell composition or inflammatory cytokine levels (all p > 0.05; ).
Table 1. Baseline exposure measurements.
Viral load and disease severity
In mice with established “Mem71” influenza infection, exposure to 3 × 30-minute aerosols of 80% ethanol daily for three days resulted in a significant reduction in influenza viral load compared to controls (mean copy number 1225 vs. 1702, p = 0.042) (). No significant change in viral load was observed after exposures to 40% (mean 1905, p = 0.70) or 60% (mean 1697, p = 0.98) ethanol (). Consistent with this model of mild influenza infection,Citation12 mice in the control group had only a minor reduction in weight after infection with a peak mean weight loss of 6.89% on infection day 6. No significant differences in mouse weight were observed at any timepoint between ethanol- and control-exposed mice (). No significant differences in mouse behavior or clinical scores were observed.
Figure 1. Efficacy of inhaled ethanol in treating established influenza infection. Female BALB/c mice received 104.5 plaque-forming units of immediate virulence “Mem71” influenza. 48-h after infection, mice were exposed to 3 days of 3x30minute exposures to saline (control) or 40%, 60%, or 80% ethanol and analyzed 24-h after the last exposure. (A) Viral load in whole lung tissue was measured by quantitative polymerase chain reaction (n = 10/group). (B) Mice were weighed daily, with weights normalized relative to starting weight (100%). bronchoalveolar lavage samples were collected by washing with chilled saline and then centrifuged to separate the cell pellet and supernatant. (C) Differential counts in the cell pellet were obtained using light microscopy after Rapid-Stain. (D) Interleukin-6 (IL-6) and tumour necrosis factor alpha (TNFα) levels in the supernatant were measured by ELISA. * = holm adjusted p < 0.05 (calculated with t-test in a and Mann-Whitney U-tests in C,D with the control group as reference).
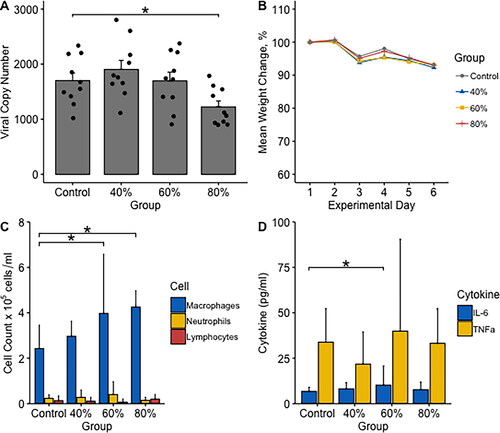
Immune modulation
As expected, influenza infection resulted in large relative increases in measured cell and cytokine levels in all group as compared to their levels measured in uninfected mice (; ). Comparing exposure groups, macrophage numbers were significantly higher in infected mice exposed to 60% (median 4.0x105cells/mL, p = 0.037) and 80% (median 4.3x105cells/mL, p = 0.002) ethanol as compared to infected control mice (median 2.4x105cells/mL). A smaller relative increase in macrophage numbers was also observed in mice exposed to 40% ethanol (median 3.0x105cells/mL, p = 0.165), but this was not statistically significant. No significant differences in neutrophil or lymphocyte numbers were observed between groups ().
We also observed a statistically significant increase in IL-6 in mice exposed to 60% ethanol (median 10.2 pg/mL, p = 0.034) relative to control (median level 6.73 pg/mL) (). Non-statistically significant increases in IL-6 were measured in 40% (median 8.17 pg/mL) and 80% (median 7.70 pg/mL) ethanol-exposed mice relative to control (p > 0.05). The increase in IL-6 was also statistically significant when all ethanol-exposed mice were pooled and compared as a single group to control (median 8.73 pg/mL, p = 0.042). No significant differences in TNFα were observed between ethanol and control mice ().
There was a statistically significant inverse correlation between viral load and macrophage count across all mice, with higher macrophage counts associated with lower viral loads (Pearsons R=-0.31, p = 0.049). No correlations between viral load and IL-6, TNFα, neutrophil, or lymphocyte levels were observed (p > 0.05).
Discussion
Ethanol’s properties make it an interesting candidate therapy for respiratory infections if it can be delivered safely to the airways via inhalation. In this study, we observed no major adverse effects from ethanol exposure across all tested concentrations, with the only statistically significant difference being a reduction in BAL neutrophils after 80% exposure, although in infected mice, neutrophils were present in all groups with no significant differences between ethanol and control groups. These results are consistent with previously published studies modeling the effects of short-term ethanol exposures on the rodent lung, which have also demonstrated no tissue damage on histopathology or large alterations in lung cellularity measured both in BAL or on histology, even after weeks of intermittent exposure.Citation11,Citation18–23 In one study using a rat model of inhaled ethanol exposure with a similar experimental set-up to ours, three fifteen-minute exposures to 65% ethanol vapor repeated for five days resulted in no significant tissue damage as assessed by immunohistochemistry.Citation18 Similar results were observed in C57BL/6J mice exposed to 50% ethanol vapor twice daily for three weeks.Citation11 Other studies of short-term ethanol exposures in rats have also shown no significant changes in BAL cellularity.Citation19,Citation20,Citation23 A lack of effects on airway histology and BAL cellularity has been observed in longer term inhalation studies of rats exposed to 3000 ppm ethanol for six hours per day over two to thirteen weeks.Citation21,Citation22 However, in direct contrast to these results, studies investigating pulmonary effects after chronic oral ethanol exposure have typically reported significant alterations in BAL cellularity with mixed reports of both increases and decreases in macrophage, lymphocyte, and neutrophil cell counts in different studies.Citation24–26 Models of acute intoxication where a single high dose of intravenous/intraperitoneal ethanol is administered have also reported significant alterations in BAL cellularity.Citation27 Collectively, these results highlight both route of administration and duration of exposure as critical factors when determining the safety of ethanol exposure, with our study lending support to the concept that short-term inhaled ethanol may be safe.
In our model of mild established influenza infection, we observed a statistically significant reduction in viral load following three days of exposure to 80% ethanol. This result is consistent with a recent report demonstrating a protective effect in lethal influenza A infection following prophylactic treatment with inhaled 50% ethanol.Citation11 However, the beneficial effect after short-duration treatment in both studies, directly contrasts with the higher viral loads and more severe disease observed in mouse models of chronic oral ethanol misuse,Citation28 highlighting once more the importance of route and duration of exposure in mediating ethanol’s beneficial versus negative effects.
This antiviral effect was dose-dependent with no significant change in viral load following exposures to 40% or 60% ethanol, which differs from the previously described efficacy in vitro at 40% and in vivo at 50% concentrations.Citation7,Citation11 However, direct comparison is challenging given that the experimental set-up, duration of exposure, airway/respiratory physiology, and distribution/deposition dynamics all mean that the concentration of ethanol achieved in the lower airways will be lower than that administered into a dosing chamber and difficult to compare/estimate.Citation29 In our study, we also deliberately chose a model of moderate influenza infection to allow characterization of safety and efficacy outcomes without mice rapidly succumbing to illness. This likely contributed to the relative magnitude of ethanol’s effect in the 80% group, given the modest viral load elevation in the control group.Citation12,Citation13 Future studies aimed at identifying the optimal dosing regimen and minimal effective concentration in more severe infection remain a priority for clinical translation.
One important consideration when interpreting the viral load results is our use of lignocaine to reduce inhalation discomfort, given that lignocaine has previously been shown to have direct anti-viral effects.Citation30 However, lignocaine was included across both ethanol and saline control exposures, making it unlikely to be the primary driver of the observed effect.
Across all tested concentrations, ethanol’s primary immunomodulatory effect appeared to be in potentiating the macrophage-dominant host response seen in control animals. Interestingly, we observed a relative increase in macrophage across all ethanol-exposure groups, but a viral load reduction only in the 80% group, suggesting the concentration required for immunomodulation may be lower than that for antiviral activity. Macrophages have previously been shown to play an essential protective role in the host immune response to influenza,Citation31 and in this model, we observed a weak inverse relationship between higher macrophage numbers and lower viral load. The role of IL-6 in influenza infection is less clear with studies showing mixed associations with both improved and worse outcomes, depending on the model.Citation32 In contrast to the observed dose-dependent relationship between increasing ethanol concentration and increasing macrophage numbers, a more modest effect on cytokine expression was observed with no clear dose-dependent relationship, suggesting ethanol may not be directly regulating their expression in this model. However, while we observed a relative potentiation of macrophages and no-inhibition of TNFα or IL-6 expression, previously-published work has frequently observed suppression of all these factors in different models of ethanol exposure,Citation11,Citation33 which again highlights the complexity of ethanol’s properties likely related to timing, dose, and duration of exposure. Future studies aimed at clarifying the role of other key immune players, including chemokines responsible for monocyte/macrophage recruitment (e.g. monocyte chemoattractant protein-1),Citation34,Citation35 will be important to characterize the full spectrum of ethanol’s antiviral and immunomodulatory properties. However, ethanol has previously been shown to have broad-spectrum immunomodulatory properties and to interact with multiple key signaling molecules/pathways (e.g. nuclear factor kappa B, toll-like receptor, and inflammasome pathways)Citation6,Citation36,Citation37 that are critically involved in the influenza-immune responseCitation38 so clarifying simple mechanistic relationships will be challenging.
One important consideration when interpreting our results, and those previously published, are the limitations of animal models of human inhalation dynamics.Citation39 Significant species differences in airway anatomy and breathing pattern influence aerosol distribution, deposition, and systemic absorption.Citation39 One critical difference is that inhaled ethanol exposure in rodent models tends to lead to high blood levels (0.05-0.5%),Citation11,Citation40 while human studies have consistently shown much lower elevations (<0.01%) and a plateauing effect,Citation9,Citation10 which may influence the translation of safety/efficacy assessments. For example, the transient features of intoxication observed in this model and similar rodent models,Citation11,Citation40 would be of major concern if reproduced in humans, given the significant deleterious biological effects of acute alcohol intoxication.Citation41 However, the reduced blood ethanol levels and lack of intoxication after high-dose ethanol inhalation in humans is reassuring.Citation10
In summary, we have demonstrated the potential efficacy of short duration, high-dose exposures to inhaled aerosolised ethanol on established influenza infection. No significant safety concerns were identified. Our results complement a recent study showing safety and a protective effect of prophylactic inhaled aerosolised ethanol on lethal influenza infection,Citation11 and lend support to inhaled ethanol as a promising and safe candidate treatment. Further studies in other infection models and with optimized dosing regimens would be helpful in extending these results toward potential clinical translation.
Acknowledgments
We would like to thank Drs Kirsty Short and Keng Chew at University of Queensland for measurement of the influenza viral titres.
Disclosure statement
The authors report there are no competing interests to declare.
Data availability statement
The authors confirm that the data supporting the findings of this study are available within the article.
Additional information
Funding
References
- Jones JC, Yen HL, Adams P, Armstrong K, Govorkova EA. Influenza antivirals and their role in pandemic preparedness. Antiviral Res. 2023;210:105499. doi:10.1016/j.antiviral.2022.105499.
- Săndulescu O, Apostolescu CG, Preoțescu LL, Streinu-Cercel A, Săndulescu M. Therapeutic developments for SARS-CoV-2 infection-Molecular mechanisms of action of antivirals and strategies for mitigating resistance in emerging variants in clinical practice. Front Microbiol. 2023;14:1132501. doi:10.3389/fmicb.2023.1132501.
- Stern A, Skalsky K, Avni T, Carrara E, Leibovici L, Paul M. Corticosteroids for pneumonia. Cochrane Database Syst Rev. 2017;12(12):CD007720. doi:10.1002/14651858.CD007720.pub3.
- Youssef J, Novosad SA, Winthrop KL. Infection risk and safety of corticosteroid use. Rheum Dis Clin North Am. 2016;42(1):157–176. doi:10.1016/j.rdc.2015.08.004.
- Albuquerque AM, Tramujas L, Sewanan LR, Williams DR, Brophy JM. Mortality rates among hospitalized patients with COVID-19 infection treated with tocilizumab and corticosteroids: a bayesian reanalysis of a previous meta-analysis. JAMA Netw Open. 2022;5(2):e220548. doi:10.1001/jamanetworkopen.2022.0548.
- Barr T, Helms C, Grant K, Messaoudi I. Opposing effects of alcohol on the immune system. Prog Neuropsychopharmacol Biol Psychiatry. 2016;65:242–251. doi:10.1016/j.pnpbp.2015.09.001.
- Kramer A, Arvand M, Christiansen B, et al. Ethanol is indispensable for virucidal hand antisepsis: memorandum from the alcohol-based hand rub (ABHR) Task Force, WHO Collaborating Centre on Patient Safety, and the Commission for Hospital Hygiene and Infection Prevention (KRINKO), Robert Koch Institute, Berlin, Germany. Antimicrob Resist Infect Control. 2022;11(1):93. doi:10.1186/s13756-022-01134-7.
- Luisada AA, Goldmann MA, Weyl R. Alcohol vapor by inhalation in the treatment of acute pulmonary edema. Circulation. 1952;5(3):363–369. doi:10.1161/01.cir.5.3.363.
- Campbell L, Wilson HK. Blood alcohol concentrations following the inhalation of ethanol vapour under controlled conditions. J Forensic Sci Soc. 1986;26(2):129–135. doi:10.1016/s0015-7368(86)72458-4.
- Hancock DG, Ditcham W, Ferguson E, et al. A phase I clinical trial assessing the safety, tolerability, and pharmacokinetics of inhaled ethanol in humans as a potential treatment for respiratory tract infections. Front Med (Lausanne). 2024;11:1324686. doi:10.3389/fmed.2024.1324686.
- Tamai M, Taba S, Mise T, Yamashita M, Ishikawa H, Shintake T. Ethanol vapor inhalation treatment inhibits lethal respiratory viral infection with Influenza A. J Infect Dis. 2023;228(12):1720–1729. doi:10.1093/infdis/jiad089.
- Gualano RC, Hansen MJ, Vlahos R, et al. Cigarette smoke worsens lung inflammation and impairs resolution of influenza infection in mice. Respir Res. 2008;9(1):53. doi:10.1186/1465-9921-9-53.
- Bozanich EM, Gualano RC, Zosky GR, et al. Acute Influenza A infection induces bronchial hyper-responsiveness in mice. Respir Physiol Neurobiol. 2008;162(3):190–196. doi:10.1016/j.resp.2008.06.011.
- Donovan C, Seow HJ, Bourke JE, Vlahos R. Influenza A virus infection and cigarette smoke impair bronchodilator responsiveness to beta-adrenoceptor agonists in mouse lung. Clin Sci (Lond). 2016;130(10):829–837. doi:10.1042/CS20160093.
- Pastino GM, Asgharian B, Roberts K, Medinsky MA, Bond JA. A comparison of physiologically based pharmacokinetic model predictions and experimental data for inhaled ethanol in male and female B6C3F1 mice, F344 rats, and humans. Toxicol Appl Pharmacol. 1997;145(1):147–157. doi:10.1006/taap.1997.8161.
- Larcombe AN, Janka MA, Mullins BJ, Berry LJ, Bredin A, Franklin PJ. The effects of electronic cigarette aerosol exposure on inflammation and lung function in mice. Am J Physiol Lung Cell Mol Physiol. 2017;313(1):L67–L79. doi:10.1152/ajplung.00203.2016.
- Short KR, Reading PC, Brown LE, et al. Influenza-induced inflammation drives pneumococcal otitis media. Infect Immun. 2013;81(3):645–652. doi:10.1128/IAI.01278-12.
- Castro-Balado A, Mondelo-Garcia C, Barbosa-Pereira L, et al. Development and Characterization of Inhaled Ethanol as a Novel Pharmacological Strategy Currently Evaluated in a Phase II Clinical Trial for Early-Stage SARS-CoV-2 Infection. Pharmaceutics. 2021;13(3):342. doi:10.3390/pharmaceutics13030342.
- Choi WS, Murthy GG, Edwards DA, Langer R, Klibanov AM. Inhalation delivery of proteins from ethanol suspensions. Proc Natl Acad Sci U S A. 2001;98(20):11103–11107. doi:10.1073/pnas.201413798.
- Montharu J, Le Guellec S, Kittel B, et al. Evaluation of lung tolerance of ethanol, propylene glycol, and sorbitan monooleate as solvents in medical aerosols. J Aerosol Med Pulm Drug Deliv. 2010;23(1):41–46. doi:10.1089/jamp.2008.0740.
- Scarino A, Noël A, Renzi PM, et al. Impact of emerging pollutants on pulmonary inflammation in asthmatic rats: ethanol vapors and agglomerated TiO2 nanoparticles. Inhal Toxicol. 2012;24(8):528–538. doi:10.3109/08958378.2012.696741.
- Scarino A, Tardif R, Charbonneau M. Influence of ALDH2 polymorphism on ethanol kinetics and pulmonary effects in male and female rats exposed to ethanol vapors. Inhal Toxicol. 2009;21(3):193–199. doi:10.1080/08958370802372815.
- Sharma S, Lee J, Gao P, Steele VE. Toxicity profile of solvents by aspiration approach for topical agent delivery to respiratory tract epithelium. Int J Toxicol. 2011;30(3):358–366. doi:10.1177/1091581810396729.
- Elliott MK, Sisson JH, Wyatt TA. Effects of cigarette smoke and alcohol on ciliated tracheal epithelium and inflammatory cell recruitment. Am J Respir Cell Mol Biol. 2007;36(4):452–459. doi:10.1165/rcmb.2005-0440OC.
- Mouton AJ, Maxi JK, Souza-Smith F, et al. Alcohol Vapor Inhalation as a Model of Alcohol-Induced Organ Disease. Alcohol Clin Exp Res. 2016;40(8):1671–1678. doi:10.1111/acer.13133.
- Poole LG, Beier JI, Torres-Gonzales E, et al. Chronic + binge alcohol exposure promotes inflammation and alters airway mechanics in the lung. Alcohol. 2019;80:53–63. doi:10.1016/j.alcohol.2018.10.008.
- Aytacoglu BN, Calikoglu M, Tamer L, et al. Alcohol-induced lung damage and increased oxidative stress. Respiration. 2006;73(1):100–104. doi:10.1159/000088680.
- Meyerholz DK, Edsen-Moore M, McGill J, Coleman RA, Cook RT, Legge KL. Chronic alcohol consumption increases the severity of murine influenza virus infections. J Immunol. 2008;181(1):641–648. doi:10.4049/jimmunol.181.1.641.
- George SC, Hlastala MP, Souders JE, Babb AL. Gas exchange in the airways. J Aerosol Med. 1996;9(1):25–33. doi:10.1089/jam.1996.9.25.
- Liu X, Zheng F, Tian L, et al. Lidocaine inhibits influenza a virus replication by up-regulating IFNalpha4 via TBK1-IRF7 and JNK-AP1 signaling pathways. Int Immunopharmacol. 2023;115:109706. doi:10.1016/j.intimp.2023.109706.
- Tate MD, Pickett DL, van Rooijen N, Brooks AG, Reading PC. Critical role of airway macrophages in modulating disease severity during influenza virus infection of mice. J Virol. 2010;84(15):7569–7580. doi:10.1128/JVI.00291-10.
- Dienz O, Rud JG, Eaton SM, et al. Essential role of IL-6 in protection against H1N1 influenza virus by promoting neutrophil survival in the lung. Mucosal Immunol. 2012;5(3):258–266. doi:10.1038/mi.2012.2.
- McCaskill ML, Romberger DJ, DeVasure J, et al. Alcohol exposure alters mouse lung inflammation in response to inhaled dust. Nutrients. 2012;4(7):695–710. doi:10.3390/nu4070695.
- Li H, Wang A, Zhang Y, Wei F. Diverse roles of lung macrophages in the immune response to influenza A virus. Front Microbiol. 2023;14:1260543. doi:10.3389/fmicb.2023.1260543.
- Boylen CE, Sly PD, Zosky GR, Larcombe AN. Physiological and inflammatory responses in an anthropomorphically relevant model of acute diesel exhaust particle exposure are sex and dose-dependent. Inhal Toxicol. 2011;23(14):906–917. doi:10.3109/08958378.2011.625454.
- Goral J, Kovacs EJ. In vivo ethanol exposure down-regulates TLR2-, TLR4-, and TLR9-mediated macrophage inflammatory response by limiting p38 and ERK1/2 activation. J Immunol. 2005;174(1):456–463. doi:10.4049/jimmunol.174.1.456.
- Nurmi K, Virkanen J, Rajamäki K, Niemi K, Kovanen PT, Eklund KK. Ethanol inhibits activation of NLRP3 and AIM2 inflammasomes in human macrophages–a novel anti-inflammatory action of alcohol. PLoS One. 2013;8(11):e78537. doi:10.1371/journal.pone.0078537.
- Mifsud EJ, Kuba M, Barr IG. Innate Immune Responses to Influenza Virus Infections in the Upper Respiratory Tract. Viruses. 2021;13(10):2090. doi:10.3390/v13102090.
- Schlesinger RB. Comparative deposition of inhaled aerosols in experimental animals and humans: a review. J Toxicol Environ Health. 1985;15(2):197–214. doi:10.1080/15287398509530647.
- Glover EJ, Khan F, Clayton-Stiglbauer K, Chandler LJ. Impact of sex, strain, and age on blood ethanol concentration and behavioral signs of intoxication during ethanol vapor exposure. Neuropharmacology. 2021;184:108393. doi:10.1016/j.neuropharm.2020.108393.
- Vonghia L, Leggio L, Ferrulli A, Bertini M, Gasbarrini G, Addolorato G, Alcoholism Treatment Study Group. Acute alcohol intoxication. Eur J Intern Med. 2008;19(8):561–567. doi:10.1016/j.ejim.2007.06.033.