Abstract
Purpose: To evaluate the usefulness of a new 10B-compound (TX-2100) as a 10B-carrier in boron neutron capture therapy (BNCT), compared with the simultaneous use of its component drugs, sodium borocapate-10B (BSH) and 3-amino-2-quinoxalinecarbonitrile 1,4-dioxide (TX-402). Further, the usefulness of mild temperature hyperthermia (MTH, 40°C, 30 min) combined with TX-2100 was also examined compared with MTH combined with the concurrent administration with its component drugs.
Materials and methods: TX-2100 is a hybrid compound that has both a hypoxic cytotoxin unit (TX-402) and a thermal neutron-sensitizing unit (BSH). TX-2100 or both TX-402 plus BSH in combination with MTH or not was administered to SCC VII tumour-bearing mice intra-peritoneally. Then, the 10B concentrations in the tumours and normal tissues were measured by γ-ray spectrometry. Meanwhile, SCC VII tumour-bearing mice were continuously given 5-bromo-2′-deoxyuridine (BrdU) to label all proliferating (P) cells in the tumours, then treated with TX-2100, TX-402 plus BSH or BSH only, in the same manner as in the biodistribution experiments, either with or without MTH. Right after thermal neutron irradiation during which intra-tumour 10B concentrations remained at similar levels, the tumours were excised, minced and trypsinized. The tumour cell suspensions thus obtained were incubated with cytochalasin-B (a cytokinesis blocker) and the micronucleus (MN) frequency in cells without BrdU labelling (=quiescent (Q) cells) was determined using immunofluorescence staining for BrdU. Meanwhile, the MN frequency in the total (P + Q) tumour cell population was determined from the tumours that were not pre-treated with BrdU. The clonogenic cell survival was also determined in mice given no BrdU.
Results: 10B biodistribution analyses in tumours, brain, skin, muscles, blood and liver indicated that the administration of TX-2100 plus MTH is most favourable for concentrating a sufficient amount of 10B in tumours and maintaining a high enough 10B concentration during irradiation. In addition, MTH had a stronger sensitizing effect when combined with TX-2100 than with the concurrent administration of its components TX-402 and BSH on both the total and Q cell populations in solid tumours.
Conclusion: MTH was very effective in combination with the newly-developed TX-2100. The sensitizing effect in combination with MTH should be examined when new 10B-carriers are designed.
Introduction
A neutron capture reaction in boron (10B(n,α)7Li) is, in principle, very effective in destroying tumours, providing that a sufficient amount of 10B can be accumulated in the target tumour and a sufficient number of very-low-energy thermal neutrons can be delivered there. The two particles generated in this reaction carry a high linear energy transfer (LET) and have a range of roughly the diameter of one or two tumour cells. Thus, most of the ionizing energy imparted to tissue is localized to 10B-loaded cells, resulting in high relative biological effectiveness (RBE) when a sufficient quantity of 10B can be distributed in the tissue Citation[1]. However, at present, it is difficult to deliver a therapeutic amount of 10B from currently used 10B-carriers throughout the target tumour, especially into the intra-tumour hypoxic cells with low uptake capacities Citation[1],Citation[2]. Meanwhile, the development of bioreductive agents that are particularly toxic to hypoxic cells is considered a promising approach to solving the problem of tumour hypoxia in cancer therapy. Thus, to confer on a conventional 10B-carrier this hypoxia-directional characteristic, a hybrid was newly designed which constituted a hypoxic cytotoxin moiety and the clinically used sodium borocaptate-10B (BSH) attached via a carbon chain linker as a pharmacokinetic controlling unit. 3-amino-2-quinoxalinecarbonitrile 1,4-dioxide (TX-402) was employed as a hypoxic cytotoxin since TX-402 was shown to be a promising hypoxic cytotoxin as well as tirapazamine, a lead compound as a bioreductive hypoxic cytotoxin Citation[3].
Mild temperature hyperthermia (MTH) was reported to increase the response of tumours to radiation by improving oxygenation through an increase in tumour blood flow Citation[4]. In addition, MTH was also shown to enhance the tumour response, especially of the intra-tumour quiescent (Q) cell population, to hypoxic cytotoxin Citation[5] and the boron neutron capture reaction Citation[6].
In a previous report, the usefulness of TX-2100 as a 10B-carrier in boron neutron capture therapy (BNCT) was demonstrated Citation[7]. However, the use of TX-2100 has not been compared with the simultaneous use of its component drugs, TX-402 and BSH. Thus, the usefulness was evaluated of TX-2100 as a newly designed 10B-carrier in BNCT, by comparing its effect with that of TX-402 plus BSH. Further, the usefulness of MTH in combination with TX-2100 was also examined, again on comparison with the concurrent administration of the component drugs of TX-2100, in terms of the biodistribution of 10B and radio-sensitizing effects under thermal neutron beam irradiation in both total (=proliferating (P) + quiescent (Q)) and Q-cell populations in solid tumours employing the newly developed method for selectively detecting the response of intra-tumour Q-cell populations in vivo Citation[2].
Materials and methods
Mice and tumours
SCC VII squamous cell carcinoma (Department of Radiology, Kyoto University) derived from C3H/He mice was maintained in vitro in Eagle's minimum essential medium supplemented with 12.5% foetal bovine serum. Cells were collected from exponentially growing cultures, and 1.0 × 105 cells of each tumour were inoculated subcutaneously into the left hind legs of 8–11-week-old syngeneic female C3H/He mice ( Japan Animal Co., Ltd., Osaka, Japan). Fourteen days after inoculation, each tumour had reached ∼1 cm in diameter. At treatment, the body weight of the tumour-bearing mice was 22.1 (19.8–24.4) g (mean 95% confidence limit). Mice were handled according to the Recommendations for Handling of Laboratory Animals for Biomedical Research, compiled by the Committee on Safety and Ethical Handling Regulations for Laboratory Animal Experiments, Kyoto University. All experimental procedures mentioned here were in accordance with institutional guidelines for the care and use of laboratory animals in research.
Compounds
The new 10B-compound (TX-2100) was synthesized from the hypoxia-specific cytotoxic bioreductive agent TX-402 Citation[8] and BSH (). The synthesis will be described in detail elsewhere, but briefly; 3-chloro-2-quinoxalinecarbonitrile-1,4-di-N-oxide was condensed with propyl alcohol amine in the presence of K2CO3 to obtain the hydroxyalkylamino derivative. This derivative was treated with bromoacetyl isocyanate and then conjugated with disodium (2-cyanoethyl)-thio-undecahydro-closo-dodecaborate (2-) to yield a cyanoethyl sulphonium compound, TX-2100. This is an essentially TX-402-BSH conjugate, that is a hybrid compound that has both a hypoxic cytotoxin unit and a thermal neutron-sensitizing unit, BSH. The structure of this compound was elucidated based on physical and spectral data.
Biodistribution experiment
TX-2100, dissolved in physiological saline, was administered to the tumour-bearing mice intra-peritoneally (0.75 µmole g−1 body weight of mice). As a control, TX-402 and BSH, both dissolved in physiological saline, were also intra-peritoneally administered simultaneously at the same dose. In addition, MTH (40°C, 30 min) was performed right after the drug administration or not. At various time points after the administration, mice were sacrificed and tumours were excised. Additionally, brain, blood, liver, muscle and skin samples were also collected. The blood samples were collected through heart puncture. The 10B concentrations in these tissues were measured by prompt γ-ray spectrometry using a thermal neutron guide tube installed at the Kyoto University Reactor (KUR) Citation[9].
Concerning MTH, the tumours grown in the left hind legs of mice were heated at 40°C for 30 min by immersing the tumour-bearing foot in a water bath. The mouse was held in a specially constructed device with the tail and right leg firmly fixed with an adhesive tape. The left tumour-bearing leg was pulled down by a special sinker (∼45 g) which was affixed to the skin of the toe with Superglue (Arone-arufa, Konishi Co., Osaka, Japan). The mice were then placed on a circulating water bath maintained at the desired temperature. The mice were air-cooled during the heat treatment Citation[10]. Temperatures at the tumour centre equilibrated within 3–4 min after immersion in the water bath and remained 0.2–0.3°C below the bath's temperature. The water bath's temperature was maintained at 0.3°C above the desired tumour temperature.
Labelling with 5-bromo-2′-deoxyuridine (BrdU)
Nine days after the tumour cell inoculation, mini-osmotic pumps (Durect Corporation, Cupertino, CA) containing BrdU dissolved in physiological saline (200–250 mg ml−1) were implanted subcutaneously, to label all P cells, for 5 days. Administration of BrdU did not change the tumour growth rate. The tumours were 1 cm in diameter on treatment. The labelling index after continuous labelling with BrdU was 55.3 (50.8–59.8)% and reached a plateau level at these stages. Therefore, tumour cells were regarded not incorporating BrdU after continuous labelling as Q-cells.
Irradiation
Based on the data from the biodistribution experiment, to obtain similar intra-tumour 10B concentrations during exposure to neutrons, irradiation was started from 60 min and 30 min after the intra-peritoneal administration of TX-2100 at a dose of 0.75 µmole g−1 and both TX-402 and BSH and BSH only at a dose of 1.2 µmole g−1, respectively, whether combined with or without MTH.
For irradiation of the tumours implanted into the left hind legs of mice, a device made of acrylic resin and capable of holding 16 mice was used and the tumour-bearing mice were irradiated with a reactor thermal neutron beam after being fixed in position with adhesive tape. When combined treatment, MTH was carried out right after the drug administration in the same manner as in the biodistribution experiment.
Thermal neutron irradiation was performed using a reactor neutron beam with a cadmium (Cd) ratio of 160. The neutron fluence was measured from the radioactivation of gold foil at both the front and back of the tumours. The tumours were small and located just beneath the surface. Therefore, the neutron fluence was assumed to decrease linearly from the front to back of the tumours. Thus, the average neutron fluence determined from the values measured at the front and back were used. Contaminating γ-ray, including secondary γ-ray, doses were measured with a thermoluminescence dosimeter powder at the back of the tumours. For the estimation of neutron energy spectra, eight kinds of activation foil and 14 kinds of nuclear reaction were used. The absorbed dose was calculated using the flux-to-dose conversion factor Citation[11]. The tumours contained in weight percentage H (10.7%), C (12.1%), N (2%), O (71.4%) and others (3.8%) Citation[12]. The average neutron flux (n cm−2 s−1) and Kerma rate (cGy h−1) of the beam were 2.0 × 109 and 96 for the thermal neutron range (less than 0.6 eV), 2.8 × 107 and 1.03 for the epithermal neutron range (0.6–10 keV), and 6.6 × 106 and 28.4 for the fast neutron range (more than 10 keV), respectively. The contaminating γ-ray dose rate was 120 cGy h−1.
Each irradiation group also included mice that were not pre-treated with BrdU.
Immunofluorescence staining of BrdU-labelled cells and micronucleus assay
Right after irradiation of the implanted tumours, tumours were excised from the mice given BrdU, minced and trypsinized. Tumour cell suspensions thus obtained were incubated for 72 h in tissue culture dishes containing complete medium and 1.0 µg ml−1 of cytochalasin-B to inhibit cytokinesis while allowing nuclear division and the cultures were then trypsinized and cell suspensions were fixed. After the centrifugation of fixed cell suspensions, the cell pellet was resuspended with cold Carnoy's fixative (ethanol:acetic acid = 3:1 in volume). The suspension was then placed on a glass microscope slide and the sample was dried at room temperature. The slides were treated with 2 M hydrochloric acid for 60 min at room temperature to dissociate the histones and partially denature the DNA. The slides were then immersed in borax-borate buffer (pH 8.5) to neutralize the acid. BrdU-labelled tumour cells were detected by indirect immunofluorescence staining using monoclonal anti-BrdU antibody (Becton Dickinson, San Jose, CA) and fluorescein isothiocyanate (FITC)-conjugated anti-mouse IgG antibody (Sigma, St. Louis, MO). To observe the double staining of tumour cells with green-emitting FITC and red-emitting propidium iodide (PI), cells on the slides were treated with PI (2 µg ml−1 in phosphate-buffered saline (PBS)) and monitored under a fluorescence microscope.
The micronucleus (MN) frequency in cells not labelled with BrdU could be examined by counting the micronuclei in the binuclear cells that showed only red fluorescence. The MN frequency was defined as the ratio of the number of micronuclei in the binuclear cells to the total number of binuclear cells observed Citation[2]. More than 400 binuclear cells were counted to determine the MN frequency. The ratios obtained in tumours not pre-treated with BrdU indicated the MN frequency at all phases in the total (P + Q) tumour cell population.
Clonogenic cell survival assay
The clonogenic cell survival assay was also performed in the mice given no BrdU using an in vivo–in vitro assay method. Tumours were disaggregated by stirring for 20 min at 37° in PBS containing 0.05% trypsin and 0.02% ethylenediamine tetraacetic acid. The cell yield was 4.5 (3.4–5.6) × 107 g−1 tumour weight. Appropriate numbers of viable tumour cells from the single cell suspension were plated on 60 or 100 mm tissue culture dishes and, 12 days later, colonies were fixed with ethanol, stained with Giemsa and counted. For the tumours that received no irradiation, the plating efficiencies for total tumour cells and the MN frequencies for total and Q-cells are shown in .
Table I. Plating efficiencies and micro-nucleus frequencies at 0 Gy.
Three mice were used to assess each set of conditions and each experiment was repeated twice. To examine the differences between pairs of values, Student's t-test was used when variances of the two groups could be assumed to be equal, otherwise the Welch t-test was used. P-values were from two-sided tests.
Results
Overall, the MN frequencies for Q-cells were significantly larger than those for total cells (p < 0.05) (). TX-2100 only and both TX402 and BSH treatments induced a significantly lower plating efficiency and higher MN frequency in both the total and Q-cell populations than absolutely no drug and BSH only treatments (p < 0.05). BSH treatment caused no significant changes in the plating efficiency or MN frequency of either the total or Q-cell population, compared with absolutely no drug treatment. In the drug administration, BSH, TX-2100 or TX-402 plus BSH, in combination with MTH induced a significant increase in the MN frequency for Q-cells, but not for the total cell populations (p < 0.05).
shows the time course of change in the concentration of 10B in solid tumours, brain, blood, liver, muscle and skin. Although concentrations were actually low in the brain, those in the muscle and skin were similar to those in the tumours and the concentrations of 10B in the blood and liver were much higher than those in the tumours. TX-2100 combined with MTH was most effective in maintaining high 10B concentrations in tumours and did not cause very high 10B concentrations in the brain, blood, liver, muscle or skin, compared with any other drug administration manner.
Figure 2. The time course of changes in the 10B concentrations in solid tumours, brain, blood collected from heart, liver, muscle and skin of tumour-bearing mice. Bars represent standard deviations. TX-2100; a newly synthesized hypoxic cytotoxin-sodium borocaptate-10B conjugate, TX-402; 3-amino-2-quinoxalinecarbonitrile 1,4-dioxide, BSH; sodium borocaptate-10B, MTH; mild temperature hyperthermia (40°C, 30 min).
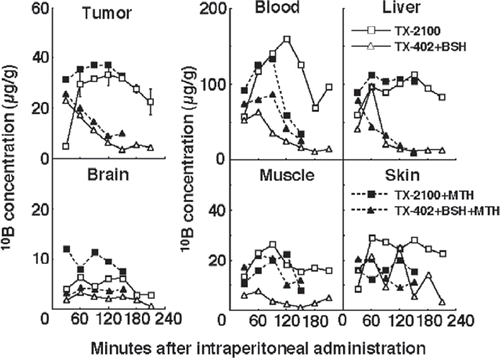
shows the clonogenic cell survival curves after irradiation with a thermal neutron beam following the administration of no 10B-carrier, BSH, both TX-402 and BSH or TX-2100 with or without MTH. TX-2100 plus MTH had a greater radio-sensitizing effect than any other administration condition. The left and right panels of show the normalized MN frequencies for thermal neutron irradiation as a function of the physically absorbed radiation dose in the total and Q-tumour cell populations, respectively. When the 10B-carrier was employed, even if no radiation was given, MN frequencies were higher than when no 10B-carrier was administered, because of the slight genotoxicity of the drug (). Therefore, for background correction, the normalized MN frequency was used to exclude the effects of the genotoxicity of the 10B-carrier. The normalized frequency is the frequency in the irradiated tumours minus the frequency in the non-irradiated tumours. All MN frequencies were actual values induced under each set of conditions. Namely, they included the values due to contaminating fast neutrons and γ-rays in the neutron beam. On any treatment condition, the normalized MN frequencies for Q-cells were significantly smaller than those for total cells (p < 0.05). The 10B concentrations in tumours were the average 10B concentrations determined from the values measured at the start and end point of neutron irradiation. The 10B concentrations in tumours for the BSH, both TX-402 and BSH, and TX-2100 administration groups were 32.1 (25.8–38.4) µg g−1, 31.9 (25.8–38.0) µg g−1 and 31.7 (30.6–32.8) µg g−1, respectively. The 10B concentrations in tumours heated by MTH for the BSH, both TX-402 and BSH, and TX-2100 administration groups were 33.1 (26.8–39.4) µg g−1, 33.0 (26.9–39.1) µg g−1 and 32.9 (31.8–34.0) µg g−1, respectively.
Figure 3. The clonogenic cell survival curves after irradiation with a thermal neutron beam following the administration of no 10B-carrier, BSH (sodium borocaptate-10B), both TX-402 (3-amino-2-quinoxalinecarbonitrile 1,4-dioxide) and BSH or TX-2100 with or without mild temperature hyperthermia (MTH, 40°C, 30 min). Bars represent standard deviations.
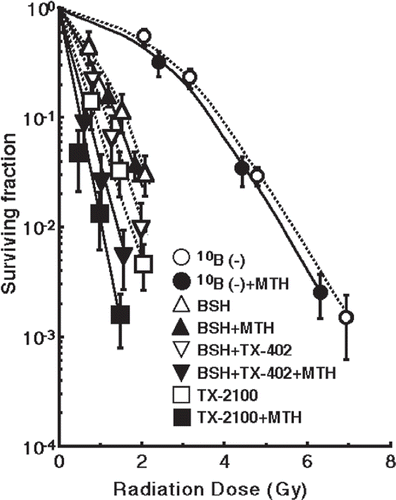
Figure 4. The normalized MN frequencies for thermal neutron irradiation as a function of the physically absorbed radiation dose in total and Q-tumour cells, respectively. Irradiation was performed following administration of no 10B-carrier, BSH (sodium borocaptate-10B), both TX-402 (3-amino-2-quinoxalinecarbonitrile1, 4-dioxide) and BSH or TX-2100 with or without mild temperature hyperthermia (MTH, 40°C, 30 min). Bars represent standard deviations.
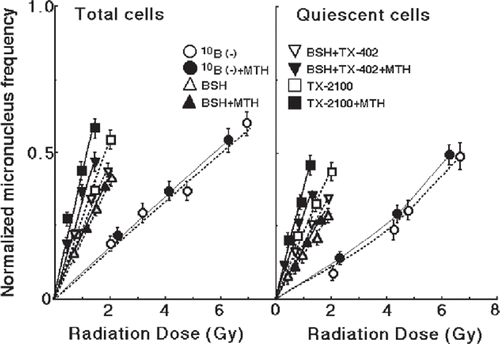
To assess the effect of the 10B-carrier on the surviving fractions in total cells and the MN frequencies in total and Q-cells, the enhancement ratio (ER) was calculated using the data given in and (). The ER values were significantly larger for TX-2100 than BSH and TX-402 plus BSH, not only in total tumour cells but also in Q-cells (p < 0.05), whether combined with MTH or not. Further, there was a tendency for the sensitivity of total cells to be enhanced slightly more markedly than that of Q-cells for drug only administration without MTH. In contrast, the combination with MTH enhanced the sensitivity of Q-cells more markedly than that of total cells, significantly on the use of TX-2100 and both TX-402 and BSH (p < 0.05) and slightly on the use of BSH.
Table II. The effects* of drugs on each end-point.
To assess the effect of MTH on the surviving fractions in total cells and the MN frequencies in total and Q-cells, the enhancement ratio (ER) was calculated using the data given in and (). The ER values for TX-2100 and both TX-402 and BSH were significantly larger than those for BSH not only in total tumour cells but also in Q-cells (p < 0.05). The values for TX-2100 were slightly larger than those for both TX-402 and BSH. In addition, the values for Q-cells were slightly larger than those for total cells on any treatment condition.
Table III. The effects* of mild temperature hyperthermia on each end-point.
Discussion
The presence of hypoxic cells in solid tumours is a serious problem in the treatment of cancer because such cells show resistance to radiotherapy and conventional chemotherapy, as well as an increased pre-disposition to metastasis Citation[12]. In contrast to normal tissues, most solid tumours have regions of low oxygen (hypoxia), low pH and low levels of glucose Citation[13]. Therefore, the hypoxic micro-environment in solid tumours represents an attractive target for tumour-selective cancer therapy Citation[13]. The development of bioreductive agents that are selectively toxic to hypoxic cells is one approach to solving the problem of tumour hypoxia in cancer therapy. 1,2,4-Benzotriazine 1,4-di-N-oxides form a novel class of anti-cancer agents that are activated bioreductively in the hypoxic cells found in solid tumours to kill the oxygen-deficient tumour cells selectively as hypoxic cytotoxins. In a previous report, hypoxic cytotoxins, such as the benzotriazine oxide derivative TPZ and quinoxaline oxide TX-402 (3-amino-2-quinoxalinecarbonitrile 1,4-dioxide) (), were shown to induce p53-independent hypoxic cytotoxicity in tumour cells by promoting apoptosis and inhibiting angiogenesis in vitro Citation[3].
In BNCT, when a therapeutic amount of 10B can be distributed throughout the target tumour, two particles generated through the neutron capture reaction in boron (10B(n,α)7Li) carry a high LET if a sufficient number of very-low-energy thermal neutrons can be delivered. Thus, they can damage hypoxic tumour cells very efficiently as well as aerobic tumour cells Citation[1]. However, it is difficult to distribute a therapeutically effective amount of 10B homogeneously throughout the target tumour including hypoxic areas using a conventional 10B-carrier (BSH or boronophenylalanine-10B (BPA)). It is especially difficult to deliver 10B from BPA Citation[14]. Consequently, the presence of hypoxic tumour cells is also associated with resistance to BNCT. Thus, a 10B-compound with the characteristics of a hypoxic cytotoxin may be a promising candidate for a 10B-carrier for use in BNCT. In a previous report, the usefulness of TX-2100 compared with BSH as a 10B-carrier in BNCT was demonstrated Citation[7]. However, the use of TX-2100 has not been compared with the simultaneously combined use of its component drugs, TX-402 and BSH. Meanwhile, a previous study concerning the characteristics of intra-tumour Q-cells clarified that the Q-cell population in SCC VII solid tumours has a much larger hypoxic fraction than the total cell population (Q-cells:Total cells = 60.3 (54.8–65.8)%:14.8 (13.4–16.2)%) Citation[15]. Thus, in this study, it was thought that the response of intra-tumour hypoxic cells to irradiation would be represented by the response of intra-tumour Q-cells to some extent.
TX-2100 was easy to solve in a small volume of physiological saline, compared with its component drugs, TX-402 and BSH. Originally, BSH is very easy, but TX-402 is pretty hard to solve there. This was partly because the partition coefficient in octanol/water (p) value for TX-2100 (p = 0.00148) is smaller than that for TX-402 (p = 0.0437) Citation[3]. This point may be one of the advantages in the synthesis of the hybrid compound, TX-2100.
TX-2100 maintained a high concentration of 10B in solid tumours for a sufficiently long time even after the 10B concentration had dropped when both TX-402 and BSH were administered (). Such 10B biodistribution kinetics in tumours is advantageous for BNCT that needs 1–2 h of irradiation. Considering that all these compounds were administered at a dose of 0.75 µmole g−1 body weight of the tumour-bearing mice, the number of 10B atoms administered per kilogram body weight was the same. However, that TX-2100 could maintain a higher 10B concentration in solid tumours after 60 min indicated that the 10B from TX-2100 had greater potential to accumulate in solid tumours than the 10B from its component drugs, TX-402 and BSH.
In addition, in combination with MTH, TX-2100 maintained a higher 10B concentration in solid tumours for a sufficiently longer time probably because of a greater dose distribution through the increase in tumour blood flow induced by the MTH Citation[4],Citation[5]. As far as currently available heating treatments are concerned, MTH is thought to be more practical and easier to use than conventional hyperthermia in which the intra-tumour temperature must be maintained above 42°C Citation[16],Citation[17]. The combination of MTH and the administration of a 10B-carrier is thought to be a useful approach to improving the distribution of 10B in solid tumours.
TX-2100 only or combined with MTH did not cause very high 10B concentrations in the brain, blood, liver, muscle or skin, compared with the administration of both TX-402 and BSH with or without MTH. No 10B concentration at any site examined here was thought to be very toxic Citation[18]. Although the 10B concentrations in the skin were almost the same as those in the tumours, neutron capture reactions between 10B and thermal neutrons are less extensive in skin than in tumours since epithermal neutron beams are often employed in BNCT to obtain thermalized epithermal neutrons at the depth of the tumour and so spare damage to the surface of the skin.
The effects on both the total and Q-cell populations in the use of TX-2100 were significantly greater than those in the simultaneous use of its component drugs, both TX-402 and BSH, even when the 10B concentrations in tumours were kept at similar levels during irradiation (p < 0.05) (). This result also supported the advantage in the design and synthesis of the hybrid compound TX-2100 from the viewpoint of its effectiveness as a 10B-carrier. Further, MTH enhanced the sensitivity of both the total and Q-tumour cell populations significantly to TX-2100 and its component drugs, TX-402 and BSH (p < 0.05) (). It was suggested that the increase in tumour blood flow by MTH Citation[4],Citation[5] induced in the increase in the distribution of each 10B-carrier, as a result, 10B into the solid tumours. Consequently, the sensitivity of the tumour cells was enhanced. Actually, similar mechanisms by MTH were previously demonstrated when combined with anti-cancer agents or hypoxic cytotoxins Citation[19],Citation[20].
Administration of the drug only, i.e. without MTH, enhanced the sensitivity of the total cell populations slightly more markedly than that of Q-cells. In contrast, combined treatment with MTH enhanced the sensitivity of Q-cells more markedly than that of total cells, significantly for TX-402 plus BSH and for TX-2100 (p < 0.05) and slightly for BSH. Also in , the enhancing effect of MTH was greater on Q-cells than on total cells. As a result, the combination with MTH reduced the difference in sensitivity between total and Q-cells for all irradiation conditions (). Meanwhile, the enhancing effect of MTH was slightly greater for TX-2100 than for TX-402 plus BSH in both total and Q-cells (). As shown in , it might be partly because of the difference between TX-2100 and TX-402 plus BSH in pattern of the MTH-induced increase in the 10B concentration in the tumour after the end of MTH and during neutron irradiation. Namely, after MTH for 30 min and during subsequent neutron irradiation for 15–45 min, the increase in the 10B concentration in the tumour by combination with MTH for TX-2100 administration was kept higher than that for TX-402 plus BSH administration (). Tumour hypoxia is a direct consequence of structural abnormalities of the micro-vasculature and functional abnormalities of the micro-circulation in solid tumours and results from either limited oxygen diffusion (chronic hypoxia) or limited perfusion (acute hypoxia, transient hypoxia or ischemic hypoxia) Citation[21]. According to a previous report Citation[22], in the SCC VII tumour, the hypoxic fraction (HF) of total cells includes a large acutely HF and small chronically HF. In contrast, the HF of Q-cells is made up of a large chronically HF and small acutely HF. In the present study, it was thought that MTH increased tumour blood flow resulting in a reduction in the extent of chronic hypoxia in the solid tumours and bringing about the distribution of a higher dose of drug to intermediately hypoxic regions derived mainly from chronic hypoxia-rich Q-cells.
Table IV. Dose ratios* for quiescent tumour cells relative to the total tumour cell population.
Solid tumours, especially human tumours, are thought to contain a high proportion of Q-cells Citation[21],Citation[23]. The presence of these cells is probably due, in part, to hypoxia and the depletion of nutrition in the tumour core and this is another consequence of poor vascular supply Citation[21],Citation[23]. It has been reported that Q-cells have lower radio-sensitivity than P-cells in solid tumours in-vivo Citation[2],Citation[21],Citation[23]. Actually, also in this study, Q-cells showed the significantly lower sensitivity than total cells under thermal neutron only irradiation without any drugs (, ). In addition, since Q-cells have significantly larger size of HF than total cells and less potential to uptake 10B from 10B-carriers, Q-cells still showed the significantly lower sensitivity than total cells under thermal neutron irradiation following the administration of a 10B-carrier (, ). This means that more Q-cells survive anti-cancer BNCT treatment than P-cells. Consequently, the control of Q-cells has a great impact on the outcome of BNCT. TX-2100 not only had significantly greater effects on both total and Q-cells with neutron irradiation than the concurrent administration of its component drugs, TX-402 and BSH, but also in combination with MTH, increased the sensitivity of Q-cells more markedly than that of total cells, resulting in a reduction in the difference in sensitivity between the total and Q-cell populations. In terms of the tumour cell-killing effect as a whole, including intra-tumour Q-cell control, the use of TX-2100 combined with MTH is a promising approach to the administration of a 10B-carrier for BNCT. Further, the sensitizing effect in combination with MTH should be tested when developing new 10B-carriers.
Finally, according to , TX-2100 is significantly more cytotoxic to both total and Q-cells than BSH. Therefore, further study to reduce this toxicity will have to be carried out.
Acknowledgements
This study was supported, in part, by a Grant-in-aid for Scientific Research (C) (16591204) from the Japan Society for the Promotion of Science.
References
- Barth RF, Coderre JA, Vicente MGH, Blue TE. Boron neutron capture therapy of cancer: Current status and future prospects. Clin Cancer Res 2005; 11: 3987–4002
- Masunaga S, Ono K. Significance of the response of quiescent cell populations within solid tumors in cancer therapy. J Radiat Res 2002; 43: 11–25
- Nagasawa H, Yamashita M, Mikamo N, Shimamura M, Oka S, Uto Y, Hori H. Design, synthesis and biological activities of antiangiogenic hypoxic cytotoxin, triazine-N-oxide derivatives. Comp Biochem Physiol A Mol Integr Physiol 2002; 132: 33–40
- Griffin RJ, Okajima K, Ogawa A, Song CW. Radiosensitization of two murine tumours with mild temperature hyperthermia and carbogen breathing. Int J Radiat Biol 1999; 75: 1299–1306
- Masunaga S, Ono K, Hori H, Kinashi Y, Suzuki M, Takagaki M, Kasai S, Nagasawa H, Uto Y. Modification of tirapazamine-induced cytotoxicity in combination with mild Hyperthermia and/or nicotinamide: Reference to effect on quiescent tumour cells. Int J Hyperthermia 1999; 15: 7–16
- Masunaga S, Ono K, Suzuki M, Takagaki M, Sakurai Y, Kobayashi T, Akuta K, Akaboshi M, Kinashi Y, Abe M. Modification of the response of a quiescent cell population with a murine solid tumour to boron neutron capture irradiation: Studies with nicotinamide and hyperthermia. Br J Radiol 1997; 70: 391–398
- Masunaga S, Nagasawa H, Gotoh K, Sakurai Y, Uto Y, Hori H, Nagata K, Suzuki M, Maruhashi A, Kinashi Y, Ono K. Evaluation of hypoxia-specific bioreductive agent-sodium borocaptate-10B conjugates as 10B-carriers in boron neutron capture therapy. Radiat Med 2006; 24: 98–107
- Hori H, Jin C-Z, Kiyono M, Shimamura M, Inayama S. Design, synthesis, and biological activity of anti-angiogenic hypoxic cell radiosensitizer haloacrtylcarbamoyl-2-nitroimidazoles. Bioorg Med Chem 1997; 5: 591–599
- Kobayashi T, Kanda K. Microanalysis system of ppm-order 10B concentrations in tissue for neutron capture therapy by prompt γ-ray spectrometry. Nucl Instrum Meth 1983; 204: 525–531
- Nishimura Y, Ono K, Hiraoka M, Masunaga S, Jo S, Shibamoto Y, Sasai K, Abe M, Iga K, Ogawa Y. Treatment of murine SCC VII tumors with localized hyperthermia and temperature-sensitive liposomes containing cisplatin. Radiat Res 1990; 122: 161–167
- Caswell RS, Coyne JJ, Randolph ML. Kerma factors for neutron energies below 30 MeV. Radiat Res 1980; 83: 217–254
- Sakurai Y, Kobayashi T. Characteristics of the KUR heavy water neutron irradiation facility as a neutron irradiation field with variable energy spectra. Nucl Instr Meth Phys Res A 2000; 453: 569–596
- Brown JM. Exploiting the hypoxic cancer cell: Mechanisms and therapeutic strategies. Mol Med Today 2000; 6: 157–162
- Masunaga S, Ono K, Sakurai Y, Takagaki M, Kobayashi T, Suzuki M, Kinashi Y, Akaboshi M. Response of quiescent and total tumor cells in solid tumors to neutrons with various cadmium ratios. Int J Radiat Oncol Biol Phys 1998; 41: 1163–1170
- Masunaga S, Ono K, Akaboshi M, Nishimura Y, Suzuki M, Kinashi Y, Takagaki M, Hiraoka M, Abe M. Reduction of hypoxic cells in solid tumours induced by mild hyperthermia: Special reference to differences in changes in the hypoxic fraction between total and quiescent cell populations. Br J Cancer 1997; 7: 588–593
- Song CW, Park HJ, Griffin RJ. Improvement of tumor oxygenation by mild hyperthermia. Radiat Res 2001; 155: 515–528
- Song CW, Park HJ, Lee CK, Griffin RJ. Implications of increased tumor blood flow and oxygenation caused by mild temperature hyperthermia in tumor treatment. Int J Hyperthermia 2005; 21: 761–767
- Maruyama K, Ishida O, Kasaoka S, Takizawa T, Utoguchi N, Shinohara A, Chiba M, Kobayashi H, Eriguchi M, Yanagie H. Intracellular targeting of sodium mercaptoundecahydrododecaborate (BSH) to solid tumors by transferrin-PEG liposomes, for boron neutron-capture therapy (BNCT). J Control Rel 2004; 98: 195–207
- Masunaga S, Ono K, Suzuki M, Kinashi Y, Takagaki M, Hori H, Kasai S, Nagasawa H, Uto Y. Usefulness of tirapazamine as a combined agent in chemoradiation and thermo-chemoradiation therapy at mild temperatures—reference to the effect on intratumor quiescent cells. Jpn J Cancer Res 2000; 91: 566–572
- Masunaga S, Nagasawa H, Uto Y, Hori H, Suzuki M, Nagata K, Kinashi Y, Ono K. The usefulness of continuous administration of hypoxic cytotoxin combined with mild temperature hyperthermia, with reference to effects on quiescent tumor cell populations. Int J Hyperthermia 2005; 21: 305–318
- Vaupel P, Kelleher DK, Hoeckel M. Oxygenation status of malignant tumors: Pathogenesis of hypoxia and significance for tumor therapy. Semin Oncol 2001; 28(Suppl 8)29–35
- Masunaga S, Ono K, Abe M. The detection and modification of the hypoxic fraction in quiescent cell populations in murine solid tumors. Br J Radiol 1993; 66: 918–926
- Brown JM, Giaccia AJ. The unique physiology of solid tumors: Opportunities (and problems) for cancer therapy. Cancer Res 1998; 58: 1408–1416