Abstract
Purpose: The purpose of this study was to construct and perform preliminary functionality evaluations of a multilayer conformal applicator with provisions for thermal monitoring, tight conformity and simultaneous microwave heating and brachytherapy treatment of large-area contoured surfaces.
Materials and methods: The multilayer conformal applicator consists of thermal monitoring catheters for fibre-optic monitoring of skin temperatures, a waterbolus, a PCB microwave antenna array, a dielectric spacer for brachytherapy considerations, brachytherapy catheters for delivering HDR radiation and an inflatable air bladder for improving conformity to contoured surfaces. The applicator also includes an elastic attachment structure to hold the applicator securely in place on the patient. The conformity of the applicator to irregular surfaces was evaluated through CT imaging of the applicator fitted onto a life-sized human torso phantom. The fluid flow dynamics of the waterbolus, which impact the effectiveness of temperature control, were evaluated with thermometry during a 19°C step change temperature of the circulating water.
Results: CT imaging showed improved conformity to the torso phantom surface following the application of gentle inward pressure from inflating the outer air bladder. Only a small number of 1–5 mm sized air gaps separated the conformal applicator and tissue surface. Thermometry testing of the bolus fluid flow dynamics demonstrated temperature uniformity within ±0.82°C across a 19 × 34 × 0.6 cm area bolus and ±0.85°C across a large 42 × 32 × 0.6 cm area bolus.
Conclusion: CT scans of the applicator confirmed that the applicator conforms well to complex body contours and should maintain good conformity and positional stability even when worn on a mobile patient. Thermometry testing of two different waterbolus geometries demonstrated that uniform circulation and temperature control can be maintained throughout large, complex bolus shapes.
Introduction
Local hyperthermia used in conjunction with radiation therapy has been proven effective in the treatment of superficial tissue disease Citation[1–5]. Although laboratory data has demonstrated that the synergism of these modalities is maximized when treatments are delivered simultaneously Citation[6], the technology for delivering hyperthermia simultaneously with radiation has not been available in the patient clinic. Universities have only recently begun to develop new equipment capable of simultaneous therapy Citation[7–10] and despite slow progress due to a lack of approved equipment and protocols, initial clinical results have been promising Citation[11]. One application that stands to benefit from this approach is the treatment of recurrent breast carcinoma in the chestwall, which often spreads into diffuse regions across the torso and is resistant to the best available therapies Citation[4], Citation[12]. This site presents a particular challenge, as it becomes necessary to treat large areas of disease spreading into otherwise normal skin that overlies complex body contours. Such treatment is possible, however, using a conformal applicator capable of treating large areas of superficial tissue disease with simultaneous microwave hyperthermia and radiation therapy.
Microwave applicators have been used successfully for years to heat small areas of superficial tissues Citation[13–15], but the challenge has been to extend the heating to larger areas of contoured anatomy Citation[12], Citation[16]. Consequently, a critical component of the new conformal applicator is the waterbolus, which provides a homogenous, high-dielectric-constant interface of controlled temperature between a large microwave array applicator and the skin surface. The temperature-regulated water provides surface cooling to minimize risk of over-heating, which might cause thermal blisters if not maintained below 45°C for the 60-min heat treatment. A number of different coupling boluses have already been designed for use with microwave antennae to exchange heat Citation[17–22]. A waterbolus suitable for the current project, however, has a number of additional requirements: the bolus must include thermal monitoring of the skin surface to provide feedback for control of microwave power to multi-element antenna arrays; a high water flow rate is required to maintain uniform surface temperatures under the multiple, independently powered antennae; and the bolus must conform to convex or concave body contours (e.g. sternum, axilla) and remain securely but comfortably in place on a moving patient for 90 min or longer, considering the time to set up and deliver treatment. These criteria may be fulfilled by forming a conformal applicator from multiple layers: thermal monitoring catheters for fiber-optic monitoring of skin temperatures, a waterbolus for microwave coupling and surface temperature control, a printed circuit board (PCB) microwave array for heating, a dielectric spacer for brachytherapy dose considerations, brachytherapy catheters for delivering high dose rate (HDR) radiation and an outer inflatable air bladder for improving conformity of the applicator to the contoured skin surface.
The present study describes the design and evaluation of this multilayer conformal applicator for treating large-area superficial disease with simultaneous heat and brachytherapy. Quantitative results characterize the applicator's effective conformity to the complex contours of the human torso, as well as the internal water flow dynamics that affect both temperature uniformity across the large surface and response time to a step change in circulating water temperature.
Methods
Conformal applicator construction
The conformal applicator consists of six distinct layers (): thermal monitoring catheters (), a waterbolus (), a PCB microwave array (), a dielectic spacer (), brachytherapy catheters (), and an inflatable air bladder (). The complete applicator also includes an elastic attachment structure for securing the applicator to the patient ().
Figure 1. Diagram of the layers in the multilayer conformal applicator, including thermal monitoring catheters, a waterbolus, a printed circuit board microwave array, a dielectric spacer, brachytherapy catheters, and an inflatable air bladder. The stretch fabric is a separate elastic garment that may be worn over the applicator.
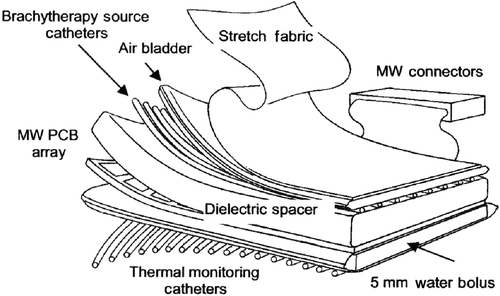
Figure 2. Sequential diagrams of an ‘L-shaped’ multilayer conformal applicator showing the different layers. (a) Rear view of the applicator showing elastic straps and skin-contacting thermometry catheters; (b) front view of the water bolus; (c) PCB array; (d) dielectric spacer; (e) brachytherapy catheters; (f) inflatable air bladder.
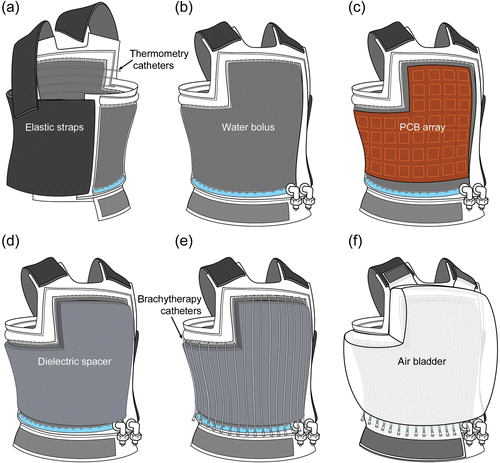
Waterbolus chamber
Several considerations must be taken with the materials used in constructing the waterbolus. To maintain good contact between the coupling bolus and the tissue surface, the bolus material must also be soft and pliable to conform comfortably to the patient's body. The bolus material must be impermeable to water and biological fluids to prevent water loss from the bolus and contamination with body fluids in contact with the bolus. This preserves sanitary conditions such that the bolus may be used on multiple patients following suitable surface cleansing. These requirements are satisfied by using thin, medical-grade, blood-barrier-rated polyvinyl chloride (PVC) of 0.3 mm (12 mil) thickness that is impenetrable to air, water, and body fluids. Layers of PVC material are heat sealed around the perimeter to fabricate a closed bag around a core of porous open cell foam added to help maintain uniform thickness of ∼5 mm across the entire bolus. A nearly uniform thickness waterbolus is important for optimal heating of superficial tissue as flexible PCB microwave antenna arrays have been designed for most uniform heating when coupled through a thin layer of water <9 mm thick Citation[23], Citation[24]. Although minor variations in bolus thickness can be accommodated by varying the microwave power Citation[24] and radiation dwell time characteristics Citation[25], maintaining a stable bolus thickness profile from initial set-up until the end of treatment delivery is critical to delivering the pre-planned doses.
In order to cool the skin effectively, the bolus must circulate temperature-controlled water uniformly and rapidly across the entire surface of the treatment area. Earlier boluses designed specifically for the conformal microwave applicator channelled water into and out of the bolus using flexible Tygon tubes with numerous holes bored into the sidewall of the tubing. The feed tube running along the top of the bolus provided even distribution of water across the bolus surface using a peristaltic pump to circulate water into and out of the bolus at equal rates. There were a number of drawbacks to using Tygon tubing for the inlet channel, however, particularly in bolus shapes other than simple rectangular structures. Connecting semi-rigid tubing so that the supply line could follow the perimeter of complex curves, such as in a bolus vest, was a complicated process that restricted bolus geometry. This was a particular concern when designing a bolus for treating large-area superficial disease, as simple rectangular boluses cannot simultaneously treat many areas of the human torso that are typically affected by superficial disease (e.g. under the arm and over the shoulder) because of poor fit to the human body. In addition, the feed tubing was prone to shifting position within the bag (e.g. cutting across corners and falling away from the outer perimeter), potentially affecting water flow distribution within the bolus layer.
To avoid such difficulties, the current effort redesigned the bolus to integrate a heat-sealed open water channel into the outer edge of the bolus for the water inlet in rectangular (), ‘L’ (), and vest-shaped boluses Citation[20]. Regularly spaced 3 mm wide gaps along the inside of the water channel allow uniform water dispersion into the bolus interior. This method of distributing water into the bolus interior from all around the bolus perimeter insures that the water flow will remain constant even during patient movement as the peripheral water channel does not shift relative to the bolus compartment. Furthermore, heat-sealing gives greater flexibility in bolus design, both in terms of designing a water channel that will deliver uniform water flow across the bolus and designing the shape of the bolus to better fit the human torso.
Figure 3. Design diagrams for waterboluses showing water ports, channels, and numbered thermocouple locations used in thermometry evaluation of fluid flow dynamics. (a) Rectangular bolus with a 19 × 34 cm treatment area; (b) ‘L’ shaped bolus with a 32 × 42 cm treatment area.
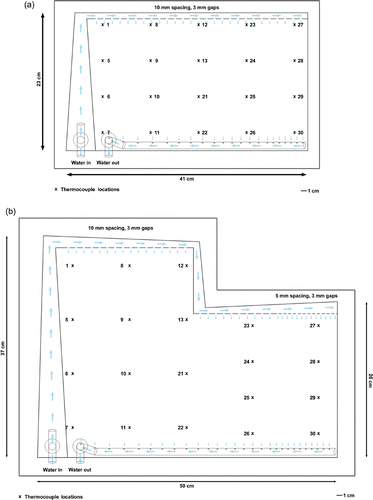
The return flow side of the waterbolus chamber is constructed from perforated, semi-rigid PVC tubing. The tube is glued along the straight bottom edge of the bolus interior to maintain the position of the tube within the bolus and the direction of the holes in the tubing into the open cell foam for unimpeded removal of water from the bolus. This tube is designed to draw water from the bolus under vacuum from a vacuum chamber that has been incorporated into the external water conditioning system.
PCB microwave array
Microwave power is delivered from a 0.2–0.3 mm thick, flexible PCB micro-strip antenna array that is sandwiched between the waterbolus and the dielectric spacer (). The multilayer PCB microwave array consists of individually powered dual concentric conductor (DCC) 3–4 cm square slot antennae etched from the front surface ground plane and driven incoherently at 915 MHz or 433 MHz Citation[26]. Up to 32 apertures may be driven simultaneously with the current conformal microwave array (CMA) system, though the largest ‘L’ and vest shaped arrays may have more than 32 apertures to allow custom selection of appropriate apertures that best correspond to the location of the target disease. Lightweight 3 mm diameter coaxial cables are connected to miniature RF connectors on one edge of the PCB array and power transmitted to the individual DCC apertures by the micro-strip feedline network.
The heating performance of DCC aperture antennae has been evaluated extensively with electromagnetic analysis programs and verified with experimental measurements in both laboratory and clinical environments. The specific absorption rate (SAR) patterns of 2–6 cm square DCC apertures have been characterized with a custom Finite Difference Time Domain (FDTD) program for numerous practical tissue conditions including homogenous muscle Citation[27–31], layered fat and muscle tissue Citation[32], muscle or layered fat and muscle tissue with underlying bone (i.e. ribs), air bubble perturbers between the coupling bolus and tissue surface, and variable thickness waterbolus Citation[24]. More recently, design optimization of the DCC antennae has continued using commercial electromagnetic simulation software to further improve impedance match and radiation efficiency of the DCC apertures Citation[33], Citation[34]. The complexity of CMA applicators has continued to increase, first by combining the heating capabilities of DCC slot apertures with non-invasive radiometric monitoring of tissue temperature Citation[30], Citation[35–38] and subsequently by adding the capability of simultaneous brachytherapy with heat treatments Citation[10], Citation[20], Citation[39].
Thermal monitoring catheters
The bolus includes provision for monitoring temperatures of the skin surface by scanning fiber-optic temperature sensors inside catheter tubing that is attached to the patient side of the waterbolus membrane (). Temperature monitoring catheters are glued to the bolus surface at regular intervals across the applicator face to facilitate temperature readings under and between each DCC antenna of the array.
Dielectric spacer
A layer of 8–10 mm thick thermally and electrically insulating foam serves as a dielectric spacer between the PCB microwave array and the brachytherapy catheters attached to the patient side of the outer inflatable air bladder (). The dielectric spacer is used to increase the brachytherapy source to skin distance in order to homogenize the radiation dose delivered to the target as described in Taschereau et al. Citation[25]. The additional separation also minimizes interaction between the brachytherapy sources and fiber-optic thermometry sensors, as recommended in previous investigations Citation[40].
Brachytherapy catheters
Brachytherapy catheters for scanning the HDR radiation source are attached to the inner surface of the air bladder () facing the PCB microwave array such that when the air chamber is secured to the waterbolus and inflated, the brachytherapy catheters are pressed snugly into the back surface of the dielectric spacer and conformal PCB array. The brachytherapy catheters are attached parallel to each other with 1 cm spacing to facilitate uniform irradiation of the superficial tissue target as reported previously Citation[25].
Inflatable air bladder
Contact between the bolus and contoured tissue surface may be improved by the incorporation of an inflatable air bladder over the PCB microwave array on the exterior of the waterbolus (). This compartment is formed from two additional layers of PVC material heat sealed around the outer perimeter and is fitted with a single quick connect fill port. For enhanced applicator versatility, the air bladder may be constructed as a separable layer and attached to the bolus with Velcro® strips along all four edges. The Velcro® allows the air bladder to be lifted away from the bolus for convenient alignment of the PCB microwave array and then secured to the waterbolus for treatment. When inflated with air, the bladder applies gentle inward pressure on all other layers of the applicator to improve conformation to concave body contours as well as the convex torso surface. This additional inward pressure helps eliminate any remaining air space between the waterbolus and skin surfaces.
Attachment structure
The coupling bolus is secured snugly but comfortably to the patient with adjustable elastic straps that are attached to the outer edge of the bolus with Velcro® fasteners (). After the wide elastic bands are stretched around the torso, the Velcro® closures secure the bolus in place over contoured anatomy in both reclining and upright positions. The snug fit of bolus to skin surface minimizes the chance of trapping air pockets between the bolus and patient, which is necessary to prevent distortion of the microwave power deposition pattern. The wide range of adjustability provided by the elastic straps and Velcro® closures helps accomplish constant tension across the bolus surface for uniform inward pressure against the torso. With appropriate strap location and tension, the weight of the bolus assembly is entirely supported and the patient is free to move during the course of treatment.
Performance evaluation
Conformity
The ability of the coupling bolus to conform to the complex surface of a human torso was tested by securing an ‘L’ shaped applicator to a human torso CT phantom (RANDO®—The Phantom Laboratory, Salem, NY) with an intact breast prosthesis constructed from a shaped polyurethane bag filled with water and attached on one side of the chest. The CT phantom surface was lubricated with a thin layer of ultrasound gel. Using the same skin preparation for clinical treatments, which facilitates positioning the bolus over the target disease and provides good thermal conduction from bolus to skin. The elastic straps were adjusted as necessary and secured with Velcro® fasteners (). Air was then pumped slowly into the air bladder until uniform inward pressure was obtained across the bolus surface. Because an additional elastic garment is often fitted over the top of the applicator to further improve conformance of the bolus and microwave antenna array to particularly complex body contours for clinical treatments, an elastic garment was also fitted over the applicator to simulate clinical conditions for this study (). The phantom and applicator were scanned with a CT scanner to assess conformity both before and after inflation of the air bladder to determine the effectiveness of the air bladder in improving conformity. After the initial scans, the phantom was moved (set upright, jostled, and laid back down) and then scanned again to test that the bolus could maintain good conformity to the surface on a mobile patient.
Flow dynamics
Flow dynamics were evaluated in two different bolus geometries: a simple 19 × 34 cm rectangular shape bolus (), and a more complex 32 × 42 cm ‘L’ shaped bolus () intended to cover the upper chest and around under the arm—areas often affected by diffuse chestwall recurrence. The rectangular bolus features a simple open water channel with uniform width and spacing of 3 mm gaps that distribute water to the bolus interior. The larger ‘L’ shaped bolus includes a more complex water channel design with increases in channel width to compensate for the pressure drop along the channel. It also has reduced spacing between gaps toward the end of the long supply channel (5 mm spacing vs 10 mm spacing near the beginning of the channel) to maintain uniform water dispersion into the bolus interior with increasing distance from the water input port. Both boluses used a perforated PVC vacuum tube glued into the bottom of the water chamber for removal of water from the bolus interior.
The dynamics of fluid flow through these two boluses was tested using a thermocouple thermometry system. Twenty thermocouples were attached to the bolus surface at regular spacing (). Room temperature (∼24°C) degassed water was pumped into the water inlet port to fill the bolus to ∼6 mm thickness. The thin bolus was secured to the surface of an upright human torso model fitted with a single intact breast prosthesis to simulate the chest of a post-mastectomy patient. The water outlet port was connected to an inline vacuum chamber and water conditioning system that continuously degassed the water to prevent microscopic air bubbles from disassociating from the warm water and accumulating on the inner surface of the bolus, which would interfere with microwave coupling. Uniform bolus thickness was maintained using a microprocessor in the water conditioning system, which controlled the peristaltic pump speed to match the inflow rate to the outflow produced by the vacuum as determined by a water height sensor in the vacuum chamber. The water conditioning system also included an inline heat exchanger to control the temperature of water being pumped into the bolus.
Following a sudden change of tubing connections, pre-heated water at 43°C was pumped through the bolus at a flow rate of 1.5 L min−1 for the rectangular bolus and 2.4 L min−1 for the larger ‘L’ shaped bolus. The rate of temperature change over time following connection to the warm water supply was measured at all 20 regularly spaced thermocouple locations across the bolus surface, as shown in .
Results
Conformity
The initial CT scan of the bolus ( and 6(a–c)) on the torso phantom showed good conformity to the contoured surface, starting along the breast prosthesis and then across the sternum and around the side of the chest under the arm. Sharp irregularities in the layered phantom surface caused by circumferential gaps between each 2 cm thick torso section contributed to the formation of wrinkles in the bolus material that resulted in air gaps that ranged from 1–7 mm in size between the waterbolus and the torso surface. The subsequent CT scan following inflation of the air bladder ( and 6(d–f)) showed an improvement in conformity, particularly at the junction of the intact breast and sternum (), and a reduction of the air gap at each discontinuity in the sectioned torso surface to ∼1–5 mm (). After the phantom underwent a series of movements to simulate a mobile patient, another CT scan was taken (), which showed no significant change in bolus conformity or applicator position over the simulated treatment area.
Figure 5. Axial CT cross-sections from the upper torso of a CT phantom fitted with the multilayer conformal applicator. (a) Axial cross-section of the upper torso prior to air bladder inflation; (b) close-up of the bolus over the breast and sternum prior to air bladder inflation; (c) axial cross section of the upper torso after air bladder inflation; (d) close-up of the bolus over the breast and sternum after air bladder inflation.
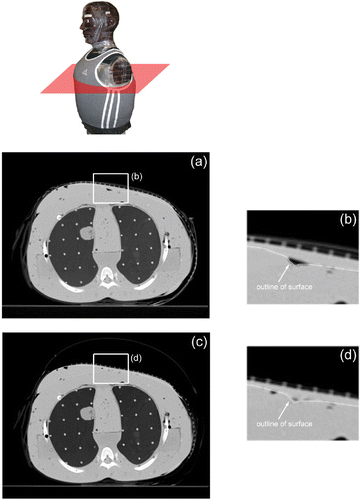
Figure 6. Axial CT cross-sections from the lower torso of a CT phantom fitted with the multilayer conformal applicator. (a) Axial cross-section of lower torso prior to air bladder inflation; (b) close-up of bolus over breast and sternum prior to air bladder inflation; (c) close-up of small air pockets under the arm prior to air bladder inflation; (d) axial cross-section of lower torso after air bladder inflation; (e) close-up of bolus over breast and sternum after air bladder inflation; (f) close-up of reduced air pockets under the arm after air bladder inflation; (g) axial cross-section of lower torso after simulated patient movement.
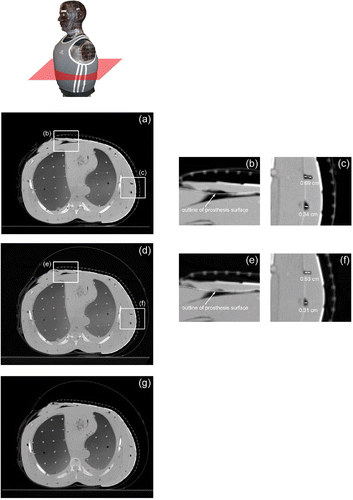
Flow dynamics
The results of the bolus thermometry tests can be seen in . The rates of temperature change measured in 20 locations on the rectangular bolus following the 19°C step change in supply water temperature varied between a maximum of 1.4°C s−1 to a minimum of 0.3°C s−1, with an average rate of 0.9°C s−1. With the exception of three outliers (thermocouple locations 7, 11 and 22 near the exit port), a spread of 1.6°C was observed at steady state out of the overall 19°C rise above baseline (). The rates of temperature change for the ‘L’ shaped bolus varied between a maximum of 1.0°C s−1 to a minimum of 0.4°C s−1, with an average rate of 0.7°C s−1. All locations on the bolus were within a spread of 1.7°C at steady state out of the overall 19°C rise above baseline ().
Discussion
A conformal waterbolus for coupling heat and radiation into tissue is a vital element of a contact applicator for treating large-area superficial disease and is requisite for effective coupling of microwave antenna arrays intended either for heating tissue Citation[12–14], Citation[31], Citation[39], Citation[41], Citation[42] or for non-invasive radiometric monitoring of tissue temperature Citation[36–38], Citation[43], Citation[44]. In addition to providing more control of skin surface temperature, a coupling bolus of correct thickness smoothes microwave antenna SAR peaks for increased heating uniformity Citation[23], Citation[31]. The multilayer conformal applicator described in this effort not only addresses the requirements of a waterbolus, but also provides additional bolus functionality through the addition of brachytherapy catheters for simultaneous radiation therapy, skin-contacting thermometry catheters, and an inflatable air bladder and elastic attachment structure that improves conformity and holds the applicator securely in place on the patient even during patient movement.
Good conformity and securing of the coupling bolus to the patient's contoured anatomy is necessary to prevent distortion of the power deposition pattern from the microwave applicator. To address this, new design considerations of an inflatable air bladder and elastic attachment structure were integrated into the much simpler waterbolus compartments previously used with external microwave hyperthermia applicators. The air bladder applies a gentle inward pressure on both the PCB antenna array and the waterbolus layer, which improves conformity to the contoured torso surface. The improvement is particularly noticeable in concave areas where traditional waterbolus compartments may separate completely from the skin surface. The elastic straps provide a snug and secure fit to the patient, both stretching the bolus to eliminate air pockets between the bolus and the tissue surface and holding the applicator securely in place throughout the course of treatment.
Conformity of the waterbolus to the contoured human torso model was determined through CT analysis. Axial CT cross-sections shown in and verified that the applicator conforms well to complex body contours over a large area, extending across an intact breast, the sternum and the chest to around the side and back of the torso in this test. This is a treatment configuration that occurs frequently in patients with chestwall recurrence of breast carcinoma Citation[12]. The CT scans (Figures 5(c, d) and 6(d–f)) also confirmed that while the flexible bolus and elastic straps provide good conformity to contoured surfaces, the outer air bladder further improves conformity to complex body contours, reducing in size or eliminating air pockets between the bolus and tissue surfaces. Additionally, a comparison between the cross-sections in show that conformity and alignment were maintained following movement and intentional jostling of the phantom and applicator set-up, indicating that the applicator should retain good conformity and stable position over the treatment site on a mobile patient.
A small number of 1–5 mm deep air gaps between the bolus and the phantom surface can be seen in and as the result of unavoidable wrinkling of the bolus material around sharp contour changes. Although trapped air may be expected to cause noticeable perturbations of the microwave field, including a concentration of SAR locally along the lateral edges of the larger pockets Citation[24], the effects of this perturbation are minimized by reducing the amount of trapped air. Furthermore, because the locations of air pockets differ between individual treatments, the minor variations in SAR are not expected to have a significant effect on the cumulative thermal dose distribution from multiple treatments.
In order to maintain the desired temperature range of 41–45°C in both skin and deeper-lying tissues during local hyperthermia with microwave applicators, it is necessary to control the tissue surface temperature to be in balance with the applied microwave power. For increasingly larger treatment areas, it becomes critical to maintain rapid and uniform fluid flow throughout the entire coupling bolus to maintain appropriate surface temperature regardless of tissue properties and required microwave power levels. Uniform fluid flow would show similar rates of temperature rise for all thermocouples regardless of position on the bolus and a relatively small spread of temperature readings at steady state. High rates of temperature change, which indicate ability to effectively add or remove heat from the skin surface, are necessary for the bolus to maintain temperature at the interface between the patient and the applicator for a range of microwave power levels in the multiple antenna array.
The thermometry results from the rectangular bolus in showed that with the exception of a small area near the exit port (thermocouple locations 7, 11 and 22 in ), the bolus demonstrates both rapid circulation and acceptably uniform water distribution, maintaining temperature throughout large treatment areas within a narrow range of ±0.82°C for this 19°C step change. This variation across the bolus should translate to ± 0.35°C for a typical 8°C step change (37–45°C) that would be seen in a clinical situation. The circulation and flow rates in the vicinity of thermocouple locations 7, 11 and 22 can be improved in future designs by simply increasing the frequency of holes in the vacuum tubing in those areas. Thermometry results for the larger ‘L’ shaped bolus in also demonstrated the bolus displays the characteristics of uniform water distribution and circulation throughout the entire bolus, with a temperature variation throughout the treatment area of ±0.85°C for the 19°C step change or ±0.36°C for an 8°C step change. The absence of outliers in the ‘L’ shaped bolus was the result of improvements over an earlier bolus design for treating large-area chestwall recurrence of breast carcinoma Citation[20]. The uniform flow and circulation in this bolus can also be seen in , a series of color wash images that represent the temperature distributions across the bolus from 10–50 s and at steady state after heated water was pumped through the bolus. offers a graphic visualization of both the high flow rate, indicated by the high rate of temperature change, and the uniformity of flow and circulation, as illustrated by the uniform front of warmer temperatures descending the bolus during the step change.
Conclusion
A multilayer conformal applicator has been developed for microwave heating and brachytherapy treatment of superficial tissue disease. Through use of multiple layers, this applicator integrates a microwave coupling bolus with capabilities for thermal monitoring and control of skin temperatures, brachytherapy delivery and a comfortable and secure fit over contoured anatomy. CT scans of the applicator secured on an anatomically accurate human torso phantom confirmed that the applicator conforms well to complex body contours and should maintain good conformity even when worn on a mobile patient. Thermometry testing of two different waterbolus geometries demonstrated that acceptably uniform circulation and temperature control can be maintained throughout large, complex bolus shapes up to 32 × 42 cm. This multilayer conformal applicator should have a positive impact on current treatments for superficial tissue disease, both through improved temperature uniformity and the ability to apply heat and brachytherapy simultaneously to large areas of contoured anatomy.
Acknowledgements
This work was supported by NIH grants RO1-CA70761, R43-CA104061, and R44-RR14940. The authors would also like to acknowledge Chris Diederich and Joe Hsu for contributions to the clinical relevance of this design, Chris Malfatti for the CT scans, and Richard Taschereau and Jean Pouliot for brachytherapy planning considerations.
References
- Sneed PK, Stauffer PR, McDermott MW, Diederich CJ, Lamborn KR, Prados MD, Chang S, Weaver KA, Spry L, Malec MK, Lamb SA, Voss B, Davis RL, Wara WM, Larson DA, Phllips TL, Gutin PH. Survival benefit of hyperthermia in a prospective randomized trial of brachytherapy boost ± hyperthermia for glioblastoma multiforme. Int J Radiat Oncol Biol Phys 1998; 40: 287–295
- Vernon CC. Superficial hyperthermia. Radiation research 1895–1995, U Hagen, H Jung, C Streffer. 10th ICRR Society, Würzburg 1995; 51, vol. 1
- Jones E, Oleson J, Prosnitz L, Samulski T, Vujaskovic Z, Yu D, Sanders L, Dewhirst MW. A randomized trial of hyperthermia and radiation for superficial tumors. JCO. 2005; 23(13)3079–3085
- Kapp DS. Efficacy of adjuvant hyperthermia in the treatment of superficial recurrent breast cancer: Confirmation and future directions. Int J Radiat Oncol Biol Phys 1996; 35: 1117–1121
- Dewey WC. Arrhenius relationships from the molecule and cell to the clinic. Int J Hyperthermia 1994; 10: 457–483
- Overgaard J. The current and potential role of hyperthermia in radiotherapy. Int J Radiat Oncol Biol Phys 1989; 16: 535–549
- Straube WL, Klein EE, Moros EG, Low DA, Myerson RJ. Dosimetry and techniques for simultaneous hyperthermia and external beam radiation therapy. Int J Hyperthermia 2001; 17: 48–62
- Novak P, Moros EG, Straube WL, Myerson RJ. SURLAS: A new clinical grade ultrasound system for sequential or concomitant thermoradiotherapy of superficial tumors: Applicator description. Med Phys 2005; 32: 230–240
- Moros EG, Straube WL, Klein EE, Maurath J, Myerson RJ. Clinical system for simultaneous external superficial microwave hyperthermia and cobalt-60 radiation. Int J Hyperthermia 1995; 11: 11–26
- Stauffer P, Schlorff J, Taschereau R, Juang T, Neuman D, Maccarini P, Pouliot J, Hsu J. Combination applicator for simultaneous heat and radiation. International Conference of the IEEE Engineering in Medicine and Biology Society. IEEE Press, San Francisco 2004
- Myerson RJ, Straube WL, Moros EG, Emami BN, Lee HK, Perez CA, Taylor ME. Simultaneous superficial hyperthermia and external radiotherapy: Report of thermal dosimetry and tolerance to treatment. Int J Hyperthermia 1999; 15: 251–266
- Stauffer PR. Thermal therapy techniques for skin and superficial tissue disease. A critical review, matching the energy source to the clinical need, TP Ryan. SPIE Optical Engineering Press, Bellingham, WA 2000; 327–367
- Stauffer PR. Evolving technology for thermal therapy of cancer. Int J Hyperthermia 2005; 21: 731–744
- Lee ER. Electromagnetic superficial heating technology. Thermoradiotherapy and thermochemotherapy, MH Seegenschmiedt, P Fessenden, CC Vernon. Springer-Verlag, BerlinHeidelberg 1995; 193–217
- Sneed PK, Stauffer PR, Li GC, Stege GJJ. Hyperthermia. Textbook of radiation oncology, SA Leibel, TL Phillips. W B Saunders Co, Philadelphia 1998; 1241–1262
- Perez CA, Gillespie B, Pajak T, Hornback NB, Emami B, Rubin P. Quality assurance problems in clinical hyperthermia and their impact on therapeutic outcome: A report by the Radiation Therapy Oncology Group. Int J Radiat Oncol Biol Phys 1989; 16: 551–558
- Sherar MD, Liu FF, Newcombe DJ, Cooper B, Levin W, Taylor WB, Hunt JW. Beam shaping for microwave waveguide hyperthermia applicators. Int J Radiat Oncol Biol Phys 1993; 25: 849–857
- de Bruijne M, Samaras T, Bakker JF, van Rhoon GC. Effects of waterbolus size, shape and configuration on the SAR distribution pattern of the Lucite cone applicator. Int J Hyperthermia 2006; 22: 15–28
- Sherar MD, Clark H, Cooper B, Kumaradas J, Liu FF. A variable microwave array attenuator for use with single-element waveguide applicators. Int J Hyperthermia 1994; 10: 723–731
- Juang T, Neuman D, Schlorff J, Stauffer PR. Construction of a conformal water bolus vest applicator for hyperthermia treatment of superficial skin cancer. International Conference of the IEEE Engineering in Medicine and Biology Society. IEEE Press, San Francisco 2004
- Chou CK, McDougall JA, Chan KW, Luk KH. Evaluation of captive bolus applicators. Med Phys 1990; 17: 705–709
- Chan KW, McDougall JA, Chou CK. FDTD simulations of Clini-Therm applicators on inhomogeneous planar tissue models. Int J Hyperthermia 1995; 11: 809–820
- Neuman DG, Stauffer PR, Jacobsen S, Rossetto F. SAR pattern perturbations from resonance effects in water bolus layers used with superficial microwave hyperthermia applicators. Int J Hyperthermia 2002; 18: 180–193
- Rossetto F, Stauffer PR. Effect of complex bolus-tissue load configurations on SAR distributions from dual concentric conductor applicators. IEEE Trans Biomed Eng 1999; 46: 1310–1319
- Taschereau R, Stauffer PR, Hsu IC, Schlorff JL, Milligan AJ, Pouliot J. Radiation dosimetry of a conformal heat-brachytherapy applicator. Technol Cancer Res Treat 2004; 3: 347–358
- Rossetto F, Stauffer PR. Theoretical characterization of dual concentric conductor microwave array applicators for hyperthermia at 433 MHz. Int J Hyperthermia 2001; 17: 258–270
- Stauffer PR, Leoncini M, Manfrini V, Gentili GB, Diederich CJ, Bozzo D. Dual concentric conductor radiator for microwave hyperthermia with improved field uniformity to periphery of aperture. IEICE Trans Comm 1995; E78-B: 826–835
- Stauffer PR, Rossetto F, Leoncini M, Gentilli GB. Radiation patterns of dual concentric conductor microstrip antennas for superficial hyperthermia. IEEE Trans Biomed Eng 1998; 45: 605–613
- Rossetto F, Stauffer PR, Manfrini V, Diederich CJ, Biffi Gentili G. Effect of practical layered dielectric loads on SAR patterns from dual concentric conductor microstrip antennas. Int J Hyperthermia 1998; 14: 513–534
- Stauffer P, Jacobsen S, Rossetto F, Diederich C, Neuman D. Dual mode antenna array for microwave heating and non-invasive thermometry of superficial tissue disease. Thermal treatment of tissue with image guidance, TP Ryan, TZ Wong. SPIE Optical Engineering Press, Bellingham, WA 1999; 139–147
- Rossetto F, Diederich CJ, Stauffer PR. Thermal and SAR characterization of multielement dual concentric conductor microwave applicators for hyperthermia, a theoretical investigation. Medical Physics 2000; 27: 745–753
- Manfrini V, Gentilli GB, Leoncini M, Helifa A, Rossetto F, Stauffer P. EM analysis of dual concentric conductor planar applicators for superficial microwave hyperthermia. International Congress of Hyperthermic Oncology, C Franconi, G Arcangeli, R Cavaliere, RomaItaly 1996; 506–508
- Maccarini PF, Rolfsnes HO, Neuman DG, Stauffer PR. Optimization of a dual concentric conductor antenna for superficial hyperthermia applications. International Conference of the IEEE Engineering in Medicine and Biology Society, ZP Liang. IEEE Press, San Francisco 2004
- Maccarini PF, Rolfsnes HO, Neuman D, Johnson JE, Juang T, Stauffer PR. Electromagnetic optimization of dual concentric conductor radiator used with a radiometric antenna for temperature controlled superficial heating. Society for Thermal Medicine, Washington, DC 2005
- Stauffer PR, Neuman D, Hwang C, Rossetto F, Diederich CJ. Proceedings of the First Joint BMES/EMBS Conference. Microwave vest for hyperthermia treatment of large area superficial disease. 1999, p 1275, vol. 2
- Jacobsen S, Stauffer PR, Neuman DG. Dual-mode antenna design for microwave heating and noninvasive thermometry of superficial tissue disease. IEEE Trans Biomed Eng 2000; 47: 1500–1509
- Stauffer PR, Jacobsen S, Neuman D, Rossetto F. Progress toward radiometry controlled conformal microwave array hyperthermia applicator. IEEE Engineering in Medicine and Biology Society, Chicago 2000; 4
- Stauffer PR, Jacobsen S, Neuman D. Microwave array applicator for radiometry controlled superficial hyperthermia. Thermal treatment of tissue: Energy delivery and assessment. San Jose: Proceedings of SPIE, TP Ryan, 2001; 19–29
- Stauffer PR, Schlorff JL, Juang T, Neuman DG, Johnson JE, Maccarini PF, Pouliot J. Progress on system for applying simultaneous heat and brachytherapy to large-area surface disease. SPIE BIOS 2005, TP Ryan. SPIE Press, San Jose 2005; 15
- Straube WL, Meigooni AS, Moros EG, Williamson JF, Myerson RJ. High dose-rate induced temperature artifacts: thermometry considerations for simultaneous interstitial thermoradiotherapy. Int J Radiat Oncol Biol Phys 1994; 30: 399–403
- Lee ER, Wilsey TR, Tarczy-Hornoch P, Kapp DS, Fessenden P, Lohrbach AW, Prionas SD. Body conformable 915 MHz microstrip array applicators for large surface area hyperthermia. IEEE Trans Biomed Eng 1992; 39: 470–483
- Gelvich EA, Mazokhin VN. Contact flexible microstrip applicators (CFMA) in a range from microwaves up to short waves. IEEE Trans Biomed Eng 2002; 49: 1015–1023
- Jacobsen S, Murberg A, Stauffer PR. Characterization of a transceiving antenna concept for microwave heating and thermometry of superficial tumors. J Electromag Waves Applic 1998; 12: 351–352
- Jacobsen S, Stauffer PR. Improved spatial resolution in thermography obtained by a two-layered structure of microstrip spirals. J Electromag Waves Applic 1999; 13: 307–323