Abstract
Purpose: The effect of whole body hyperthermia (WBH) at 41.8–42°C on the cellular immune system is still poorly investigated. The aim of this study was to identify genes that become upregulated in peripheral blood lymphocytes (PBLs) of cancer patients during a combined treatment with WBH and chemotherapy by generating complex arrays of cDNA.
Methods: PBLs were obtained from four patients with different malignancies treated with WBH and varying cytostatic schedules before treatment and immediately thereafter. After constructing subtracted cDNA libraries, clones were screened for cDNA induction by dot-blot and semi-quantitative RT-PCR (sq-RT-PCR).
Results: Among 192 clones, 39 cDNAs were significantly upregulated. Sequencing revealed three groups of genes for which upregulation of mRNA was confirmed by sq-RT-PCR. The first group consisted of genes encoding for various heat shock proteins (HSP 60, 90a, 90b, 105). Further sq-RT-PCR demonstrated differential expression of HSP27 and HSP70 as well. The second group (calcyclin-binding-protein, haemoglobin-beta-chain) comprised genes without pre-specified association to hyperthermia. The cDNA encoding macrophage-inflammatory-protein-1-beta was also observed and may be associated with the pre-described activation of lymphocyte sub-populations during WBH.
Conclusion: Treatment with WBH and chemotherapy elicits significant short-term effects on the expression of a variety of genes responsible for cellular integrity, stimulation and migration of immune effector cells. Further investigation is warranted to more clearly define the role of those genes for the clinical effect of WBH.
Introduction
Various reports on the cellular, molecular and immunologic effect of hyperthermia are available which have mostly been derived from in vitro and animal models. One of the hallmarks of hyperthermia research is that external heat induction has a self-contained cytotoxic effect at temperatures >43°C which is clearly enhanced with the synchronous application of radiation and certain cytotoxic drugs already at lower temperatures. More recently, it has been demonstrated that hyperthermia exhibits its cell-killing effect by induction of necrosis, apoptosis or cell cycle-arrest. In addition, various humoral (tumour blood, nutrient and oxygen supply; hormonal and metabolic changes) and cellular (alterations of structures associated to the cell membrane and cytoskeleton, macromolecule synthesis, DNA-repair, intra-cellular signalling pathways; induction of heat shock protein synthesis) effectors of hyperthermia have been identified and characterized Citation[1–6].
Recent results have also highlighted a number of mechanisms by which hyperthermia may interact with the immune system, such as changes in cytokine patterns and alterations of lymphocyte sub-populations Citation[2], Citation[7]. In this context, the fact that hyperthermia is capable to induce the synthesis of various heat shock proteins (HSPs) has gained particular interest. Indeed, HSPs are believed to procure more or less specific immunogenic effects and thus have attracted interest for tumour vaccination and gene therapeutic strategies Citation[8], Citation[9]. However, even if the idea that hyperthermia could be implemented into modern immunotherapeutic approaches is striking, the effect of systemic heat application on the human immune system is still poorly understood. Indeed, only a few clinical studies have addressed this topic, referring to investigations of cytokine and lymphocyte alterations in patients suffering from heat stroke Citation[10], in volunteers treated within a hot water bath Citation[11–13] or in cancer patients treated with either moderate (‘fever-range’) Citation[14] or extreme (41.5–42°C) Citation[15–18] whole-body hyperthermia (WBH). However, no clinical data on the molecular effects of WBH on immune effector cells are yet available which may help to provide insight into whether this treatment modality may exert its clinical anti-tumour effects.
This study presents the results of a pilot study in which suppression subtractive hybridization (SSH) was used to define the cDNA profile that is induced in peripheral blood lymphocytes of cancer patients immediately after a combined treatment with WBH and chemotherapy.
Materials and methods
Patients and blood samples
Four consecutive patients who were treated with a combination of WBH and chemotherapy at the institution were enrolled. All patients suffered from different metastatic tumours (colorectal cancer: n = 2; ovarian cancer: n = 1; cystadenocarcinoma of the parotis gland: n = 1) and had progressed after treatment with conventional chemotherapy. The two patients with colorectal cancer were treated with folinic acid and 5-flourouracil, combined with mitomycin Citation[19] or oxaliplatin Citation[20], respectively, the patient with metastatic cystadenocarcinoma of the parotis gland received carboplatin (CBCDA) and the patient with ovarian cancer was treated with CBCDA and ifosfamide Citation[21]. Details on the treatment procedure are given in Hildenbrandt et al. Citation[19], Citation[22]. The first set of peripheral blood samples was obtained immediately after intubation and induction of total intravenous anaesthesia at a body-core temperature of 37.0°C. Citation[23], Citation[24]. The second set of blood samples was drawn after a body-core temperature of 42°C has been maintained for 60 min and chemotherapy was completed.
The study protocol was approved by the local ethics committee and detailed written informed consent was obtained from every patient prior to treatment.
Isolation of peripheral blood lymphocytes and flow cytometry
Isolation of peripheral blood lymphocytes (PBL) was performed by density gradient centrifugation on Ficoll-Hypaque (Biochrom, Berlin, Germany). Using all cells from the PBL inter-phase ring, this standard procedure generally results in a purity of 90–95% of lymphocytes. Those were further analysed with regard to their sub-populations by flow cytometric analyses using mAbs from Coulter Immunotech (Marseilles, France) against CD3 (clone UCHT1), CD4 (13B8.2), CD8 (B9.11), CD14 (RM052), CD16 (3G8), CD19 (J4.119), CD38 (T16), HLA-DR (B8.12.2) and CD56 (N901).
RNA isolation and reverse transcription
Total cellular RNA was harvested from 1 × 106 PBLs using RNeasy-Kit (Qiagen, Hilden, Germany) followed by DNase I treatment (Promega, Mannheim, Germany). In order to increase the likelihood of isolating genes that were representative for all patients investigated, RNAs of all patients were combined equally. To obtain a sufficient amount of double-stranded cDNA (ds-cDNA) for subtraction experiments, cDNAs were pre-amplified with a SMART PCR cDNA synthesis kit (Clontech, Palo Alto, CA), a method for the generation of high-quality cDNA from small amounts of RNA. SMART-ds-cDNA was obtained from each 1 µg pooled total RNA of four patients before and after treatment with Advantage KlenTaq polymerase (Clontech; 19 PCR cycles).
Suppression subtractive hybridization (SSH)
To identify differentially expressed clones, cDNAs were spotted on macro-arrays on identical filters that were hybridized with DID-labelled probes that were enriched for cDNAs expressed before treatment. Using this technique, differentially expressed cDNA clones could be clearly identified. For further analyses, samples were enriched by forward subtraction using cDNA fragments from the RNA of PBLs after WBH as tester and before WBH as driver according to the manufacturer's PCR Select protocol (Clontech). The PCR Select Kit is based on the SSH-Technique that has been used in several studies to identify differentially expressed genes Citation[25]. Briefly, PCR-generated ds-cDNAs were digested with Rsa I to generate blunt-ended small cDNA fragments (size distribution 0.2–2 kB). For the first hybridization, two tester populations (duplicates of cDNAs after WBH) with different adaptors (1 + 2R) and driver cDNAs without adaptors (cDNAs before WBH) with a tester:driver ratio of 1:30 were incubated for 8 h at 68°C and for the second hybridization the two tester populations were pooled in the presence of new driver in excess (tester:driver ratio 1:40) and allowed to incubate for another 18 h at 68°C. Differentially expressed cDNAs were then selectively amplified by two suppressive PCRs (first PCR: 27 cycles, second nested PCR: 10 cycles, with 1 µl of the 1:10 diluted 1. PCR product in 25 µl) using primers specific for the two adaptors. Using this technique, only cDNA fragments that were present in greater abundance in the tester (cDNAs after WBH) than in the driver population (cDNAs before WBH) were equipped with both adaptors 1 and 2R. Therefore, only these fragments were exponentially amplified during the final PCR step, leading to an enrichment of the differentially expressed genes. The subtracted two PCR products were used for T/A cloning and generation of hybridization probes. The subtraction efficiency of subtracted in comparison to unsubtracted cDNAs was analysed by PCR with two oligonucleotide primers specific for human glyceraldehyde-3-phosphate dehydrogenase (GAPDH, Clontech).
Cloning
The SSH-derived cDNAs were ligated into a pT-Adv-vector using AdvanTAge™ PCR Cloning Kit (Clontech) and transformed into competent TOP10F′ E. coli bacteria which were cultured on LB agar plates containing kanamycin, IPTG and X-galactose for blue-white screening. White colonies were picked and incubated for 24 h at room temperature in LB medium. Colonies were used as templates for colony PCR. Clones containing inserts were identified by PCR using REDTaq™ DNA polymerase (Sigma-Aldrich, Taufkirchen, Germany) and M13 Primers (sense: 5′-GGC ACC CCA GGC TTT ACA CTT TAT-3′, antisense: 5′-CGG GCC TCT TCG CTA TTA CG-3′). An Eppendorf Mastercycler Gradient (Eppendorf, Hamburg, Germany) was programmed as follows: initial denaturation for 10 min at 94°C to lyse the bacteria; 30 cycles (1 min 94°C for denaturation, 1 min 60°C for annealing, 1 min 72°C for extension); 10 min 72°C for final extension. The samples were then separated by a 2%-agarose gel containing ethidium bromide. Clones yielding a single PCR product were selected for further analysis.
Macro-arrays
PCR-amplified cDNA fragments were denatured with 0.6 M NaOH and spotted onto two identical Hybond N+ nylon membranes (Amersham Pharmacia Biotech, Freiburg, Germany) using a 96 well dot blotter (Schleicher & Schüll, Dassel, Germany). Membranes were neutralized with Tris-HCl, washed with distilled water and cross-linked by UV-Stratalinker 2400 (Stratagene, Amsterdam, Netherlands). Membranes were tested using a probe generated from second suppressive PCR (10 cycles) with cDNAs after WBH as tester and cDNAs before WBH as driver. A second probe was generated with cDNAs before WBH as tester and cDNAs after WBH as driver. The PCR products were purified using a QIAquick PCR Purification Kit (Qiagen) and the adaptors were then removed by digestion with restriction enzyme Rsa I, followed by a second purification step using a Qiaquick PCR Purification Kit. The probes were then digoxigenin (DIG)-labelled using DIG High Prime (Roche, Mannheim, Germany). Membranes were pre-hybridized for 1 h at 37°C with 20 ml of DIG Easy Hyb (Roche). The probe containing DIG Easy Hyb (5 ng probe/ml DIG Easy Hyb) was incubated for 16 h at 37°C. The membranes were washed with 2 × SSC and 0.1% SDS (2 × 15 min) at room temperature, followed by 0.5 × SSC and 0.1% SDS (2 × 30 min) at 68°C. For detection of bound DIG labelled probe anti-DIG-AP F(ab) fragments (Roche) were used. After washing, detection was done with alkaline phosphatase chemiluminescent substrate CSPD (Roche).
DNA sequencing
Results of duplicate blots were scanned densitometrically and the ratio of signals by after-WBH- and before-WBH-probes was calculated. Plasmid DNA of positive clones (>2-fold increased expression) was isolated using a Qiagen Plasmid Mini Kit (Qiagen). Direct sequencing was performed using a BigDye™ Terminator Cycle Sequencing Ready Reaction Kit (Perkin-Elmer/Applied Biosystems, Weiterstadt, Germany) and M13-sense-Primer. Sequencing reaction products were analysed with an ABI 310 sequencer (Perkin-Elmer/Applied Biosystems). Homology searches were performed using BLAST program (http://www.ncbi.nlm.nih.gov).
Semi-quantitative rt-PCR analysis of differentially expressed genes
Oligonucleotide primers were synthesized (TIB Molbiol, Berlin, Germany) on the basis of the mRNA sequence of the obtained sequences encoding for heat shock protein (HSP) 60, HSP90a, HSP90b, HSPp105, calcyclin binding protein (CacyBP), macrophage inflammatory protein-1 beta (MIP-1 beta) and haemoglobin beta chain (HBB). Sequence for cDNA encoding for GAPDH was obtained from Clontech. Sense and anti-sense primers are listed in .
Table I. Genes induced by whole body hyperthermia and chemotherapy in peripheral blood lymphocytes of patients with metastatic adenocarcinomas and the reference gene GAPDH.
Semi-quantitative RT-PCR was performed by normalizing the samples to GAPDH content. Template for PCR was the subtracted cDNA (1:10 dilution, obtained after two suppressive PCR) and cDNA from each patient derived from RNA before and after WBH, reversely transcribed with hexanucleotids, without SMART amplification and SSH procedure. The bands were evaluated densitometrically normalized to GAPDH cDNA. PCR bands were analysed using NIH Image 1.60. Measurements of samples obtained before and after treatment were compared by t-tests for paired samples.
Results
mRNA was isolated from PBLs of four patients with different metastatic adenocarcinomas obtained before and after a combined treatment with WBH and chemotherapy. Analysis of PBLs according to lymphocyte sub-populations revealed a decrease in the number of T4-cells, while numbers of NK-cells increased during treatment (for further details on the course of lymphocate sub-populations during WBH and chemotherapiy see Citation[15]). Using SSH, a cDNA library enriched for cDNAs that were increased after therapy was generated. cDNAs with different signal intensity before and after treatment were identified by macro-arrays and chosen for further evaluation ().
Figure 1. Dot-blot hybridization of cDNA library enriched for cDNAs upregulated in peripheral blood lymphocytes after whole body hyperthermia probed with PBL cDNAs increased after (after WBH) and before whole body hyperthermia combined with chemotherapy (before WBH), respectively. Marked are clones of cDNAs that are increased ((a)1–3), as well as cDNAs with low (b) and high initial signal intensity (c) that are not differentially expressed.
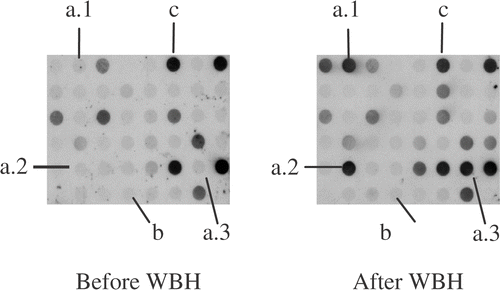
Among 192 clones investigated, 39 cDNAs were found to be significantly upregulated. Sequencing revealed cDNAs of published genes in 37 of 39 cases and the repetitive sequence L1Mc4 in one case. Of the remaining 36 cDNAs, genes of the HSP 90 family were identified in 18 cDNAs (HSP 90 alpha: n = 5, HSP 90 beta: n = 7, HSP 90 alpha homologue: n = 1, HSP 89: n = 5), HSP 60 in five, the gene of the calcyclin binding protein (CacyPP) in five, HSP 105 in four, the gene of the haemoglobin beta chain (HBB) in three and the gene of the macrophage inflammatory protein 1–beta (Mip-1β) in one sample. Among these genes, cDNAs encoding for the heat shock proteins HSP60, HSP90a, HSP90b, HSP105, calcyclin binding protein (CacyBP), macrophage inflammatory protein-1 beta (MIP-1 beta) and haemoglobin beta chain (HBB) were chosen for further analysis (). Due to their constitutional expression in various human tissues and well-known relevance to the physiology of heat application, the cDNAs for HSP27 and HSP70 were also included into this set of genes, although they were not detectable in the SSH libraries (data not shown).
To confirm differential cDNA expression pattern, semi-quantitative RT-PCR was performed. All cDNAs, except for HSP27 and HSP70, were absent or expressed at very low levels in the SMART-ds-cDNA amplifications generated from PBL of all patients before treatment, but strongly expressed in the cDNA amplifications isolated from PBL after therapy ().
Figure 2. Semi-quantitative RT-PCR (sqRT-PCR) with 1:10 diluted second suppressive PCR material as template generated from combined RNAs from all patients, −: before and +: after WBH with simultaneous chemotherapy, M: 100 bp ladder. Shown are identical cycles for every gene tested. Genes induced are shown (HSP60, HSP90a, HSP90b, HSP105, CacyBP, MIP-1 beta, HBB and the house keeping gene GAPDH for control).
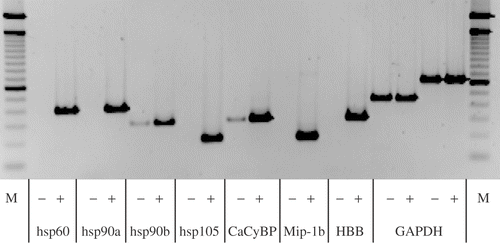
This study further investigated whether the different HSP genes and those of CacyBP, MIP-1 beta and HBB were also differentially expressed in PBL of the individual patients. In all patients the cDNAs were significantly increased after therapy between 2.2-fold (±0.7; HSP70) and 7.8-fold (±2.4; CacyBP; ). For hsp 105, only a statistical trend (p = 0.09) was observed.
Figure 3. Densitometric evaluation of sqRT-PCR with cDNA of four different patients undergoing WBH and chemotherapy (lymphocyte samples before and after treatment). Shown is the relative increase of expression after therapy (×fold ± SD) of HSP27, HSP60, HSP70, HSP90a, HSP90b, HSP105, CacyBP, MIP-1 beta, HBB.
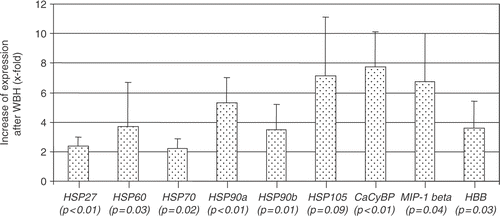
Discussion
The aim of the present report was to identify cDNAs that are upregulated in PBL of patients undergoing combined treatment with WHB and chemotherapy for metastatic solid tumours in the early after-treatment phase by using the SSH-method. To increase the likelihood that cDNA changes were identified caused by WBH itself, blood samples were obtained from patients with different tumour entities treated with different chemotherapeutic agents, which were drawn after patients had been put into general anaesthesia. Furthermore, identical amounts of RNA from these patients before and after therapy, respectively, were combined.
It was found that cDNAs encoding for the heat shock proteins (HSPs) HSP60, HSP90a, HSP90b, HSP105, calcyclin binding protein (CacyBP), macrophage inflammatory protein-1 beta (MIP-1 beta) and haemoglobin beta chain (HBB) were upregulated. HSP27 and HSP70 cDNAs were not detectable in the SSH libraries, but a differential expression of the genes of these heat shock proteins could be detected by using semi-quantitiative rt-PCR.
The HSPs form a group of highly conserved proteins that are induced by heat and by other forms of stress. They constitute at least five sub-groups that differ in molecular mass and, partially, in biologic function Citation[26–28]. Although the precise functions of most HSPs are still unknown, they generally act as molecular chaperones. Some HSPs (HSP 27, HSP 70) are regarded as ‘general survival proteins’ with the property to protect cells from stress-induced apoptosis. Sub-groups of HSPs have also been described to own important immunologic functions, as they may act as antigen-presenting molecules and contribute to the maturation of dendritic cells. Recent findings further suggest that some HSPs interact with antigen presenting cells through the CD 91 receptor, inducing the re-presentation of chaperoned peptides by MHC-molecules, translocation of NF-kappa B into the nuclei and secretion of proinflammatory cytokines Citation[28–30]. In hyperthermia, HSPs are upregulated to protect cells from heat dam Citation[31], Citation[32] and are also involved into the phenomenon of thermotolerance Citation[33]. Solid tumour cell lines have been described in which a heat-inducible form of HSP 70 is expressed on the cell surface, thereby mediating MHC-independent cell-lysis Citation[34]. However, the role of heat-induced HSPs expression in human tissues for clinical hyperthermia still needs to be further defined.
Recent investigations performed concomitant to clinical WBH-trials suggest that WBH may induce a proinflammatory cytokine response and activates NK- and T-cells in cancer patients Citation[2], Citation[15–18]. The finding that WBH combined with chemotherapy induces the upregulation of cDNAs for a series of HSPs in PBL supports the hypothesis that during this treatment modality HSPs may exert a cytoprotective effect for PBL and may be involved into the induction of a proinflammatory cytokine response and NK-cell activation.
The gene encoding for CacyBP was among the cDNAs that were induced at the highest rates in PBL by WBH combined with chemotherapy. To the authors’ knowledge, this is the first report that CacyBP is expressed in PBL and is stimulated in these cells by this treatment. CacyBP functions as a receptor for the calcium-binding protein Calcyclin (S100A6) which belongs to the family of S100 proteins. Calcyclin is a growth-associated gene that is differentially expressed during the cell cycle. It is upregulated in proliferating and differentiating cells Citation[35] and is induced upon transition from G1- to S-phase Citation[36]. Although one did not find the gene for calcyclin among those induced by this therapy, the differential expression of the cDNA encoding for the corresponding receptor, CacyBP, is in line with the hypothesis that simultaneous application of WBH and chemotherapy has a stimulating effect on PBLs.
Surprisingly, this study also detected induction of HBB in the cDNA samples analysed. Under physiological conditions, only erythroid cells are able to synthesize the beta chain of haemoglobin and expression of HBB in normal human lymphocytes has not been reported so far. One hypothesis to explain this result is that HBB is actually expressed by PBLs. Indeed, it had been demonstrated that murine lymphoid Ba/F3 cells could be induced to express HBB upon transfection of the erythropoeitin receptor Citation[37]. Razin et al. Citation[38] reported that cell type specific expression of the alpha globin gene in chicken cells is co-determined by a selective hyper- or under-methylation of a CpG island upstream of the alpha globin gene locus. However, it is still unclear whether HBB actually has a role in lymphocyte biology and which mechanisms regulate its differential expression pattern in these cells. Another explanation is that the detection of HBB-DNA is due to contamination of the PBL fraction by nuclear-containing erythroid progenitors. Unfortunately, this possibility was not raised when the experiments were processed and, thus, this study did not include respective markers into the flow analyses. Therefore, the interpretion of HBB-upregulation observed remains uncertain.
The observation that cDNA encoding MIP-1 beta is upregulated in PBL following WBH and chemotherapy is in line with the known role of MIP-1 beta as a functionally relevant CC chemokine belonging to a group of ‘type 1 cytokines’ Citation[39]. MIP-1 beta is secreted by activated NK cells, CD8+ T and CD4+ T helper 1 (Th1) cells. As a chemoattractant it regulates leukocyte activation and trafficking and acts as coactivator of macrophages Citation[39]. Of note, MIP-1 beta appears to influence control of fever by its ability to induce and to suppress experimental fever Citation[40]. Therefore, one reasonable hypothesis to explain the upregulation of MIP-1 beta during WBH may be a role of its gene products in the physiological reaction induced by an artificial elevation of the body core temperature in humans, whereby further details of this mechanism remain unclear.
There are some caveats when interpreting this differential cDNA expression pattern. Although all patients had already received general anaesthesia (GA) when the first blood sample was obtained, one cannot rule out the possibility that the unspecific stimuli elicited by GA or other parts of the WBH-treatment may have influenced the cDNA alterations described. However, due to the uncertainty about the results to be expected, as well as due to the high costs and efforts associated with every single subtractive hybridization, there was not the possibility to include control groups into this pilot study (e.g. patients undergoing general anaesthesia or cancer patients treated with chemotherapy alone). Therefore, further investigation is warranted to confirm that the gene expression pattern observed in PBLs of these patients is exclusively induced by the WBH procedure. For example, it still needs to be examined whether the observed cDNA changes translate also into induction of the corresponding proteins.
Yet, to the authors’ best knowledge, the current study is the first to define a differential cDNA profile of PBL that is stimulated in patients undergoing combination therapy of WBH and chemotherapy. It is hypothesized that this therapeutic approach elicits cDNA changes in PBL that may be responsible for the cellular integrity, stimulation and migration of immune effector cells. Further studies will address the issue to what extent these molecular mechanisms are actually involved in the therapeutic effects attributed to this experimental modality of cancer treatment.
Acknowledgements
This work was supported by grants from Deutsche Krebshilfe, Deutsche Forschungsgemeinschaft (DFG-SFB 273) and Fritz-Bender Stiftung.
References
- Dewhirst MW, Viglianti BL, Lora-Michiels M, Hanson M, Hoopes PJ. Basic principles of thermal dosimetry and thermal thresholds for tissue damage from hyperthermia. Int J Hyperthermia 2003; 19: 267–294
- Hildebrandt B, Wust P, Ahlers O, Dieing A, Sreenivasa G, Kerner T, Felix R, Riess H. The cellular and molecular basis of hyperthermia. Crit Rev Oncol Hematol 2002; 43: 33–56
- Jaattelä M. Heat shock proteins as cellular lifeguards. Ann Med 1999; 31: 261–271
- Kampinga HH, Dynlacht JR, Dikomey E. Mechanism of radiosensitization by hyperthermia (≥43°C) as derived from studies with DNA repair defective mutant cell lines. Int J Hyperthermia 2004; 20: 131–139
- Lepock JR. Cellular effects of hyperthermia: Relevance to the minimum dose for thermal damage. Int J Hyperthermia 2003; 19: 252–266
- Urano M, Kuroda M, Nishimura Y. For the clinical application of thermochemotherapy given at mild temperatures. Int J Hyperthermia 1999; 15: 79–107
- Repasky E, Issels R. Physiological consequences of hyperthermia: Heat, heat shock proteins and the immune response. Int J Hyperthermia 2002; 18: 486–489
- Li CY, Dewhirst MW. Hyperthermia-regulated immunogene therapy. Int J Hyperthermia 2002; 18: 586–596
- Manjili MH, Wang XY, Park J, Macdonald IJ, Li Y, Van Schie RC, Subjeck JR. Cancer immunotherapy: Stress proteins and hyperthermia. Int J Hyperthermia 2002; 18: 506–520
- Hammami MM, Bouchama A, Shail E, Aboul Enein HY, al Sedairy S. Lymphocyte subsets and adhesion molecules expression in heatstroke and heat stress. J Appl Physiol 1998; 84: 1615–1621
- Blazickova S, Rovensky J, Koska J, Vigas M. Effect of hyperthermic water bath on parameters of cellular immunity. Int J Clin Pharmacol Res 2000; 20: 41–46
- Downing JF, Taylor MW. The effect of in vivo hyperthermia on selected cytokines in men. Lymphokine Res 1987; 6: 103–109
- Kappel M, Stadeager C, Tvede N, Galbo H, Pedersen BK. Effects of in vivo hyperthermia on natural killer cell activity, in vitro proliferative responses and blood mononuclear cell subpopulations. Clin Exp Immunol 1991; 84: 175–180
- Kraybill WG, Olenki T, Evans SS, Ostberg JR, O’Leary KA, Gibbs JF, Repasky EA. A phase I study of fever-range whole body hyperthermia (FR-WBH) in patients with advanced solid tumours: Correlation with mouse models. Int J Hyperthermia 2002; 18: 253–266
- Ahlers O, Hildebrandt B, Dieing A, Deja M, Bohnke T, Wust P, Riess H, Gerlach H, Kerner T. Stress induced changes in lymphocyte subpopulations and associated cytokines during whole body hyperthermia of 41.8–42.2°C. Eur J Appl Physiol 2005; 95: 298–306
- Atanackovic D, Nierhaus A, Neumeier M, Hossfeld DK, Hegewisch-Becker S. 41.8°C whole body hyperthermia as an adjunct to chemotherapy induces prolonged T cell activation in patients with various malignant diseases. Cancer Immunol Immunother 2002; 51: 603–613
- Katschinski D, Wiedemann GJ, Longo W, d’Oleire FR, Spriggs D, Robins HI. Whole body hyperthermia cytokine induction: A review, and unifying hypothesis for myeloprotection in the setting of cytotoxic therapy. Cytokine Growth Factor Rev 1999; 10: 93–97
- Robins HI, Kutz M, Wiedemann GJ, Katschinski DM, Paul D, Grosen E, Tiggelaar CL, Spriggs D, Gillis W, D’Oleire F. Cytokine induction by 41.8°C whole body hyperthermia. Cancer Lett 1995; 97: 195–201
- Hildebrandt B, Drager J, Kerner T, Deja M, Loffel J, Stroszczynski C, Ahlers O, Felix R, Riess H, Wust P. Whole-body hyperthermia in the scope of von Ardenne's systemic cancer multistep therapy (sCMT) combined with chemotherapy in patients with metastatic colorectal cancer: A phase I/II study. Int J Hyperthermia 2004; 20: 317–333
- Hegewisch-Becker S, Gruber Y, Corovic A, Pichlmeier U, Atanackovic D, Nierhaus A, Hossfeld DK. Whole-body hyperthermia (41.8°C) combined with bimonthly oxaliplatin, high-dose leucovorin and 5-fluorouracil 48-hour continuous infusion in pretreated metastatic colorectal cancer: A phase II study. Ann Oncol 2002; 13: 1197–1204
- Strobl B, Janni W, Rjosk D, Rack BK, Kornya L, Bakhshandeh-Bath A, Hegewisch-Becker S, Hildebrandt B, Sommer HL. Chemotherapy with carboplatin/ifosfamide combined with whole body hyperthermia in recurrent ovarian cancer—results of a phase II study (Dolphin-1-Study). Proc Am Soc Clin Oncol 2002; 19: 2523
- Hildebrandt B, Hegewisch-Becker S, Kerner T, Nierhaus A, Bakhshandeh-Bath A, Janni W, Sommer H, Riess H, Wust P. Current status of radiant whole-body hyperthermia at temperatures >41.5. Int J Hyperthermia 2005; 21: 169–183
- Kerner T, Deja M, Ahlers O, Loeffel J, Hildebrandt B, Wust P, Gerlach H, Riess H. Whole body hyperthermia: A secure procedure for patients with various malignancies?. Intensive Care Med 1999; 25: 959–965
- Kerner T, Hildebrandt B, Ahlers O, Deja M, Riess H, Draeger J, Wust P, Gerlach H. Anaesthesiological experiences with whole body hyperthermia. Int J Hyperthermia 2003; 19: 1–12
- Diatchenko L, Lau YF, Campbell AP, Chenchik A, Moqadam F, Huang B, Lukyanov S, Lukyanov K, Gurskaya N, Sverdlov ED, et al. Suppression subtractive hybridization: A method for generating differentially regulated or tissue-specific cDNA probes and libraries. Proc Natl Acad Sci USA 1996; 93: 6025–6030
- Buchner J. Supervising the fold: Functional principles of molecular chaperones. FASEB J 1996; 10: 10–19
- Garrido C, Gurbuxani S, Ravagnan L, Kroemer G. Heat shock proteins: Endogenous modulators of apoptotic cell death. Biochem Biophys Res Comm 2001; 286: 433–442
- Srivastava P. Roles of heat-shock proteins in innate and adaptive immunity. Nat Rev Immunol 2002; 2: 185–194
- Menoret A, Chandawarkar R. Heat-shock protein-based anticancer immunotherapy: An idea whose time has come. Semin Oncol 1998; 25: 654–660
- Tamura Y, Peng P, Liu K, Daou M, Srivastava PK. Immunotherapy of tumors with autologous tumor-derived heat shock protein preparations. Science 1997; 278: 117–120
- Narita N, Noda I, Ohtsubo T, Fujieda S, Tokuriki M, Saito T, Saito H. Analysis of heat-shock related gene expression in head-and-neck cancer using cDNA arrays. Int J Radiat Oncol Biol Phys 2002; 53: 190–196
- Zhang HJ, Drake VJ, Morrison JP, Oberley LW, Kregel KC. Selected contribution: Differential expression of stress-related genes with aging and hyperthermia. J Appl Physiol 2002; 92: 1762–1769, discussion 1749
- Kregel KC. Heat shock proteins: Modifying factors in physiological stress response and aquired thermotolerance. J Appl Physiol 2002; 92: 2177–2186
- Multhoff G. Activation of natural killer cells by heat shock protein 70. Int J Hyperthermia 2002; 18: 576–585
- Guo XJ, Chambers AF, Parfett CL, Waterhouse P, Murphy LC, Reid RE, Craig AM, Edwards DR, Denhardt DTI. Dentification of a serum-inducible messenger RNA (5B10) as the mouse homologue of calcyclin: Tissue distribution and expression in metastatic, ras-transformed NIH 3T3 cells. Cell Growth Differ 1990; 1: 333–338
- Rossi AM, Hirschhorn RR. Expression of growth-regulated genes in normal and SV40 transformed hamster fibroblasts. J Cell Biochem 1991; 47: 165–173
- Chiba T, Nagata Y, Kishi A, Sakamaki K, Miyajima A, Yamamoto M, Engel JD, Todokoro K. Induction of erythroid-specific gene expression in lymphoid cells. Proc Natl Acad Sci USA 1993; 90: 11593–11597
- Razin SV, Ioudinkova ES, Scherrer K. Extensive methylation of a part of the CpG island located 3.0–4.5 kbp upstream to the chicken alpha-globin gene cluster may contribute to silencing the globin genes in non-erythroid cells. J Mol Biol 2000; 299: 845–852
- Dorner BG, Scheffold A, Rolph MS, Huser MB, Kaufmann SH, Radbruch A, Flesch IE, Kroczek RA. MIP-1alpha, MIP-1beta, RANTES, and ATAC/lymphotactin function together with IFN-gamma as type 1 cytokines. Proc Natl Acad Sci USA 2002; 99: 6181–6186
- Minano FJ, Fernandez-Alonso A, Myers RD, Sancibrian M. Hypothalamic interaction between macrophage inflammatory protein-1 alpha (MIP-1 alpha) and MIP-1 beta in rats: A new level for fever control?. J Physiol 1996; 491: 209–217