Abstract
Purpose: To investigate the treatment-related morbidity and quality of life (QoL) during thermotherapy using superparamagnetic nanoparticles in patients with locally recurrent prostate cancer.
Materials and Methods: Ten patients with biopsy-proven locally recurrent prostate cancer following primary therapy with curative intent and no detectable metastases were entered on a prospective phase I trial. Endpoints were feasibility, toxicity and QoL. Following intraprostatic injection of a nanoparticle dispersion, six thermal therapy sessions of 60 min duration were delivered at weekly intervals using an alternating magnetic field. National Cancer Institute (NCI) common toxicity criteria (CTC) and the European Organization for Research and Treatment of Cancer (EORTC) QLQ-C30 and QLQ-PR25 questionnaires were used to evaluate toxicity and QoL, respectively. In addition, prostate specific antigen (PSA) measurements were carried out.
Results: Maximum temperatures up to 55°C were achieved in the prostates at 25–30% of the available magnetic field strength. Nanoparticle deposits were detectable in the prostates one year after thermal therapy. At a median follow-up of 17.5 months (3–24), no systemic toxicity was observed. Acute urinary retention occurred in four patients with previous history of urethral stricture. Treatment-related morbidity was moderate and QoL was only temporarily impaired. Prostate-specific antigen (PSA) declines were observed in eight patients.
Conclusions: Interstitial heating using magnetic nanoparticles was feasible and well tolerated in patients with locally recurrent prostate cancer. Deposition of nanoparticles in the prostate was highly durable. Further refinement of the technique is necessary to allow application of higher magnetic field strengths.
Introduction
Thermal therapies have been evaluated extensively for both hyperplastic and malignant conditions of the prostate during the last few decades Citation[1]. While thermoablative temperatures (>45°C) can cause direct cell death and are suitable for monotherapy of benign prostatic enlargement and prostate cancer, hyperthermic temperatures between 40°C and 45°C are generally being used in conjunction with irradiation to treat cancer, taking advantage of the known synergism between these two modalities Citation[2]. Clinical hyperthermia of prostate cancer is complex and has been hampered by technical difficulties related to the anatomical position and the high perfusion of this organ. Several techniques have been used for regional hyperthermia of prostate cancer, including radiofrequency, microwave or ultrasound applicators Citation[1]. Effective temperatures achieved in the prostate with these techniques were often limited by electric field elevation at electrical boundaries. A minimally invasive approach, in which a fluid containing magnetic nanoparticles (magnetic fluid) is injected directly into superficial or deep-seated tumors, was developed for interstitial thermotherapy Citation[3]. In vitro studies have shown the excellent power absorption characteristics of magnetic fluids in an alternating magnetic field Citation[4]. The feasibility and efficacy of magnetic nanoparticle thermotherapy has been demonstrated in preclinical studies Citation[5–7]. We conducted a phase I study using this technology in patients with locally recurrent prostate cancer. Treatment options for this stage include androgen deprivation, salvage radical prostatectomy, salvage brachytherapy and cryotherapy Citation[8]. While radical prostatectomy may offer the highest potential for cure, it is not suitable for all patients with recurrent disease. The current study aimed to investigate the feasibility and morbidity of magnetic nanoparticle thermotherapy and to evaluate changes in quality of life (QoL). Feasibility was defined as the ability to attain at least hyperthermic temperatures in the prostates at the maximum field strength tolerated for 60 min without signs of toxicity and to achieve sufficiently durable interstitial deposition and homogeneous distribution of nanoparticles in the prostates to allow for six thermal treatments at weekly intervals.
Methods
Patients
Ten patients with biopsy-proven locally recurrent prostate cancer were enrolled on a prospective phase I trial. Three patients had a local recurrence following radical or suprapubic prostatectomy and irradiation, the other patients had radio-recurrent disease, which was androgen-independent in two cases and hormone-refractory in one case. Patients were either not suitable for or refused salvage radical prostatectomy. Endpoints were feasibility, toxicity and quality of life. The study protocol was approved by the local ethical committee and the trial was conducted according to the principles of the latest version of the Declaration of Helsinki and the guidelines for Good Clinical Practice. Inclusion criteria were histological evidence of local recurrence of prostate cancer following therapy with curative intent, a serum prostate-specific antigen (PSA)-value < 20 ng/ml, ECOG performance status of 0–1 and written informed consent. Exclusion criteria were evidence of systemic disease in computed tomography (CT), magnetic resonance imaging (MRI) and bone scan, the presence of secondary malignancies other than well-controlled squamous cell carcinoma of the skin, metal implants such as hip prosthesis or metallic foreign bodies located less than 30 cm distance from the prostate, chronic inflammatory diseases of the rectum and symptomatic bladder outlet obstruction or significant voiding disorders. Patient characteristics are provided in .
Table I. Patient characteristics.
Thermal treatment and follow-up
The nanoparticles used in this study had an average core size of 15 nm and were coated with an aminosilane-type shell in water (MFL AS, MagForce Nanotechnologies AG, Berlin, Germany). A detailed description of treatment planning based on thin-sliced (2.5 mm) CT-scans of the prostate, the procedure of transperineal injection of magnetic fluid into the prostate, thermal therapy parameters as well as invasive and non-invasive thermometry is given elsewh Citation[9], Citation[10]. In brief, the nanoparticle dispersion was injected transperineally into the prostates under general anesthesia and transrectal ultrasound (TRUS)/fluoroscopy guidance with the patients in lithotomy position. Closed-end catheters were placed in the prostate for invasive thermometry during the first and last thermotherapy session (median number of measurement points 28 [11–35]). Intraluminal thermometry in the urethra and in the rectum was carried out during each treatment. Native CT-scans were repeated after nanoparticle injection to visualize the distribution of nanoparticles in the prostates and allow for temperature calculations Citation[9]. Non-invasive temperature calculations in the prostates, derived from nanoparticle volume and density in CT as well as the SAR of the magnetic fluid at a given magnetic field strength, were correlated with direct temperature mappings in defined measurement points to estimate the three-dimensional intraprostatic temperature distribution in each patient Citation[9]. Six thermotherapy sessions were delivered at weekly intervals with the first magnetic field applicator for use in humans (MFH300F, MagForce Nanotechnologies AG, Berlin), using an alternating magnetic field with a frequency of 100 kHz and a variable field strength (2.5–18 kA/m). Blood pressure, pulse rate and oxygen saturation were recorded every 15 min.
The follow-up schedule included outpatient visits at three and six months post-treatment for physical examination, abdominal and transrectal ultrasound, prostate-specific antigen (PSA) and routine blood tests as well as patient reported QoL. In case of documented biochemical progression, imaging studies were repeated and a prostate biopsy was obtained, unless systemic progression was observed.
Assessment of toxicity and quality of life
National Cancer Institute (NCI) common toxicity criteria (CTC, version 2.0) were used to evaluate treatment-related morbidity during the treatment period of six weeks and follow-up visits. Early toxicity was defined as occuring during the treatment period of six weeks and up to three months follow-up and late toxicity thereafter. For the evaluation of changes in QoL, the European Organization for Research and Treatment of Cancer (EORTC) QLQ-C30, version 3.0, and QLQ-PR25 questionnaires were used. The EORTC QLQ-C30 core questionnaire contains five functional scales (physical, role, emotional, cognitive and social functioning), a global QoL scale, three symptom scales (nausea and vomiting, fatigue, pain) and six single items (dyspnea, insomnia, appetite loss, constipation, diarrhea and financial difficulties) Citation[11]. The EORTC QLQ-PR25 prostate cancer-specific module contains five symptom scales (urinary symptoms/problems, bowel symptoms/problems, treatment-related symptoms, sexual functioning and sexual activity) Citation[12]. The raw scores, representing the average score of items included in each scale, were transformed to a scale score ranging from 0 to 100. For functional scales and the global QoL scale, higher scores represent a higher level of functioning or global QoL. For symptom scales and single items, higher scores indicate a higher level of symptoms or problems. QoL changes of 10 points or more are considered clinically relevant Citation[13]. The questionnaires were compiled by the patients before thermotherapy, at mid-treatment (third week), at the end of treatment (week six), three months and six months after heat treatment. The QoL evaluation at six months of patient 10 is missing due to short follow-up. This patient had experienced almost no toxicity and no significant deterioration of QoL at any of the previous timepoints. For the analysis, this case was handled as missing data and the method of imputation (latest score taken forward) was used.
Statistical analysis
The program GraphPad Prism, version 4.03 for Windows, was used for statistical analysis (GraphPad Software, San Diego, California, USA). Analysis of variance for repeated measures (ANOVA, Dunnett's multiple comparison test) was used for comparison of the means of all variables in the two questionnaires at different time points versus baseline. Regarding changes of each parameter over time, the means of intraindividual differences are given. Differences of p < 0.05 were considered to be statistically significant.
Results
Feasibility and temperatures
The procedures of intraprostatic magnetic fluid injection into the prostate and subsequent thermal therapy were feasible in all patients. However, homogeneous distribution of magnetic fluid in pre-irradiated prostate tissue was difficult to achieve. One patient received five thermotherapy sessions, all other patients underwent six treatments as planned. Maximum temperatures up to 55°C were achieved in the prostates at 25–30% of the available magnetic field strength. The median T20, T50 and T90 in the prostates were 41.1 (40.0–47.4), 40.8 (39.5–45.4) and 40.1°C (38.8–43.4), respectively. Median urethral and rectal temperatures were 40.5°C (38.4–43.6) and 39.8°C (38.2–43.4). A satisfactory correlation between invasive measurements and non-invasive temperature calculations was found in the prostates, as described in a separate report Citation[9]. The median calculated thermal dose derived from the fit of invasive and non-invasive measurements was CEM 43°C T90 = 7.8 min (3.5–136.4, mean: 20.9 min).
Median follow-up is 17.5 months (3–24). Deposition of nanoparticles in the prostates was found to be extremely durable. Four patients underwent control imaging and biopsy of the prostate one year post-thermotherapy. In the CT images, nanoparticle deposits in the prostates were still clearly visible (, left). Nanoparticles could also be detected histologically in these patients (, right). However, repeated heating was not attempted.
Figure 1. Example of an unenhanced computed tomography scan obtained one year after a single injection of magnetic fluid into the prostate, followed by six thermotherapy sessions (left). Hyperdense nanoparticle deposits in the prostate are still clearly visible. Histology image obtained by prostate biopsy of the same patient 1 year after treatment (right). Iron-oxide nanoparticles (brown) are still present in the prostate tissue (hematoxylin-eosin staining, ×200).
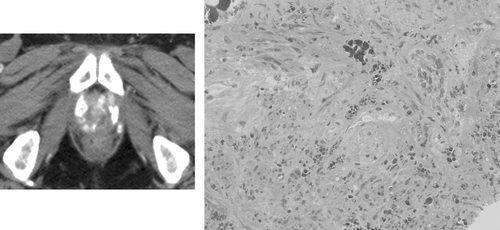
Morbidity
Alternating magnetic field strengths of 4–5 kA/m were tolerated throughout the treatment time in all patients. Minor rise of pulse rate and blood pressure were recorded in some patients towards the end of thermal treatments. Higher magnetic field strengths caused discomfort in the groin or perineal region. In some patients, temperature maxima of up to 44°C were observed at the skin level, typically in folds of the scrotal and anal region. Both undesired effects could be managed by cooling, wiping off sweat from the skin, ventilation and shielding, using salt water-filled pads. While the intensity of pain in both of these anatomical regions correlated with increasing intensity of the magnetic field during thermal therapy, there was no direct correlation of this toxicity with temperatures achieved in the prostates. We observed interindividual and also intraindividual differences with regard to the occurrence pain during heat treatments, which could not be related to an absolute threshold of magnetic field strength, but depended critically on correct positioning of the cooling device and on whether the skin was kept dry. No systemic toxicity was observed. Temporary bladder drainage with a transurethral or suprapubic catheter for 2–4 weeks due to acute urinary retention was necessary in four patients (all with previous history of urethral stricture/impaired urinary flow rate following radiation therapy). All of these patients have resumed normal micturition. One patient subsequently experienced worsening urinary urge and frequency due to a bladder neck contraction, while urethra and prostate showed no pathological findings. Following bladder neck incision, his symptoms were immediately relieved. Grade 3 urinary toxicity was noted in two patients, with both bladder spasms and urinary frequency grade 3 in one patient and bladder spasms grade 3 and urinary frequency grade 2 in the other. In both cases, grade 3 side effects were observed only following magnetic nanoparticle injection and subsequent first thermal treatment. Dysuria grade 2 was present in two and grade 1 in three patients. A mild erythema of the groin in one patient was treated with ointments and disappeared during the further course of thermotherapy. In one patient, a febrile urinary tract infection required antibiotic treatment. A CT-scan of the pelvis in this patient showed a small tissue reaction without enhancing nanoparticles in the upper thigh. Analysis of a small aspirate from this lesion showed some inflammatory cells, no tumor cells and no nanoparticles. The patient was symptom-free following one week of antibiotic treatment, but the last thermotherapy session was omitted. One patient had a stress urinary incontinence grade 1 before thermotherapy, which remained unchanged after treatment. Late treatment-related morbidity was not observed in our study so far.
Quality of life
Changes in QoL during and after magnetic nanoparticle thermotherapy in comparison with the baseline status are illustrated in . There was no significant deterioration of physical functioning, global health status and treatment-related symptoms during the study. A significant deterioration of several items was observed, such as social functioning (p < 0.0001, at all timepoints), role functioning (p = 0.0008, weeks 3–6), fatigue (p = 0.0154, week 3), pain (p = 0.0081, week 3), financial difficulties (p = 0.0022, week 6), urinary symptoms (p = 0.0445, week 3) and sexual functioning (p = 0.0096, week 6). Thus, all parameters returned to pre-treatment level 6 weeks or 3 months after thermal therapy, apart from social functioning which remained impaired. Two patients complained of worsening erectile dysfunction following thermotherapy and received treatment with phosphodiesterase-5-inhibitors.
Table II. Evaluation of changes in quality of life (QoL) over time. Measurements were carried out before thermotherapy (baseline), at mid-treatment (week 3), at the end of thermotherapy (week 6) and after 3 and 6 months.
Clinical outcome
Hormonal therapy was discontinued in patients 1, 6, 7 and 10 at least 2 months prior to the beginning of thermal therapy to exclude artifactual low baseline PSA. The remaining (patients 3, 8 and 9) had hormone-independent disease. A PSA decline was observed in eight patients at the end of treatment. A decrease of >70% occurred in patient 9, a decrease of 40% in patient 10 and a decrease of ≤25% in the remaining patients. Mean duration of PSA-control, defined as the time to increase beyond the level before thermotherapy, was 4.5 months (3–6). Of the two patients with no PSA response, one had a suboptimal magnetic fluid coverage of a small local recurrence and the other had probably pre-existing metastatic disease. Three patients were found to have distant disease during the follow-up. Four patients had positive prostate biopsies one year post-thermotherapy and started salvage irradiation or androgen deprivation. Two patients with a rising PSA refused prostate biopsy and started further therapy. One patient was lost to follow-up after the six months-visit and one patient has an ongoing PSA response.
Discussion
Clinical hyperthermia of prostate cancer remains a challenging problem. The high incidence of this disease and the trend towards minimally-invasive and less radical therapies along with easy accessibility for such procedures would make thermotherapy or thermoradiotherapy seem attractive treatment options. A biological rationale for thermoradiotherapy of prostate cancer has been demonstrated in vitro and in vivo Citation[14]. However, due to the long natural course of this disease, a benefit of hyperthermia in terms of a radiation-enhancing effect is difficult to demonstrate. Due to the anatomical position of the prostate, external application of a thermal treatment is problematic: The prostate is shielded anteriorly by fat and bone and surrounded posteriorly and cranially by hollow organs containing air and fluid. The different conductivities of these tissues to radiofrequency or ultrasound waves applied for heating purposes may cause undesired reflexion, scattering or absorption of thermal energy outside the target area in critical regions, while the high intra- and periprostatic perfusion may act as a heat sink. All these phenomena have limited achievable temperatures in the prostate with conventional heating techniques Citation[15–20]. As a result, none of the hyperthermia applicator techniques developed so far has been used for an evaluation of thermoradiotherapy of prostate cancer in the framework of phase III studies. Instead, the optimization of existing equipment as well as the search for new technologies offering better heating characteristics for this deep-seated organ is ongoing Citation[1].
In the current study, we demonstrated that magnetic nanoparticle thermotherapy is feasible and that hyperthermic to thermoablative temperatures can be achieved in the prostates at relatively low field strengths Citation[9]. Alternating magnetic field strengths of 4–5 kA/m were tolerated throughout the treatment time. Higher field strengths caused local discomfort. The origin of these complaints is not completely clear, but could be attributed to two distinct phenomena:
Firstly, boundary effects between tissues of different dielectric constants and conductivity, well known and much more pronounced in E-fields during radiofrequency hyperthermia, may also be present to a lesser degree in H fields and account for the dull pain without any visible skin reaction. This could occur at bone surfaces and possibly also in soft tissues. The patient with the inflammatory tissue reaction in the groin had reported similar symptoms during thermotherapy, possibly caused by such boundary effects.
Secondly, the current path narrows in skin folds and therefore, current density increases and may lead to relative hot spots, potentially causing skin reactions. In magnetic nanoparticle thermotherapy, they were only observed during the treatment of pelvic tumors, where the cross-section of the body is relatively high. In fact, tolerated magnetic field strengths during magnetic nanoparticle thermotherapy of brain tumors were much higher, e.g. 10–14 kA/m Citation[21]. Since field strengths of up to 18 kA/m can be applied with the applicator used in this study and given the quadratic increase of SAR with increasing magnetic field strength, significantly higher temperatures could be achieved with this technique. Also, by increasing homogeneity of intraprostatic nanoparticle distribution, lower temperature gradients and higher minimal temperatures in the target region would be attainable.
Treatment-related early morbidity was moderate and manageable in our study. Significant urinary symptoms almost exclusively appeared during the first three weeks of treatment. At later timepoints, only treatment-related pain occurred and most patients were symptom-free during the interval between the thermotherapy sessions. However, the follow-up is currently too short to exclude late toxicity.
QoL was only temporarily impaired by magnetic nanoparticle thermotherapy. Because of the small number of patients in our study, these results must be interpreted with caution, since changes in single patients may have had a large impact on the overall results, potentially overstating the effect on the whole group. Thus, the power to detect true differences in QoL may be limited in this small series of patients and larger studies would be required to validate these results. Only one previous prospective study investigating QoL in prostate cancer patients treated with hyperthermia and irradiation has been published Citation[22]. Van Vulpen and co-workers analyzed the influence of hyperthermia, delivered externally (in 8 patients) or interstitially (in 12 patients) using a radiofrequency device, on QoL during conventional external radiotherapy. This thermoradiotherapy group was compared to 58 patients with prostate cancer in whom only external irradiation had been administered. They found that a temporary deterioration of social, psychological and disease-related symptoms occurred in all patients with no worse outcome of patients receiving hyperthermia. There was also no difference in QoL between patients receiving external and interstitial hyperthermia.
Regarding oncological outcome, PSA declines following thermotherapy were observed in our current study, which suggest potential efficacy of this treatment. However, these responses were of limited extent and duration. Due to several limitations, a meaningful interpretation of oncological efficacy is not possible in our current phase I study. Firstly, the time interval between discontinuation of hormonal therapy and the beginning of thermal therapy may not have been sufficiently long for the normalization of PSA in two patients. Secondly, the patient cohort was heterogeneous with many patients having undergone multiple local and systemic pre-treatments and most patients presented with unfavourable prognostic features. There appeared to be a correlation between higher temperatures, homogeneous intraprostatic distribution of nanoparticles and PSA decline, observed in later patients of our series Citation[9].
In patient 2 of our series, who had a PSA of 40.7 ng/ml at diagnosis and a Gleason score of 4 + 3 in all biopsy cores prior to thermotherapy, distant metastasis in a hilar lymph node measuring 3.4 cm in diameter was detected nine months after thermotherapy. A bone scan, X-ray and CT of the thoracic spine, but no chest X-ray had been performed previously in this case. The lesion was confirmed to be a prostate cancer metastasis by CT-guided biopsy and histology. The unusual metastatic site in this case caused concern regarding a possible contribution of thermal therapy to spreading or growth of the lesion. There have been a few reports of increased metastases in animal models, mostly after whole-body hyperthermia, but also after local heating in a prostate cancer model Citation[23]. However, there is also experimental data showing no evidence of such phenomena in hyperthermia and thermoablation of prostate canc Citation[24], Citation[25]. To our knowledge, reports of increased metastases in humans undergoing thermal treatments have not been published. In our case, a heat-induced metastasis seems unlikely, given the short time interval and the size of the lesion. Moreover, the doubling of PSA within three months despite thermal therapy (from 6.4 to 12.4 ng/ml) would suggest pre-existing metastatic disease. One way to circumvent the unproven but potential problem of heat-induced metastasis in clinical thermoradiotherapy is to administer a few fractions of radiotherapy before starting hyperthermia.
A number of thermal ablation techniques have been employed as salvage therapies for local failure following irradiation of prostate cancer, namely interstitial microwave thermal therapy, radiofrequency interstitial tumor ablation (RITA), transrectal high-intensity focused ultrasonography (HIFU) and ferromagnetic self-regulating thermoseeds Citation[26–29]. Significant PSA decreases and cancer-free biopsy rates up to 80% after therapy were reported with these techniques. However, additional endocrine treatment was necessary in 56% of patients undergoing HIFU and 50% of patients receiving RITA had positive biopsies one year after therapy.
Thermal therapy using magnetic nanoparticles may offer several advantages over conventional and also other magnetically induced heating techniques: Theoretically, this method allows for contactless, selective heating of deep-seated tumours. The large number and overall surface of magnetic elements within magnetic fluids result in excellent power absorption capabilities, far superior to larger metallic implants such as seeds Citation[4]. The results of our study as well as animal experiments performed previously suggest that our technique may in principle be suitable for thermal ablation as a monotherapy or hyperthermia in combination with radiotherapy of prostate canc Citation[7], Citation[9]. However, using the currently available equipment for magnetic nanoparticle thermotherapy of prostate cancer, the application of sufficiently high magnetic field strengths to achieve thermoablative temperatures may cause unspecific heating outside the target volume in a proportion of patients as well as local discomfort during thermal treatments, which are limiting factors. Besides, intratumoral distribution of nanoparticles is still suboptimal at present. On the other hand, deposition of nanoparticles in the prostates was found to be highly durable. Further approaches are currently being explored by our group to increase the performance of the overall technology.
At present there is no system available neither for direct magnetic fluid injection under real-time visual control, nor for reliable imaging of cancer within the prostate. Thus, selective ablation of prostate cancer while sparing normal tissue is not possible at present. With improved diagnostic imaging methods, this may become an interesting issue in the future. However, since for the time being average temperatures achieved in the prostates with our technique are hyperthermic, treatment of the whole prostate volume, together with irradiation, should be further evaluated.
Conclusions
Interstitial heating using magnetic nanoparticles was feasible in patients with previously irradiated and locally recurrent prostate cancer. Treatment-related toxicity was moderate and QoL was only temporarily impaired. Until the method will be more refined and higher magnetic field strengths can safely be applied to yield thermal ablation as a monotherapy, this treatment modality may be evaluated in combination with irradiation in patients with localized prostate cancer.
Acknowledgements
This study was supported by the EFRE Project “NanoMed”, Nanotechnology in Medicine, No 2000–22006 2ue/2 and in part by the Lieselotte-Beutel Foundation (project prostate center). The authors thank Lara Eckelt, Eva Wasserberg, Cornelia Cordes, Sabine Müller, Young-Suk Frisch and Dipl. Ing. V. Brüß, for valuable technical assistance and Dr Florian Fritzsche, Department of Pathology, Charité-Universitätsmedizin Berlin, for the micrographs obtained from prostate biopsies.
References
- Stauffer PR. Evolving technology for thermal therapy of cancer. Int J Hyperthermia 2005; 21: 731–744
- Hildebrandt B, Wust P, Ahlers O, Dieing A, Sreenivasa G, Kerner T, Felix R, Riess H. The cellular and molecular basis of hyperthermia. Crit Rev Oncol Hematol 2002; 43: 33–56
- Jordan A, Maier-Hauff K, Wust P, Johannsen M. Nanoparticles for thermotherapy. Nanomaterials for cancer therapy, CSSR Kumar. Wiley-VCH, Weinheim 2006; 242–258
- Jordan A, Wust P, Fähling H, John W, Hinz A, Felix R. Inductive heating of ferrimagnetic particles and magnetic fluids: Physical evaluation of their potential for hyperthermia. Int J Hyperthermia 1993; 9(1)51–68
- Johannsen M, Jordan A, Scholz R, Lein M, Koch M, Deger S, Roigas J, Jung K, Loening SA. Evaluation of magnetic fluid hyperthermia in a standard rat model of prostate cancer. J Endourol 2004; 18: 495–500
- Johannsen M, Thiesen B, Jordan A, Taymoorian K, Gneveckow U, Waldöfner N, Scholz R, Koch M, Lein M, Jung K, et al. Magnetic fluid hyperthermia (MFH) reduces prostate cancer growth in the orthotopic Dunning R3327 rat model. Prostate 2005; 64(1)283–92
- Johannsen M, Thiesen B, Gneveckow U, Taymoorian K, Waldöfner N, Scholz R, Deger S, Jung K, Loening SA, Jordan A. Thermotherapy using magnetic nanoparticles combined with external radiation in an orthotopic rat model of prostate cancer. Prostate 2006; 66(1)97–104
- Touma NJ, Izawa JI, Chin JL. Current status of local salvage therapies following radiation failure for prostate cancer. J Urol 2005; 173: 373–379
- Johannsen M, Gneveckow U, Thiesen B, Taymoorian K, Cho CH, Waldöfner N, Scholz R, Jordan A, Loening SA, Wust P. Thermotherapy of prostate cancer using magnetic nanoparticles: Feasibility, imaging and three-dimensional temperature distribution. Eur Urol Nov 17, 2006, (Epub ahead of print)
- Johannsen M, Gneveckow U, Eckelt L, Feussner A, Waldöfner N, Scholz R, Deger S, Wust P, Loening SA, Jordan A. Clinical hyperthermia of prostate cancer using magnetic nanoparticles: Presentation of a new interstitial technique. Int J Hyperthermia 2005; 21: 637–647
- Aaronson NK, Ahmedzai S, Bergmann B, Bullinger M, Cull A, Duez NJ, Filiberti A, Flechtner H, Fleishman SB, de Haes JC. The European Organization for Research and Treatment of Cancer (EORTC) QLQ-C30: A quality-of-life instrument for use in international clinical trials in oncology. J Natl Cancer Inst 1993; 85: 365–376
- Borghede G, Sullivan M. Measurement of quality of life in localized prostatic cancer patients treated with radiotherapy. Development of a prostate cancer-specific module supplementing the EORTC QLQ-C30. Qual Life Res 1996; 5: 212–222
- Osoba D, Rodrigues G, Myles J, Zee B, Pater J. Interpreting the significance of changes in health-related quality-of-life scores. J Clin Oncol 1998; 16: 139–144
- Ryu S, Brown SL, Khil MS, Kim SH, Kim JH. Preferential radiosensitization of human prostatic carcinoma cells by mild hyperthermia. Int J Radiat Oncol Biol Phys 1996; 34: 133–138
- Van Vulpen M, De Leeuw AA, Raaymakers BW, Van Moorselaar RJ, Hofman P, Lagendijk JJ, Battermann JJ. Radiotherapy and hyperthermia in the treatment of patients with locally advanced prostate cancer: Preliminary results. BJU Int 2004; 93: 36–41
- Anscher MS, Samulski TV, Dodge R, Prosnitz LR, Dewhirst MW. Combined external beam irradiation and external regional hyperthermia for locally advanced adenocarcinoma of the prostate. Int J Radiat Oncol Biol Phys 1997; 37: 1059–1065
- Hurwitz MD, Kaplan ID, Hansen JL, Prokopios-Davos S, Topulos GP, Wishnow K, Manola J, Bornstein BA, Hynynen K. Hyperthermia combined with radiation in treatment of lovally advanced prostate cancer is associated with a favourable toxicity profile. Int J Hyperthermia 2005; 21: 649–656
- Algan O, Fosmire H, Hynynen K, Dalkin B, Cui H, Drach G, Stea B, Cassady JR. External beam radiotherapy and hyperthermia in the treatment of patients with locally advanced prostate carcinoma. Cancer 2000; 89: 399–403
- Kalapurakal JA, Pierce M, Chen A, Sathiaseelan V. Efficacy of irradiation and external hyperthermia in locally advanced, hormone-refractory or radiation recurrent prostate cancer: A preliminary report. Int J Radiat Oncol Biol Phys 2003; 57: 654–664
- Tilly W, Gellermann J, Graf R, Hildebrandt B, Weißbach L, Budach V, Felix R, Wust P. Regional hyperthermia in conjunction with definitive radiotherapy against recurrent or locally advanced prostate cancer T3 pN0 M0. Strahlenther Onkol 2005; 181: 35–41
- Maier-Hauff K, Rothe R, Scholz R, Gneveckow U, Wust P, Thiesen B, Feussner A, Von Deimling A, Waldöfner N, Felix R, et al. Intracranial thermotherapy using magnetic nanoparticles combined with external beam radiotherapy: Results of a feasibility study on patients with glioblastoma multiforme. J Neurooncol 2007; 81(1)53–60, Ref. on line 320
- Van Vulpen M, De Leeuw AAC, Van Gellekom MPR, Van der Hoeven J, De Graeff A, Van Morselaar JRA, Van der Tweel I, Hofman P, Lagendijk JJ, Battermann JJ. A prospective quality of life study in patients with locally advanced prostate cancer, treated with radiotherapy with or without regional or interstitial hyperthermia. Int J Hyperthermia 2003; 19: 402–413
- Lein M, Koenig F, Misdraji J, McDougal WS, Jung K, Loening SA, Hasan T, Ortel B. Laser-induced hyperthermia in rat prostate cancer: Role of site of tumor implantation. Urology 2000; 56: 167–172
- Greenstein A, Koontz WW, Jr,. Does local hyperthermia affect metastasis of a human prostate carcinoma grown in athymic nude mice?. Int J Hyperthermia 2002; 18: 285–91
- Bataille N, Vallencien G, Chopin D. Antitumoral local effect and metastatic risk of focused extracorporeal pyrotherapy on Dunning R-3327 tumors. Eur Urol 1996; 29: 72–77
- Sherar MD, Gertner MR, Yue CKK, O'Malley ME, Toi A, Gladman AS, Davidson SR, Trachtenberg J. Interstitial microwave thermal therapy for prostate cancer: Method of treatment and results of a phase I/II trial. J Urol 2001; 166: 1707–1714
- Shariat SF, Raptidis G, Masatoschi M, Bergamaschi F, Slawin KM. Pilot study of radiofrequency interstitial tumor ablation (RITA) for the treatment of radio-recurrent prostate cancer. Prostate 2005; 65(3)26–267
- Gelet A, Chapelon JY, Poissonnier L, Bouvier R, Rouviere O, Curiel L, Janier M, Vallencien G. Local recurrence of prostate cancer after external beam radiotherapy: Early experience of salvage therapy using high-intensity focused ultrasonography. Urology 2004; 63: 625–629
- Master VA, Shinohara K, Carroll PR. Ferromagnetic thermal ablation of locally recurrent prostate cancer: Prostate specific antigen results and immediate/intermediate morbidities. J Urol 2004; 172: 2197–2202