Abstract
Genetic response of human umbilical vein cells (HUVECs) to heat shock was studied using gene expression analysis. HUVECs were subjected to heat treatment at 44°C and 55°C for 1 h, respectively. Four hours after the treatment, gene expression of the treated cells and control cells (37°C) were profiled using cDNA microarray. Data were analyzed using bioinformatics tools, and the results were verified by real-time quantitative PCR experiment. It has been shown that a large number of genes were regulated indicating global response to heat treatment at the genetic level.
Introduction
Recent clinical trials indicated that hyperthermia was effective in the treatment of human malignant tumor, especially in conjugation with radiotherapy or chemotherapy Citation[1]. Thermal effects on tumor tissue microcirculation have been studied, and injury of endothelial cells, which form the inner lining of the blood vessels, is one of the major causes to microcirculation disruption during hyperthermia Citation[2].
Previous studies have paid a great deal of attention to the tumor cell response under hyperthermia Citation[3–7]. Only few were on the endothelial cells, although they are essential to tumor development and progression and have been extensively studied in the area of anti-angiogenesis for drug therapy Citation[8]. Our previous work showed that the loosened junctions between endothelial cells during hyperthermia could facilitate the nano-particle drug delivery into tumor tissue Citation[9], implying that their physiological response may play an important role in tumor therapy.
For mammalian cells, the heat shock effects have been found to be global. They involve almost all known structural and functional systems in cells through activation or inhibition of certain gene expressions Citation[10], Citation[11]: protein activities such as inhibition of translation Citation[12], protein denaturation Citation[13], protein degradation, changes of a variety of kinases and phosphatases Citation[14–16] and cytoskeleton Citation[17], cellular ion homeostasis Citation[18], Citation[19], etc. We also found that the actin cytoskeleton and Ca2+ changed in cytoplasm and nucleolus in endothelial cells during hyperthermi Citation[20], Citation[21]. The global effects of hyperthermia on vascular endothelial cells need to be investigated further.
As the development of advanced technologies, complementary deoxyribonucleic acid (cDNA) microarray enables the expression analysis of tens of thousands genes simultaneously Citation[22] and has been widely used in many areas of cell biology. Using this method, we can observe the global response of endothelial cells during hyperthermia at the genetic level. In this study, the gene expressions of the treated endothelial cells were quantitatively profiled. Gene ontology tools were used to find the significantly changed genes and their functional categories.
Materials and methods
Cell culture
Human umbilical vein endothelial cells (HUVECs; Cascade Biologics, Inc.) were used in this study. Cells were maintained in Medium 200 with microvascular growth supplement (Cascade Biologics, Inc.) at 37°C, 5% CO2, and 95% relative humidity. Cell viability was routinely assessed using the trypan blue exclusion method.
Heat treatment
Ten microliters of 2 × 105 cell/ml endothelial cells were seeded in 75-mm2 screw-topped polystyrene flasks (Coning Inc., USA). After 2 days of incubation, cells reached logarithmic growth stage. The culture medium was replaced 30 min before the heat treatment. The treatment was administrated by immersing flasks in a temperature-regulated water bath (DK-S22, Jinghong Co., Shanghai, China). The wall temperature of the flask in the water bath was calibrated and maintained at 44°C or 55°C (within the error limits of ±0.1°C) for 1 h, respectively. After the treatment, the cells were kept in the incubator for another 4 h. A total of four groups of cells were treated and two at each temperature.
Assessment of apoptosis and necrosis ratio
The ratio of apoptosis and necrosis of heat-treated endothelial cells was assessed using Hoechst/PI (Vybrant® Apoptosis Assay Kit, Catalog Number: V13244, Invitrogen Inc.) labeling technique. Briefly, cells were harvested and washed in cold phosphate-buffered saline (PBS). The cell density was adjusted to 1 × 106 cells/ml in PBS. One microliter of the Hoechst 33 342 solution and 1 µl of the PI solution were added to each 1 ml of cell suspension. Subsequently, the cells were incubated on ice for 20–30 min. After the incubation, the stained cells were analyzed by fluorescence microscopy using UV/488 nm dual excitation and the fluorescence emission measured at ∼460 nm and >575 nm. Live cells show fluorescence at a low level, apoptotic cells show blue fluorescence at a higher level, and necrotic cells show both blue and red fluorescence. The ratios of apoptosis/necrosis were measured at 4, 12, 24, 36, 48, 60 and 72 h after the heat treatment.
Isolation of RNA
After the heat treatment followed by 4 h incubation, cells were harvested in ice-cold phosphate-buffered saline (PBS) with trypsin (0.025%). RNA isolation was performed by following the protocol of the kit (Cat. No. 15596-026, Invitrogen Co. Ltd). RNA yield was determined spectrophotometrically, and the quantity and quality of the RNA were estimated from the absorption at 260 nm and 280 nm. Also, gel electrophoresis on 0.9% agarose gel containing 1:10 000 ethidium bromide was performed to check the RNA. Isolated RNA was put in de-ionized water and stored at −70°C. A similar procedure was followed to extract RNA from all the treated and the control cells (untreated).
cDNA production
Two milligrams of total RNA were heated to 70°C for 5 min, then immediately frozen in liquid nitrogen and lyophilized. The lyophilized RNA was combined with a master mix containing 2 µg oligo dT, 6 µl of 5X first-strand buffer, 3 µl of 5 mM DTT (GIBCO), 3 µl of 5 nM dNTPs mixture, 1 µl RNase inhibitor, 1.5 µl SuperScript RT (GIBCO), and then incubated at 37°C. The reaction was stopped by the addition of 4 µl EDTA (0.5 M, Sigma).
Microarray preparation, hybridization and imaging
Microarray Printing Operon Technologies Genome Oligo Set Version 3.0 contains 34 580 70mer probes representing 24 650 genes and 37 123 gene transcripts. These sense oligos were printed from 93 384 round-bottom-well oligo plates resuspended at a concentration of 25 µM in 8 µl total volume (DMSO) at a spot diameter of 120 µm and a spot center to center distance of 155 µm on Type VII slides (Amersham). The slides were UV crosslinked at the energy setting of 4500 µJ × 100 and baked at 90°C for 20 min.
The control cells (untreated) were labeled with Cy3 and the heat-treated cells (at 44°C or 55°C for 1 h) were labeled with Cy5. Stratagene's FairPlay aminoallyl kit and protocol were used. Slide hybridization and scanning using a manual hybridization chamber and a lifter slip (Erie Scientific, #25X60I-2-4789), 80 µl of the reaction consisted of 50% formamide (Fisher), 1 × hybridization buffer (Amersham, #RPK0325), and 400 picomoles of each probe (Cy3 and Cy5 labeled). The hybridization reaction mix was heated to 90°C for 1–2 min, and then was placed on ice. The hybridization reaction was added to the slide and the chamber was assembled and wrapped in foil. The chamber was placed inside a hybridization oven at 37°C for 16 h. After hybridization, the slides were subjected to a series of washes as follows: 1 × SSC, 0.1% SDS 55°C for 5 min, 0.1 × SSC, 0.1% SDS 25°C for 5 min, 0.5 × SSC 55°C for 5 min and power air-dried. The slides were scanned using a Scan Array Express HT scanner (Perkin Elmer). Data analysis-Spot finding and quantification was performed using Digital Genome (MolecularWare Inc.). Cy3 and Cy5 median intensity value minus local background for each spot was used for further analysis using GeneSpring (Angilent). The ratios of Cy5/Cy3 were used for further analysis, and per chip normalization of the 50th percentile was used. We replicated two experiments for each treatment and control. So there are two arrays comparing 44°C treatment to control, and two arrays comparing 55°C treatment to control. As a quality control, those spots with hybridization intensities (spot median intensity minus local background) of less than 100 were deemed as background hybridization signal and removed from further analysis. For example, to identify genes that are over-expressed in 44°C heat-treated cells, the hybridization intensities should be greater than 100 in both replicated arrays. To identify genes that are over-expressed in control cells vs. heat-treated cells at 44°C the signal intensities from the control hybridization should be greater than 100 in both replicated arrays. We used ratios as measurements and analyzed two replicate arrays separately (instead of averaging the hybridization signals) and only took those showed consistently (i.e. in both arrays) up- or down-regulated genes as truly differentially expressed genes. Two folds were used as the cutoff threshold.
Confirmatory real-time quantitative PCR
Each RNA sample used for microarray analysis was also subjected to real-time quantitative PCR (qPCR) experiment to validate the results. Five genes were selected including two up-regulated genes TP53BP and DNAJB1, two down-regulated genes BUB1A and CCND2, and glyceradehyde-3-phosphate dehydrogenase (GADPH), which is one of the most commonly used housekeeping genes and used as the control in comparison of gene expressions. DNAase-treated total RNA was extracted and reverse-transcripted as described above. The primers were as follows: TP53BP, 5′-CATTAGAGGACATTTAGCGTG-3′ (forward) and 5′-GGCAGGAGGACTGGTTTAG-3′ (reverse), designed to yield a 225-bp amplicon; DNAJB1, 5′-CCCCAAAACACCCGAGAA-3′ (forward) and 5′-ATGGTCCACAACTGGTAGAAAG-3′ (reverse), designed to yield a 193-bp amplicon; BUB1A, 5′-ATGTGGAAGAGGGCTTAGAG-3′ (forward) and 5′-CATTTTGGAAGGCATTTGTT-3′ (reverse), designed to yield a 176-bp amplicon; CCND2, 5′-CAGGAGTGGGAACTGGTGG-3′ (forward) and 5′-GCTCAAGCCTCATCTTACCG-3′ (reverse), designed to yield a 181-bp amplicon; GADPH, 5′-TGATGACATCAAGAAGGTGGTGAAG-3′ (forward) and 5′-TCCTTGGAGGCCATGTGGGCCAT-3′ (reverse), designed to yield a 240-bp amplicon. The PCR amplification mixture in a final volume of 25 µl consisted of 1 X Taq DNA polymerase buffer, 0.25 mM deoxyribonucleoside triphosphate (dNTP), 0.2 µM each primer, 2.5 U Hot start Ex Taq (TaKaRa, Code No.: DRR006A) iQTM SYBR Green Supermix (BIO-RAD) and 1 µl cDNA sample. All the amplicons were confirmed by sequencing. The real-time PCR was performed using Rotor-Gene RG-3000A (Corbett Research) equipped with Rotor-Gene 6.0 for Windows. A pre-amplification denaturation for 4 min at 94°C was performed and amplification was carried out for 40 cycles. Each cycle was 94°C for 30 s, 54°C for 30 s, and 72°C for 30 s. The final extension step was performed for 10 min at 72°C. Fold differences were calculated via a mathematical model described by Pfaffl Citation[23] using the formula: 2−(ΔΔCt), where ΔΔ Ct is ΔCt(heat treatment) − ΔCt(control), ΔCt is Ct(test gene) − Ct(control gene), and Ct is the cycle at which the threshold is crossed.
Gene ontology analyses
Bioinformatics tool EASEonline Citation[24], which provides integrated solutions for the annotation and analysis of genome-scale datasets derived from high-throughput technologies such as microarray and proteomic platforms, was used to analyze the relatively enriched gene ontology categories of experimental results. Before the analysis, the genes with no correlations to any functional categories or not detected in four groups of the treated cell were deleted. The results were saved as a.txt file, and submitted through the Internet. Fisher's exact test was performed to examine the relative enrichment of certain category (p < 0.1).
Results
Microarray results
The fluorescence data from the microarray measurements were transferred into ratios (heat treated/control). All the genes extracted from the cells heated at either 44°C or 55°C were divided into three groups: up-regulated, down-regulated, unchanged. If one gene expression increased more than 2 folds in both experiments at the same temperature, then the gene was considered to be up-regulated at that temperature. On the other hand, if one gene expression decreased to lower than 0.5 folds of the control, then the gene was considered to be down-regulated. The change between 0.5 folds to 2 folds was considered to be unchanged. lists the number of genes changed in three groups (up-regulated, down-regulated, unchanged) after the heat treatment at the two temperatures (44°C and 55°C), respectively. The heat map of all up-regulated, down-regulated and unchanged is also drawn as shown in Appendix I. The heat map was created by Cluster 3.0 with hierarchical cluster. Similarity metric is Euclidean distance and Clustering method is average linkage. The four columns in the map stand for the two micro-array experiments at 44°C and 55°C (from left to right), respectively.
Table I. Number of genes changed after heat treatment at the two temperatures (44°C and 55°C), respectively.
Orthogonal analysis of regulated genes
To show the gene expression difference in cells treated at the two temperatures, an orthogonal analysis was performed. In , all the genes were divided into nine groups according to the change in their expression after the heat treatment at 44°C or 55°C, respectively. The criterions are given in the above.
Table II. Orthogonal analyses of regulated genes at 44°C and 55°C. The number inside circle stands for the group number. The number in the table stands for the number of genes.
Gene ontology (GO) analysis
The relatively significant genes in every category are calculated by online software EASE (NIH) as described in the Materials and methods. The relatively enriched GO categories are listed in . The details of the genes in each group are given in Appendix II.
Table III. Relatively enriched functional categories of each group in .
Quantitative real-time PCR results
In order to assess the reliability of the cDNA microarray, several genes were sequenced and the induction or repression was validated by real-time PCR. In general, the correlation between the micro-array experiments and real-time PCR analysis was very high. The results of two representative genes DNAJB1 (Genebank: BC019827) and CCND2 (Genebank: M88082) are shown in .
Discussion
In this study, the gene expression profile changes in endothelial cells after heat treatment have provided a global view of the cellular response to heat. In contrast to the previous studies Citation[3–5], we used the largest gene array scale, about 35 000 probes on one single gene chip including 24 650 genes and 37 123 gene transcripts. The gene expression patterns of the endothelial cells under a relatively high temperature (55°C) were profiled for the first time. Thousands of genes were found changed after the treatment. There were many more up-regulated genes than the down-regulated genes. The gene ontology hierarchy method was used to group the expressed genes into different functional categories. One can find that the changed genes were related to almost every aspect of cellular physiological activities.
In , groups A and B show the numbers of genes either up-regulated or down-regulated at both 44°C and 55°C. One can call these genes the general heat shock response genes, because their expressions are not dependent on the treatment temperature. Many reported heat-shock-related genes were found in these two groups. Also, there were several functional categories that have never been reported before.
The up-regulated genes include two general gene categories of cell communication and signal transduction. There are G-protein-related genes, including MRGPRX1, GNB2, HCP5, LGR6, GPR20, C14 up-regulated through heat shock. The G-protein signaling pathway is stimulated on the cell surface and transfers the signal to the cell nucleolus. A previous study has shown that cell integrin and cytoskeleton protein were decreased after hyperthermia at 43°C Citation[17]. But, in our results, two integrin-related genes ITGA7 (Integrin 7) and ITGA4 (Integrin, alpha 4) were all up-regulated. The decrease in the protein profile may induce the gene expression of these proteins. In the receptor signaling protein activity category, AKAP6, SHANK2, TIAM2, MAPK6, TYRO3, MAP3K7IP1 all participate in the important signaling transduction member such as PKA, MAPK.
The down-regulated general response genes mainly include the genes related to ion binding, changes in the cytoplasm (cytoplasm, oxidoreductase activity, mitochondrion, lipid metabolism) and response to abiotic stimulus. Four calcium ion binding genes—S100A11P, CAPNS1, ANXA5, EPS15—are all included in the metal ion binding category. Our previous study showed that calcium ion concentration increased in both the nucleolus and cytoplasm during hyperthermia Citation[21]. The decrease of calcium ion binding protein may contribute to this process. Many studies have shown that heat stress can cause the increase of reactive oxygen species and accelerate metabolism rate in cells. In this study, the related genes were all down-regulated. The increase of reactive oxygen species and metabolism may negatively down-regulate the expression of the related genes. Abiotic stimulus stands for the non-living stimulus, IL8 is the gene response to biochemical stimulus, and the other two genes are not related to heat stress. May be the up-regulation detection of external stimulus can depress the other stress-related genes.
The genes in groups B and D changed reversely at the temperature of 44°C and 55°C. Results showed that the number of genes in these two groups was very small compared with the number in the general response groups mentioned above. Also there are no relatively significant genes in these groups.
Groups C, F and G, H show the numbers of genes changed differently at 44°C or 55°C. Many studies had showed that heat shock can cause cell cycle arrest Citation[25–27]. Our previous study also showed that cell cycle was a very important factor in determining the sensitivity of cells to heat shock Citation[20]. In the present results, most genes in group C were cell-cycle-related genes. GML participates in DNA damage response, especially signal transduction by P53 class mediator, resulting in cell cycle arrest. IGF 2 participates in many biological pathways including the cell proliferation control. MNT negatively regulates cell proliferation by binding to DNA binding proteins at its N-terminal Sin3-interaction domain. None of the cell cycle related genes was found in the cells treated at 55°C. Cells went to necrosis directly without going through any gene changes related to cell cycle.
In group G, which includes the genes that were up-regulated specifically at 55°C, these genes were mainly related to protein synthesis activities. This may suggest that, in necrotic endothelial cells, genes related to protein synthesis were up-regulated 6–8 h before the cell death. Very few studies have been done on the gene expression pattern of cells undergoing necrosis. A previous study has proven that protein denaturation occurred during and after heat treatment Citation[13]. Protein denaturation occurs in mammalian cells at temperatures exceeding 40–42°C Citation[28], and more protein denaturation occurred at the temperature of 55°C. Our hypothesis is that in the beginning of the heating at 55°C, protein aggregates formed dramatically in the cytoplasm. The sudden loss of so much protein inside the cell stimulates a dramatic up-regulation of protein synthesis related genes to rescue the cells from death.
In comparison to the previously reported heat induced gene expression changes, studies, there are about 10–15% of the up-regulated or down-regulated genes in common, such as: genes HSP32 (Z82244) Citation[4], Citation[6], HSP70 (U65785) Citation[4] and related chaperonin CCTD (AF026291) Citation[4]; cell growth-, proliferation- and differentiation-related genes COIL (U06632) Citation[4], PLAGL1 (U81992) Citation[4], DP1 (L23959) Citation[3] and WEE1 (X62048); membrane transport function genes NEDD4L (AB007899) Citation[4], KPNB3 (Y08890) Citation[4] and C3AR1 (X98248) Citation[6]; signal transduction genes PRKACB (M34181) Citation[6], YES1 (M15990) Citation[6], PTPN7 (M64322) Citation[6] and CSK (X59932) Citation[5]. Also there are genes related to other functions such apoptosis, immune function, RNA stability and degradation, transcription, etc. Most of the overlapping genes belong to groups A, E and they are general heat responsive genes.
For the biological implication, some phenomena reported in previous studies can be partially explained from the genetic response point of view. Our previous study Citation[9] showed that the hyperthermia treatment could facilitate the drug extravasation in tumor tissue, and the morphology change of endothelial cells played a major role in the process. In this study, many cytoskeleton- and integrin-related genes were found to be up-regulated and down-regulated after heat treatment, indicating the correlated genetic responses. Nikfarjam Citation[2] found that the major reason of heat-induced tumor damage was the injury of endothelial cells. The injury of endothelial cells is mainly caused by heat-induced apoptosis or necrosis. Some apoptosis-related genes were found to be changed; i.e. specific gene Jun (BC009874) reported in our (4 folds in 44°C and 3 folds in 55°C) and other studies Citation[3], Citation[7]. There were many studies about the relationship between c-Jun expression and apoptosis Citation[29] and it was showed that ceramide was involved with c-Jun expression of heat-shock-induced apoptosis Citation[30].
In summary, the gene expression change after heat treatment has been profiled at a huge scale in this study. A large number of genes has been found either up-regulated or down-regulated, indicating that the cellular response to hyperthermia is global and pleotric at the genetic level. Results of the preliminary analyses presented here warrant further investigation on functional protein response to hyperthermia in the near future.
Acknowledgments
This work was supported by National Natural Science Foundation of China (NSFC50436030), Science and Technology Commission of Shanghai Municipality (05DZ22321), Ministry of Science and Technology of China (2006CB0D0100), and Special Funding from Shanghai Center for Systems Biomedicine. We also thank Mr Zhenmin Zhu for his help with data analysis and Ms Jian Wang for her help with real-time quantitative PCR experiment.
References
- Hildebrandt B, Wust P, Ahlers O, Dieing A, Sreenivasa G, Kerner T, Felix R, Riess H. The cellular and molecular basis of hyperthermia. Crit Rev Oncol Hematol 2002; 43: 33–56
- Nikfarjam M, Muralidharan V, Malcontenti-Wilson C, Christophi C. Progressive microvascular injury in liver and colorectal liver metastases following laser induced focal hyperthermia therapy. Lasers Surg Med 2005; 37: 64–73
- Narita N, Noda I, Ohtsubo T, Fujieda S, Tokurik M, Sito T, Saito H. Analysis of heat-shock related gene expression in head-and-neck cancer using cDNA arrays. Int J Radiat Oncol Biol Phys 2002; 53: 190–196
- Sonna LA, Gaffin SL, Pratt RE, Cullivan ML, Angel KC, Lilly CM. Effect of acute heat shock on gene expression by human peripheral blood mononuclear cells. J Appl Physiol 2002; 92: 2208–2220
- Dinh HK, Zhao B, Schuschereba ST, Merrill G, Bowman PD. Gene expression profiling of the response to thermal injury in human cells. Physiol Genomics 2001; 7: 3–13
- Sonna LA, Wenger CB, Flinn S, Sheldon HK, Sawka MN, Lilly CM. Exertional heat injury and gene expression changes: A DNA microarray analysis study. J Appl Physiol 2004; 96: 1943–1953
- Hirano H, Tabuchi Y, Kondo T, Zhao QL, Ogawa R, Cui ZG, Feril LB, Kanayama S. Analysis of gene expression in apoptosis of human lymphoma U937 cells induced by heat shock and the effects of α-phenyl N-tert-butylnitrone(PBN) and its derivatives. Apoptosis 2005; 10: 331–340
- Folkman J. Angiogenesis. Annu Rev Med 2006; 57: 1–18
- Liu P, Zhang A, Xu Y, Xu LX. Study of non-uniform nanoparticle liposome extravasation. Int J Hyper 2005; 21: 259–270
- Lindquist S. The heat-shock response. Annu Rev Biochem 1986; 55: 1151–1191
- Morimoto RI. Regulation of the heat shock transcriptional response: Cross talk between a family of heat shock factors, molecular chaperones, and negative regulators. Genes Dev 1998; 12: 3788–3796
- Panniers R. Translational control during heat shock. Biochimie 1994; 76: 737–747
- Lepock JR. Protein denaturation during heat shock. Adv Mol Cell Biol 1997; 19: 223–259
- Obata T, Brown GE, Yaffe MB. MAP kinase pathways activated by stress: The p38 MAPK pathway. Crit Care Med 2000; 28: 67–77
- Han SI, Ha KS, Kang KI, Kim HD, Kang HS. Heat shock-induced actin polymerization, SAPK/JNK activation, and heat-shock protein expression are mediated by genistein-sensitive tyrosine kinase in K562 cells. Cell Biol Int 2000; 24: 447–457
- Han SI, Oh SY, Woo SH, Kim KH, Kim JH, Kim HD, Kang HS. Implication of a small GTPase Rac1 in the activation of c-Jun N-terminal kinase and heat shock factor in response to heat shock. J Biol Chem 2001; 276: 1889–1895
- Luchentti F, Mannello F, Cnonico B, Battistelli M, Burattini S, Falcieri E, Papa S. Integrin and cytoskeleton behavior in human neuroblastoma cells during hyperthermia-related apoptosis. Apoptosis 2004; 9: 635–648
- Gaffin SL, Koratich M, Hubbard RW. The effect of hyperthermia on intracellular sodium concentrations of isolated human cells. Ann NY Acad Sci 1997; 81: 3637–3639
- Koratich M, Gaffin SL. Mechanisms of calcium transport in human endothelial cells subjected to hyperthermia. J Therm Biol 1999; 24: 245–249
- Chen B, Zhou M, Xu LX. Study of vascular endothelial cell morphology during hyperthermia. J Therm Biol 2005; 30: 111–117
- Xu LX, Chen B, Zhou M. Change of individual vascular endothelial cell calcium during hyperthermia. J Therm Biol 2006; 31: 302–306
- Schena M, Shalon D, Heller R. Parallel human genome analysis: Microarray-based expression monitoring of 1000 genes. Proc Natl Acad Sci USA 1997; 93: 10614–10619
- Pfaffl MW. A new mathematical model for relative quantification in real-time RT-PCR. Nucleic Acids Res 2001; 29: e45
- Dennis G, Jr, Sherman BT, Hosack DA, Yang J, Gao W, Lane HC, Lempicki RA. DAVID: Database for Annotation, Visualization, and Integrated Discovery. Genome Biol 2005; 4: 3, Available online: http://apps1.niaid.nih.gov/david/
- Kuhl NM, Rensing L. Heat shock effects on cell cycle progression. Cell Mol Life Sci 2000; 57: 450–463
- Maldonado-Codina G, Llamazares S, Glover DM. Heat shock results in cell cycle delay and synchronisation of mitotic domains in cellularised Drosophila melanogaster embryos. J Cell Sci 1993; 105: 711–720
- Kuhl NM, Rensing L. Heat shock effects on cell cycle progression. Cell Mol Life Sci 2000; 57: 450–463
- Lepock JR. Cellular effects of hyperthermia: Relevance to the minium dose for thermal damage. Int J Hyperthermia 2003; 19: 252–266
- Shaulian E, Karin M. AP-1 as a regulator of cell life and death. Nat Cell Biol 2002; 4: 131–136
- Kondo T, Matsuda T, Kitano T, Takahashi A, Tashima M, Ishikura H, Umehara H, Domae M, Uchiyama T, Okazaki T. Role of c-jun expression increased by heat shock and ceramide activated caspase-3 in HL-60 cell apoptosis. J Biol Chem 2000; 275: 7668–7676
Appendix I
Heat map of all up-regulated, down-regulated and unchanged genes
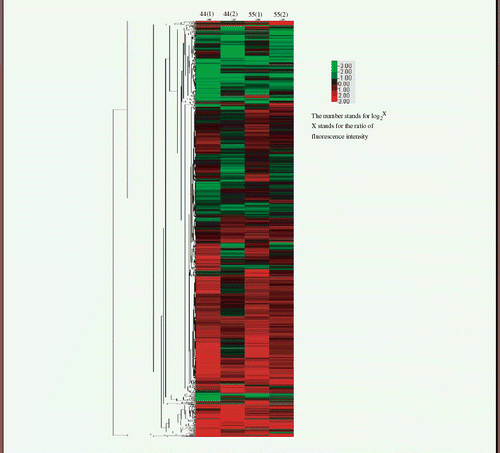
Appendix II
Group 1
Cell communication.
Signal transduction.
Receptor signaling protein activity.
Organismal movement.
Detection of external stimulus.
Group 3
Regulation of cell cycle.
Metabolism.
Biosynthesis.
Protein biosynthesis.
Cell cycle.
Nucleobase, nucleoside, nucleotide and nucleic acid metabolism.
Macromolecule biosynthesis.
Group 5
Metal ion binding.
Cytoplasm.
Oxidoreductase activity.
Oxidoreductase activity.
Mitochondrion.
Calcium ion binding.
Lipid metabolism.
Response to abiotic stimulus.
Group 6
Cytosol.
Large ribosomal subunit.
Cytosolic ribosome (sensu Eukarya).
Ribosome.
Structural constituent of ribosome.
Cytoplasm.
Structural molecule activity.
Cytosolic large ribosomal subunit (sensu Eukarya).
Protein binding.
Chromatin modification.
Protein biosynthesis.
Protein metabolism.
Cell fraction.
DNA packaging.
Chromosome organization and biogenesis (sensu Eukarya).
Establishment and/or maintenance of chromatin architecture.
Nuclear organization and biogenesis.
Ribonucleoprotein complex.
Calcium ion binding.
Regulation of transcription from Pol II promoter.
Chaperone activity.