Abstract
Purpose: To determine the critical thermal dosimetry and relative efficacy for RF ablation combined with external beam radiation (XRT) or liposomal doxorubicin (LD), in an animal tumor model.
Materials and methods: This study was performed in two phases, in 13–18 mm diameter R3230 tumors subcutaneously implanted into Fischer rats. In phase 1, tumors (n = 30) were randomized into six groups. RF energy (titrated to 70°C tip temperature) was applied for either 2.5 or 5 min (n = 15, each group). For each duration, one of three adjuvant therapies was applied (n = 5, each): no therapy (control), LD (1 mg intravenously, 30 min post-RF), or XRT (20 Gy at 1 Gy min−1, within 2 h post-RF), with sacrifice at 48 h for pathologic analysis. In phase 2, thermal mapping was performed in 20 tumors throughout RF application (70°C; 5 min), at 1.5–7 mm distances from the active electrode tip. Temperature profiles throughout the tumor were constructed and were used to interpolate temperatures over time at the critical ablation margin, to derive maximum threshold temperature, AUC (area under the curve) and CEM43 (cumulative equivalent minutes at 43°C). Ablation sizes and all calculated values were compared within and across experimental groups using MANOVA statistics with pair-wise T-test for individual comparisons.
Results: RF/XRT produced the largest coagulation (11.7 ± 1.5 mm at 2.5 min, ≥15 ± 0.7 mm at 5 min), followed by RF/LD, and then RF alone (p < 0.001 for all comparisons). RF/XRT demonstrated temperature threshold decreases from RF alone of 11.7 ± 0.01°C and 12.7 ± 0.38°C at 2.5 and 5 min respectively (with absolute thresholds of 42°C for XRT compared to 52°C for RF alone). RF/LD had decreases of 4.0°C at 2.5 min and 4.4°C at 5 min. Thermal dose requirements (AUC) decreased by 7.79% or 9.28% for RF/LD compared to ≥19.36% or 25.82% for RF/XRT at 2.5 and 5 min (p < 0.001). CEM43 values followed similar patterns (p < 0.001), but with a reduction of 101 and 104 in magnitude for RF/LD and RF/XRT therapies at 5 min, respectively.
Conclusions: For a standardized RF dose, the combination of high dose XRT and RF increased ablation size compared to RF and liposomal doxorubicin or RF alone. Increased ablation size is more closely associated with decreased temperature threshold necessary to induce coagulation, rather than the total thermal dose.
Introduction
Radiofrequency (RF) ablation is a rapidly maturing minimally invasive image-guided tumor therapy that is increasingly utilized for the treatment of focal hepatic, renal, bone and lung tumors Citation[1–6]. However, RF treatment efficacy is hindered by its inability to effectively treat tumors greater than 3–5 cm in diameter Citation[7–9]. This limitation has led to the development of combined therapies that aim to increase coagulation by modulating biophysical properties such as tumor electrical and thermal conductivity as well as blood flow Citation[10–16], or modulation of thermal sensitivity to heat with adjuvant therapies Citation[17].
Along these lines, several animal studies combining RF ablation with liposomal doxorubicin demonstrate increases in coagulation and reduced tumor growth rates over either therapy alone Citation[18],Citation[19]. Studies further suggest a synergy between these combined therapies and a lower thermal dose requirement to achieve coagulation Citation[20]. However, the precise mechanism remains unknown. Additionally, clinical treatments combining RF ablation and other thermal ablation therapies including either hepatic artery infusion chemotherapy with floxuridine and 5-fluorouracil or chemoembolization with doxorubicin have produced improved local control with tolerable side effects Citation[21].
More recently, the effects of combined RF ablation and radiation therapy have been explored. In early clinical studies, Grieco et al. demonstrated the effects of combined external beam radiation (XRT) and RF treatment in primary non-operable non-small cell lung cancer Citation[22]. Similarly, Horkan et al. Citation[23] established RF and radiation therapy dose-dependent tissue sensitivity associated with the combination therapy in a small animal tumor model, though the effects of combined therapy, particularly thermal dosimetry, must be further defined. Horkan et al. further suggested that combination RF/radiation therapy had greater endpoint survival and local cure rates, compared to RF/liposomal doxorubicin or RF alone Citation[23]. Specifically, while D’Ippolito et al. Citation[24] observed a 20% local tumor control rate with RF/liposomal doxorubicin, while, Horkan et al. Citation[23] demonstrated an 82% local cure rate and 120 day survival rate of 50% when combining RF ablation instead with high dose (20Gy) external beam radiation in a similar animal model. However, to date, no head-to-head comparison between these therapies has been made.
The purpose of this study, therefore, was to determine the critical thermal dosimetry for tumor ablation combined with external beam radiation or liposomal doxorubicin, while simultaneously determining the relative efficacy of RF combined with external beam radiation versus RF ablation combined with an optimized liposomal doxorubicin regimen in a controlled animal model.
Materials and methods
Overall experimental design
The study was performed in two phases. In phase 1, animals (n = 30) were randomized into six treatment groups to determine the amount of coagulation necrosis that was induced by different combined treatment regimens. Tumors measuring 13–18 mm received RF energy (e.g. electrode tip temperatures titrated to 70°C) for 2.5 min or 5 min (n = 15, each group). At each RF duration, tissues received one of three potential additional adjuvant therapies post–RF: no adjuvant therapy (control) (n = 5), RF combined with liposomal doxorubicin (LD) (n = 5), and RF combined with radiation therapy (n = 5). Liposomal doxorubicin was administered in a 1 mg dose via IV, 30 min post-RF ablation. XRT was applied at a 20 Gy dose at a rate of 1 Gy per minute, within two hours post-RF ablation and animals were sacrificed 48 hr after treatment for pathologic studies. In the second phase of the study, a thermal mapping study was also performed in a second set of animals (n = 20) to determine heating profiles over time throughout tumors receiving the defined course of RF ablation, as described below. Together, these experiments enable coagulation diameter, maximum temperature, and two methods of calculation of total thermal dose at the margin of ablation (AUC and CEM43 Citation[25–27]), to serve as endpoints for comparison.
Animal model
The Institutional Animal Care and Use Committee approved this protocol. For all experiments and procedures, anesthesia was induced using intraperitoneal (IP) injection of a mixture of ketamine (50 mg/kg; Ketaject; Phoenix Pharmaceutical, Inc., St Joseph, MO) and xylazine (5 mg/kg; Bayer, Shawnee Mission, KS). When necessary, booster anesthesia injections at one-tenth the dose were administered every 30–60 min IP. The experiments were performed with a well established tumor model Citation[17],Citation[28], the R3230 mammary adenocarcinoma in Fischer female rats, the strain in which the tumor was originally derived (mean weight, 150 ± 20g; age range 7–9 weeks) (Taconic Farms, Germantown, NY). All steps in tumor implantation in the animal model have been previously described in several studies using this model Citation[18–19].
Radiofrequency application
A 500 kHz RF generator (CC-1, Radionics®, Burlington, MA) was used to apply conventional, monopolar RF () using a 1 cm tip, 21 gauge electrode placed in the center of the tumor, as has been previously described Citation[17],Citation[18]. Each animal was placed on a standardized grounding pad (Radionics®), to complete the RF circuit, RF energy was applied for 2.5 or 5 min as required with the generator output titrated to maintain a designated tip temperature (70 ± 2°C, mean 90.4 mA ± 25.8, range 48–160 mA).
Figure 1. Rat tumor model. This figure demonstrates the experimental set up of RF ablation within the R3230 breast tumor model where (A) illustrates the grounding pad, (B) the RF generator, and (C) denotes the tumor with RF needle inserted. Inset: This illustrates the RF electrode A and thermocouple B inserted into an acrylic marker for insertion at specific radii to enable thermal mapping.
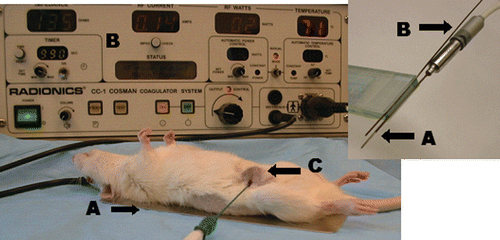
Liposomal doxorubicin ddministration
PEG stabilized, long circulating, liposomal doxorubicin (Doxil®; ALZA Pharmaceuticals, Palo Alto, CA) was administered via direct femoral injection using a dosage of approximately 8 mg/kg (1 mg in 500 µl dilution). The dosage was chosen based upon previous studies demonstrating a marked coagulation increase to 13.5 mm following RF ablation using prior parameters Citation[18]. Injection occurred 20–30 min post-RF application, based upon time course studies performed by Goldberg et al. demonstrating maximal synergistic effect with this protocol Citation[17].
Radiation therapy
External beam radiation was performed at a dosage of 1.0 ± 0.1 Gy for a duration of 20 min, giving a total 20 Gy dose over time, based upon prior work by Horkan et al. Citation[23] using the same tumor model. Animals were positioned on their sides on a lead sheet, with a slit to allow radiation delivery. Subsequently, the sheet was inverted and a second lead sheet was added for lung protection. An RT 250 irradiator (Philips, Eindhoven, Netherlands) was operated at 250 kV and 12 Amps, using a filtration of 0.4 mm tin and 0.25 mm copper. The distance from the radiation source to the skin was 32 mm.
Thermal mapping studies
Thermal mapping was performed in 20 separate animals with 13–18 mm tumors to determine the heating history throughout the entire tumor during ablation, as a means of determining the critical ablation parameters of minimum threshold temperature and thermal dose. Animals were divided into five groups (n = 4) where RF ablation was performed at 70°C tip temperature for duration of 5 min. Thermocouples measuring temperature (°C) were placed at five different radii from the active electrode tip: 1.5, 2.5, 3.5, 5.0, and 7.0 mm ( inset). Temperatures were recorded in intervals of 30 sec throughout the duration of ablation. Temperatures at each time interval and distance were averaged and maximum temperatures determined. For every 30 sec, negative exponential curves were calculated. Temperatures at the critical ablation margin, the interface between white coagulation and unablated tumor tissue for all 30 specimens of Phase I, were interpolated from these curves and the total heating history was constructed. Given the radial heating of RF ablation, this is the margin where one sees ablative effects with the lowest thermal dose Citation[20]. This mapping study was used to derive three key endpoints: maximum threshold temperature (the isotherm at which coagulation was seen at the margin of coagulation at the conclusion of the ablation); AUC (area under the curve), which represents total thermal energy input (i.e. an integration of temperature over time) at the margin, and CEM43 (cumulative equivalent minutes at 43°C) using the formula:t = time interval, T = average temperature during time interval t, R = number of minutes needed to compensate for a 1° temperature change either above or below the break point.
Quantification of tumor coagulation necrosis and histologic studies
Induced coagulation necrosis (tumor destruction) was measured 48 h after treatment, based upon results from prior studies Citation[29],Citation[30]. Animals were sacrificed with pentobarbital overdose, and tumors were excised and sectioned. In addition to hemotoxylin-eosin staining, histopathologic studies included staining for mitochondrial enzyme activity by incubating representative tissue sections for 30 min in 2% 2,3,5-triphenyl tetrazolium chloride (TTC) at room temperature. This latter test was used to identify irreversible non-specific cellular injury Citation[31] during the early stages of RF-induced tissue necrosis Citation[28],Citation[32], and has been previously shown to correlate with histopathologic findings of RF-induced tumor coagulation Citation[28],Citation[30]. Gross measurements of tumor destruction were performed on both TTC-stained and unstained sections, and the extent of visible coagulation was measured with calipers (). Coagulation diameter (longest measurement perpendicular to the inserted electrode Citation[28] was determined by consensus of two observers who jointly measured and verified the findings. Previous studies have documented close correlation between gross pathologic and histopathologic findings for RF-induced coagulation Citation[33].
Figure 2. Cross section of stained ablated tumor. This figure demonstrates a cross section of RF ablated tumor stained with TTC. Sharp demarcation is noted at the margin (arrow) between the white, ablated tissue and the unablated surrounding tumor tissue stained pink. Central dot in the white coagulation denotes the original position of the RF electrode.
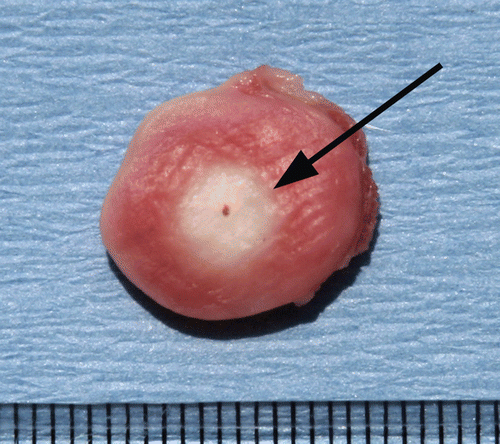
Statistical and data analysis
For each experimental group, coagulation diameters were measured at 48 h and correlated to calculated values. Results were compared using MANOVA statistics with pair-wise T-test for individual comparison. Calculated threshold temperatures corresponding to the AUC (i.e. the total heating history of the tissue (°C·sec)) were also compared among the groups using MANOVA.
Results
Effects of combined therapies on RF coagulation
Significant differences in coagulation diameter were identified among the groups as compared with controls (p < 0.001) (). RF combined with radiation therapy produced the greatest amount of tumor destruction (11.7 ± 1.5 mm at 2.5 min, ≥15 ± 0.7 mm at 5 min, (i.e. ablation consistently extending to the tumor margins)). Comparatively, RF combined with liposomal doxorubicin produced less coagulation (8.6 ± 0.2 at 5 min) than radiation therapy, yet more than the control treatment (6.7 ± 0.2 at 5 min; p < 0.001) (). Differences in duration of treatment also produced differences in coagulation diameter. For example, a difference of at least 3 mm was exhibited between 2.5 min (11.7 ± 1.5 mm) and 5 min (≥15 mm) for combined treatment of RF and radiation therapy.
Table I. The effects of application duration upon combined adjuvant therapies. This table demonstrates that an increase in RF dose produces increases in coagulation, AUC, and CEM43. In comparison to RF alone and RF combined with Doxil, high dose XRT produces increases in coagulation while decreasing AUC and CEM43 (percentage decreases in AUC are based upon the baseline of RF alone for a given duration). Within each experimental group, duration of application had no effect on temperature threshold for ablation.
Thermal dosimetry
Classic sigmoidal Boltzmann curves were obtained for each temperature time curve at distances of 1.5–7 mm (r2 = 0.94–0.99) () Citation[20]. Calculations based upon these temperature measurements demonstrated significant decreases in the ablation temperature threshold (p < 0.001) for combined therapies. The temperature required for ablation decreased by 11.7 ± 0.01°C (from 52°C to 40.3°C) and 12.7 ± 0.38°C (from 52.4°C to 39.7°C) for RF application combined with radiation therapy for 2.5 and 5 min, respectively. By comparison, RF/liposomal doxorubicin, yielded only a 4°C decrease (i.e. a 47.2°C threshold) at 2.5 min and 4.4°C decrease (i.e. a 48°C threshold) at 5 min.
Figure 3. Thermal mapping of temperature distributions within a subcutaneous tumor. Mean temperature profiles for multiple measurements at five distances within the tumor (1.5–7 mm from the electrode) are presented with T-bars. The temperatures increase in a sigmoidal Boltzmann distribution (R2 = 0.94–0.99).
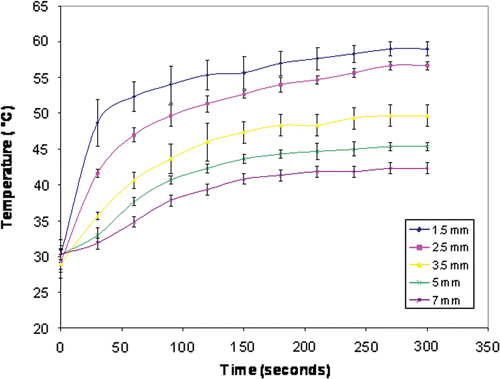
Correlation of thermal dose (AUC and CEM43)to coagulation
A reduced thermal dose requirement for RF/liposomal doxorubicin and even greater reduction for RF/radiation therapy was observed at both durations of RF application compared to RF alone (p < 0.001). However, all therapies performed at durations of 5 minutes yielded greater AUC and CEM43 values than those performed at 2.5 min (p < 0.001). The percentage decrease in AUC values for each combined therapy were similar, regardless of duration, in comparison the RF control. AUC values for RF applications decreased by 7.79% and 9.28% when followed by liposomal doxorubicin injection, and by at least 19.36% and 25.82% when followed by radiation therapy at 2.5 and 5 min, respectively (). CEM43 values followed similar patterns (p < 0.001), but with a reduction of 101 and 104 in magnitude for liposomal doxorubicin and radiation therapies at 5 min, respectively.
Discussion
Classically, studies combining external beam radiation therapy and/or chemotherapy with low temperature hyperthermia (treatment duration of 1–2 hours at 42–45°C Citation[27],Citation[34]) have suggested synergy and increased efficacy for treatment of tumors in the lung Citation[35],Citation[36], prostate Citation[37], bladder Citation[38],Citation[39], and other sites Citation[40]. In contrast, targeted minimally-invasive tumor therapy with RF ablation uses focal heating of the tumor to extremely high temperatures, though large areas of the tissue beyond the zone of coagulation are still exposed to non-coagulative hyperthermic temperatures (40–50°C) Citation[20]. Therefore, more recently, several preliminary studies have combined RF ablation with adjuvant therapies such as external beam radiation and IV chemotherapy to increase tumor destruction by modulated thermal sensitivity of tumor cells in these peripheral zones exposed to non-lethal temperatures Citation[19],Citation[22],Citation[23],Citation[30]. Given the increased clinical adoption of many of these combinations, continued investigation into both the specific mechanisms of combined therapies and clinical optimization strategies, are required. Along these lines, our study further defines the relationship between radiation therapy and RF ablation, as well as its greater efficacy in comparison to RF combined with doxorubicin.
This six-arm study varying RF dose and combined adjuvant demonstrated the varying efficacy of radiation therapy and liposomal doxorubicin in this tumor model. As has been previously demonstrated in this model, combination therapies result in greater coagulation compared to RF alone. In this study, we further observed significantly greater coagulation for treatment of RF/radiation therapy compared to RF/liposomal doxorubicin, and with both combination therapies over RF alone. These findings, especially the suggestion of complete tumor coagulation observed with RF/radiation therapy at 5 min, are consistent with a prior study by Horkan et al. in which most tumors had complete local tumor regression Citation[23].
Significant differences in the temperature threshold to achieve ablation were also observed between various therapies at both durations, and were ∼40°C for RF/radiation therapy,∼48°C for RF/liposomal doxorubicin, compared to ∼52°C for RF alone. These findings suggest that increased coagulation with RF/radiation therapy occurs due to the ability of adjuvant radiation therapy to induce greater damage to tumor cells in the peripheral zone exposed to previously reversible injury from non-lethal temperatures Citation[34]. While the exact underlying mechanisms require further investigation, the difference in temperature threshold between adjuvant radiation and liposomal doxorubicin likely reflects differences in mechanism of cell injury, or variable effects on an as yet ill-defined mechanism, with radiation combined therapy being a greater potentiator for cell death at lower thermal dose. Indeed, further investigation into possible underlying mechanisms such as the increased free radical and/or heat shock protein formation that leads to greater tumor necrosis, the role of oxidative stress Citation[41], and changes in programmed cell death (apoptosis) Citation[42], is required. Differences in the uniformity of tissue effects, given that interstitial distribution of chemotherapy is likely more heterogeneous in distribution compared to the effects of radiation therapy, is also a likely contributory factor.
AUC, measuring the total heating curve at the margin of coagulation, a conventional measure of total thermal dose, had a similar percentage decrease with either combination radiation (19.36–25.82%) or liposomal doxorubicin (7.79–9.28%) for both RF durations. A similar pattern was observed for CEM43, though this measurement is likely to have even more limited clinical application for ablation given the very wide variability in magnitude (up to 104) in this study, similar to widely variable results (1011) reported by Mertyna et al. in various ex vivo and in vivo tissues Citation[20]. Yet our results also suggest that classic hyperthermia rules may not apply to higher temperatures and shorter durations of RF combined treatment given the fact that thermal dosimetry changed with time, whereas it has been reported as being constant for classic low temperature hyperthermia scenarios. As a result, temperature endpoints should likely be relied upon in the clinic at this time, realizing that wide variations in thermal sensitivity have been reported for different tissues Citation[20].
Some limitations in our study warrant noting. Our results were obtained through experimentation using the R3230 breast tumor model, thus our conclusions are primarily applicable to this tumor type. Thus, it is conceivable that a combination of RF and chemotherapy may be more beneficial than RF with radiation therapy for other clinical and relevant tumor types such as hepatocellular and renal cell carcinoma, which may be heterogeneous (i.e. cystic) or hypervascular Citation[43],Citation[44]. Furthermore, we only included a single chemotherapy agent, liposomal doxorubicin, in this study, and presumably this tumor line may demonstrate different sensitivities to other agents. Indeed, a prior study combining RF ablation with different liposomal chemotherapeutic agents in this same animal model has demonstrated varying tumor sensitivity to various agents Citation[19]. Along similar lines, we use a single radiation dose, and while this has been previously identified as the optimal dose Citation[23], differing and more clinically realistic radiation doses may not be as effective compared to chemotherapy. Additionally, interpolative thermometry in this study was facilitated by the orderly decrease in heat distribution observed in this relatively homogeneous tumor model. Yet, prior studies have suggested that variable tumor composition or tumors with high perfusion demonstrate non-uniform heating surrounding RF electrodes, with documented rapid exponential decreases in tissue temperature Citation[10],Citation[45]). Furthermore, major heat-sink effect from blood vessels will decrease the level of hyperthermic sensitization of tumor to irradiation Citation[46],Citation[47]. Thus, further work and tailoring is likely warranted.
In conclusion, this study demonstrates that the combined therapy of high dose radiation therapy and RF markedly increases ablation size in comparison to traditional RF or RF combined with liposomal doxorubicin. Additionally, the correlation between ablation size and specific threshold temperature at the ablation margin suggests that temperature, rather than thermal dose, may serve as a more applicable marker for successful RF application in a clinical setting. However, this study further supports the strong clinical potential clinical of combined adjuvant therapies, necessitating further research into mechanisms of operation.
Acknowledgements
Supported by grant number NCI 1R01CA112533–01 “Enhanced RF Tumor Ablation with Liposomal Chemotherapy” from the National Institutes of Health, USA.
References
- Goldberg SN, Gazelle GS, Mueller PR. Thermal ablation therapy for focal malignancy: A unified approach to underlying principles, techniques, and diagnostic imaging guidance. AJR Am J Roentgenol 2000; 174: 323–331
- Clasen S, Pereira PL. [Radiofrequency ablation of lung tumors]. Rofo 2006; 178: 852–861
- Dodd GD, III, et al. Minimally invasive treatment of malignant hepatic tumors: At the threshold of a major breakthrough. Radiographics 2000; 20: 9–27
- Dupuy DE, et al. Percutaneous radiofrequency ablation of malignancies in the lung. AJR Am J Roentgenol 2000; 174: 57–59
- Hines-Peralta A, Goldberg SN. Review of radiofrequency ablation for renal cell carcinoma. Clin Cancer Res 2004; 10: 6328S–34S
- Lindner NJ, et al. Percutaneous radiofrequency ablation in osteoid osteoma. J Bone Joint Surg Br 2001; 83: 391–396
- Livraghi T, et al. Hepatocellular carcinoma: Radio-frequency ablation of medium and large lesions. Radiology 2000; 214: 761–768
- Rossi S, et al. Percutaneous treatment of small hepatic tumors by an expandable RF needle electrode. AJR Am J Roentgenol 1998; 170: 1015–1022
- Solbiati L, et al. Hepatic metastases: Percutaneous radio-frequency ablation with cooled-tip electrodes. Radiology 1997; 205: 367–373
- Goldberg SN, et al. Radio-frequency tissue ablation: Effect of pharmacologic modulation of blood flow on coagulation diameter. Radiology 1998; 209: 761–767
- Patterson EJ, et al. Radiofrequency ablation of porcine liver in vivo: Effects of blood flow and treatment time on lesion size. Ann Surg 1998; 227: 559–565
- Leveillee RJ, et al. Enhanced radiofrequency ablation of canine prostate utilizing a liquid conductor: The virtual electrode. J Endourol 1996; 10: 5–11
- Ahmed M, et al. Image-guided percutaneous chemical and radiofrequency tumor ablation in an animal model. J Vasc Interv Radiol 2003; 14: 1045–1052
- Lobo SM, et al. Radiofrequency ablation: Modeling the enhanced temperature response to adjuvant NaCl pretreatment. Radiology 2004; 230: 175–182
- Liu Z, et al. Radiofrequency tumor ablation: Insight into improved efficacy using computer modeling. AJR Am J Roentgenol 2005; 184: 1347–1352
- Shahidi AV, Savard P. A finite element model for radiofrequency ablation of the myocardium. IEEE Trans Biomed Eng 1994; 41: 963–968
- Goldberg SN, et al. Radiofrequency ablation of hepatic tumors: Increased tumor destruction with adjuvant liposomal doxorubicin therapy. AJR Am J Roentgenol 2002; 179: 93–101
- Ahmed M, et al. Combination radiofrequency ablation with intratumoral liposomal doxorubicin: Effect on drug accumulation and coagulation in multiple tissues and tumor types in animals. Radiology 2005; 235: 469–477
- Ahmed M, et al. Combined radiofrequency ablation and adjuvant liposomal chemotherapy: Effect of chemotherapeutic agent, nanoparticle size, and circulation time. J Vasc Interv Radiol 2005; 16: 1365–1371
- Mertyna P, et al. Radiofrequency ablation: Variability in heat sensitivity in tumors and tissues. J Vasc Interv Radiol 2007; 8: 647–654
- Scaife CL, et al. Feasibility of adjuvant hepatic arterial infusion of chemotherapy after radiofrequency ablation with or without resection in patients with hepatic metastases from colorectal cancer. Ann Surg Oncol 2003; 10: 348–354
- Grieco CA, et al. Percutaneous image-guided thermal ablation and radiation therapy: Outcomes of combined treatment for 41 patients with inoperable stage I/II non-small-cell lung cancer. J Vasc Interv Radiol 2006; 17: 1117–1124
- Horkan C, et al. Reduced tumor growth with combined radiofrequency ablation and radiation therapy in a rat breast tumor model. Radiology 2005; 235: 81–88
- D'Ippolito G, et al. Percutaneous tumor ablation: Reduced tumor growth with combined radio-frequency ablation and liposomal doxorubicin in a rat breast tumor model. Radiology 2003; 228: 112–118
- Sapareto SA, Dewey WC. Thermal dose determination in cancer therapy. Int J Radiat Oncol Biol Phys 1984; 10: 787–800
- Sapareto SA, et al. Effects of hyperthermia on survival and progression of Chinese hamster ovary cells. Cancer Res 1978; 38: 393–400
- Sapareto SA, Raaphorst GP, Dewey WC. Cell killing and the sequencing of hyperthermia and radiation. Int J Radiat Oncol Biol Phys 1979; 5: 343–347
- Goldberg SN, et al. Percutaneous tumor ablation: Increased coagulation by combining radio-frequency ablation and ethanol instillation in a rat breast tumor model. Radiology 2000; 217: 827–831
- Goldberg SN. Comparison of techniques for image-guided ablation of focal liver tumors. Radiology 2002; 223: 304–307
- Goldberg SN, et al. Percutaneous tumor ablation: Increased necrosis with combined radio-frequency ablation and intravenous liposomal doxorubicin in a rat breast tumor model. Radiology 2002; 222: 797–804
- Liszczak TM, et al. Limitations of tetrazolium salts in delineating infarcted brain. Acta Neuropathol (Berlin) 1984; 65: 150–157
- Goldberg SN, et al. Percutaneous tumor ablation: Increased necrosis with combined radio-frequency ablation and intratumoral doxorubicin injection in a rat breast tumor model. Radiology 2001; 220: 420–427
- Goldberg SN, et al. Treatment of intrahepatic malignancy with radiofrequency ablation: Radiologic-pathologic correlation. Cancer 2000; 88: 2452–2463
- Dewhirst MW, et al. Basic principles of thermal dosimetry and thermal thresholds for tissue damage from hyperthermia. Int J Hyperthermia 2003; 19: 267–294
- Sakurai H, et al. Cytotoxic enhancement of low dose-rate irradiation in human lung cancer cells by mild hyperthermia. Anticancer Res 1998; 18: 2525–2528
- Schroder C, et al. Technique and results of hyperthermic (41°C) isolated lung perfusion with high-doses of cisplatin for the treatment of surgically relapsing or unresectable lung sarcoma metastasis. Eur J Cardiothorac Surg 2002; 22: 41–46
- Peschke P, et al. Interstitial radiation and hyperthermia in the Dunning R3327 prostate tumour model: Therapeutic efficacy depends on radiation dose-rate, sequence and frequency of heating. Int J Radiat Biol 1996; 70: 609–616
- van der Heijden AG, et al. Effect of hyperthermia on the cytotoxicity of four chemotherapeutic agents currently used for the treatment of transitional cell carcinoma of the bladder:. An in vitro study. J Urol 2005; 173: 1375–1380
- Iizumi T, et al. Immunochemotherapy for murine bladder tumor with a new human recombinant tumor necrosis factor (rTNF-S), VP-16 and hyperthermia. J Urol 1989; 142: 386–389
- Manning MR, et al. Clinical hyperthermia: Results of a phase I trial employing hyperthermia alone or in combination with external beam or interstitial radiotherapy. Cancer 1982; 49: 205–216
- Spitz DR, et al. Metabolic oxidation/reduction reactions and cellular responses to ionizing radiation: A unifying concept in stress response biology. Cancer Metastasis Rev 2004; 23: 311–322
- Ozben T. Oxidative stress and apoptosis: Impact on cancer therapy. J Pharm Sci 2007
- Namasivayam S, et al. Hypervascular hepatic focal lesions: Spectrum of imaging features. Curr Probl Diagn. Radiol 2007; 6: 107–123
- Sheir KZ, et al. Differentiation of renal cell carcinoma subtypes by multislice computerized tomography. J Urol 2005; 174: 451–455;discussion 455
- Goldberg SN, et al. Percutaneous radiofrequency tissue ablation: Does perfusion-mediated tissue cooling limit coagulation necrosis?. J Vasc Interv Radiol 1998; 9: 101–111
- Lu DS, et al. Effect of vessel size on creation of hepatic radiofrequency lesions in pigs: Assessment of the ‘heat sink' effect. AJR Am J Roentgenol 2002; 178: 47–51
- Haemmerich D, et al. Hepatic bipolar radiofrequency ablation creates coagulation zones close to blood vessels: A finite element study. Med Biol Eng Comput 2003; 41: 317–323