Abstract
A polyacrylamide gel (PAG) containing bovine serum albumin (BSA) is introduced as a new tissue-mimicking phantom for the purpose of visualizing three-dimensional coagulation temperature distribution during radiofrequency ablation (RFA). The coagulation temperature of the phantom can be changed at the same range of biological tissue (50–60°C) by adjusting the pH from 4.3 to 4.7. The phantom is transparent except in thermal coagulation regions which are ivory white. The physical properties of the phantom, such as density, electrical conductivity and specific heat capacity, are very favorable, similar to those of soft tissues. We illustrate the usefulness of the phantom in visualizing RFA lesions. This phantom has magnetic resonance properties which change drastically upon thermal coagulation, enabling its use for the characterization of RFA device, quality assurance, treatment planning and treatment verfication. The PAG containing BSA, whose pH was adjusted from 4.3 to 4.7, is an attractive tissue-mimicking phantom suitable for RFA investigations.
Introduction
Radiofrequency ablation (RFA) has now been one of the most promising minimally invasive techniques for the treatment of most soft organs’ pathologies, such as the elimination of cardiac arrhythmias Citation[1], or the destruction of solid tumors in different locations, including liver Citation[2], kidney Citation[3], bone Citation[4], lung Citation[5], breast Citation[6], prostate Citation[7] and thyroid Citation[8]. As a technique, the use of radiofrequency energy in ablation procedures has been well established. The intent of RFA devices is to thermally necrose tissue by raising targeted tissue temperatures to approximately 50°–100°C for a period of 10–15 minutes in tumor ablation Citation[9],Citation[10] and 1–2 minutes in cardiac ablation. For tumor ablation, the electrodes are commonly inserted percutaneously into tissues where cancerous tumors have been identified with the guidance of the imaging modalities, such as ultrasound, CT or MRI. Once the electrodes are positioned, radiofrequency energy is delivered through the electrode into the targeted tissue, and to a dispersive ground pad that is applied to the skin of the patient.
Like other thermal coagulation techniques, however, accurate knowledge of the RFA device characterization is essential to this technique. Use of a tissue-mimicking phantom to visualize the three-dimensional temperature distribution is useful for investigating the characteristic heating shape and range of various RFA devices. Therefore, the phantom can play a great role in the thermal therapy study, such as device development, testing and calibration of heating devices, verification of treatment plans, quality assurance of therapeutic delivery systems in clinical practice and personnel training. Lots of materials have been used for phantoms for thermal studies, including polyacrylamide gel (PAG) Citation[11], gelatine Citation[12], agar Citation[13] and carrageenan Citation[14]. The PAG containing protein, such as BSA or egg white, has been used for thermal study by many investigators Citation[11],Citation[15–17]. The phantom which is mainly made from PAG and BSA has several merits for an ideal thermal gel material: (a) stability at the high temperatures experienced in thermal coagulation therapy (50°–100°C); (b) a solid form to avoid heat transfer by convection; (c) high optical transparency at room temperature and three-dimensional visualization of the heating area as a ivory white turbidity (protein coagulation); (d) accurate visualization of the coagulation lesion without thermal hysteresis; (e) some physical properties, such as specific heat capacity, similar to those of human tissues; (f) sufficient strength and low fragility; (g) chemical and physical long-term stability; (h) ease of production. However, the BSA coagulates at above approximately 70°C, which is higher than biological tissue (50°–60°C) Citation[18–21]. The coagulation temperature of the BSA adjusted to the same range of the biological tissue by changing the pH was previously reported by McDonald et al. Citation[17]. They introduced the method to lower the coagulation temperature of the phantom whose pH was adjusted from 4.3–4.7 by use of citrate buffer. In their research, the coagulation temperature of the phantom was approximately 50°C, 55°C, and 60°C at pH 4.3, 4.5, and 4.7, respectively. And they also pointed out that the phantom could be used for multi-modality of thermal study. To the best of our knowledge, however, the application of this kind of phantom to RFA study has not been reported hitherto in literature. So the aim of the present study is to introduce the preliminary application of this phantom to RFA experiment investigations.
Methods and materials
Tissue-mimicking phantom fabrication
This tissue-mimicking phantom gel is based on a PAG mixed with BSA, a protein used here as a temperature-sensitive indicator, which has been described previously Citation[17]. The recipe and fabrication procedure for phantom in the present study was almost wholly adapted from theirs except for some modifications: (1) The citric acid anhydrate is replaced by citric acid monohydrate (Sigma-Aldrich, C1909); (2) The total concentration of the acrylamide and bis-acrylamide is 10% and cross-linking concentration of the N, N methylene-bis-acrylamide is 5%; (3) BSA solution (30.8% w/v) is replaced by lyophilized powder (Tianchen, Shanghai, China, 223NXB038875); and (4) Intralipid is replaced by glycerol (99% w/v, Tianchen, Shanghai, China). The recipe for phantom (1 L) with a citrate buffer concentration of 0.2 M and pH of 4.3 and was presented in .
Table I. The recipe for phantom with a citrate buffer concentration of 0.2 M and pH of 4.3. (1L).
In the present study, a rectangular parallelopiped mould (250 mL, 7.7 cm × 6.5 cm × 5 cm) was used for phantom fabrication.
Phantom coagulation temperature measurement
The coagulation temperatures of the phantoms were measured using a spectrophotometer (LangGuang 711, Shanghai Precision & Scientific Instrument Co. Ltd, Shanghai, China). The coagulation temperature was calculated and defined as the relative absorbance through a gel phantom, when the absorbance through a cuvette containing the uncoagulated fresh phantom was taken as 0 and the absorbance at coagulated phantom was measured. The threshold for coagulation was the temperature where absorbance was 50% or greater than that of the absorbance measured for a coagulated sample.
Multiple phantom samples (2 mL) at each pH were prepared in cuvettes () and heated slowly in a water bath from 45° to 65°C for BSA coagulation (). The procedures for measurement were taken as follows: (1) The cuvette containing the fresh phantom was warmed to room temperature (24°–25°C), wiped with a lab wipe and placed into the sample holder. (2) The cover was closed and the light control knob used to set the meter needle to ‘0’ on the absorbance scale. (3) The cuvette was removed and heated in the water bath (approximately 10 min). (4) Then the cuvette was removed from the bath, cooled to room temperature, wiped and placed into the sample holder and the cover closed. (5) The absorbance was read and recorded. Each cuvette was removed from the bath at an interval of 1°C. In the present study, eight independent experiments (8 samples) were conducted at each pH (4.2–4.8), giving a total of 56 coagulation temperature measurements.
Phantom physical properties measurement
To determine electrical conductivity of the phantom, we need to measure three values, including electrical resistance (R), distance (d) and transverse cross-section area of the electric current (S). In the present study, a digital LCR meter (E4980A, Aglient, USA) was used to measure the electrical resistance of the phantom. The refrigerated phantom was warmed to 37°C and the procedure was performed at the frequency of 470 Hz. The electrical conductivity of the samples, s (Ω−1 m−1), was calculated according to the following equation:where d is the thickness of the test sample in the direction of electric current (m), R is the electrical resistance of the sample (O) and S is the cross-section area of the electric current through the sample (m2). In the present study the cross-sectional area of the electric current is determined by the measurement of the area of phantom where the electrodes of LCR meter are applied.
A total of twelve electrical conductivity measurements were performed on twelve phantom samples, four each from three different pH samples (4.3, 4.5, and 4.7). A total of nine specific heat capacity measurements were performed on nine samples, three each from three different pH samples (4.3, 4.5, and 4.7). And a total of eighteen density measurements were performed on eighteen samples, six each from three different pH samples (4.3, 4.5, and 4.7).
The density of the phantom was obtained as the mass divided by the volume of a tested sample. The specific heat capacity of the phantom was obtained by conventional calorimetry by using a Dewar vessel with a heat capacity of 149 J°C−1.
Radiofrequency ablation of the phantoms
RITA System (RITA Model 1500 RF Generator with Starburst™ XL electrode, RITA Medical System, USA) was used during the procedure. The method of the phantom heating experiment was illustrated in . The refrigerated phantoms were warmed to room temperature (24°–25°C). The procedure was performed by inserting the electrode trocar into the phantom with 2 cm in depth and the nine curved prongs were deployed by pushing the piston down the shaft of the electrode with the deployment diameter of 2 cm (the marks distal to the piston informed the operator about the deployment diameter) (). The generator was operated in A mode (automatic temperature control: average of all heating to target temperature). Power was set to 50 W, with the target heat at 80°C and the procedure was continued 7 minutes. The development of the coagulation lesions in phantoms were recorded by digital camera during the procedure. In addition, the coagulation lesions were investigated using gray-scale ultrasound (TECHNOSMPX DU8 Diagnostic Ultrasound System with a 10 MHz linear array probe, ESAOTE, Italy) and MRI (1.5T Sigma Magnetic Resonance Imaging Scanner, GE Medical Systems, USA) following the ablation.
Results
Phantom coagulation temperature measurement
The coagulation temperature of the tissue-mimicking phantom for the different pH samples is given in . It illustrates that the coagulation temperature of the phantom increased with pH at the range of 4.2 to 4.8. The coagulation temperature was 50.8 ± 0.7°C, 55.3 ± 0.5°C, and 59.0 ± 0.5°C at pH 4.3, 4.5 and 4.7, respectively.
Phantom physical properties
The phantom was an optical transparent solid form gel with light amber. The physical properties of the different pH phantoms (4.3, 4.5 and 4.7) with 2% BSA concentrations are presented in . The densities of the different pH phantoms (4.3, 4.5 and 4.7) were 1.069 ± 0.003 kg m−3, 1.069 ± 0.003 kg m−3, and 1.068 ± 0.002 kg m−3, respectively. The electrical conductivities of the different pH phantoms (4.3, 4.5 and 4.7) were 0.117 ± 0.003 S m−1, 0.118 ± 0.005 S m−1, and 0.118 ± 0.003 S m−1, respectively (37°C, 470 kHz). And the specific heat capacities of the different pH phantoms (4.3, 4.5 and 4.7) were 3,676 ± 195 J kg−1 °C−1, 3,797 ± 153 J kg−1 °C−1, 3,810 ± 142 J kg−1 °C−1, respectively. These parameters were almost similar to those of human liver Citation[22],Citation[23].
Table II. The physical properties of the phantoms and human liver (mean ± SD).
Radiofrequency ablation of the phantoms
An ivory white coagulation lesion developed around the tip of the central prong in less than 20 seconds during heating (). The coagulation lesion enlarged gradually and more coagulation lesions developed and enlarged around the tip of the other prongs advancing with the time (). After 7 minutes of heating, the coagulation lesions merged into an ellipsoid region (). All of the coagulation lesions in the phantoms showed well-defined boundaries from the uncoagulation regions and demonstrated different temperature distribution in different pH phantoms. The size of the coagulation lesions decreased from pH 4.3 to 4.7 in 7 minutes of ablation and represented the coagulation temperature approximately 50°, 55° and 60°C for pH 4.3, 4.5 and 4.7, respectively (). The coagulation lesion in the phantoms cannot be demonstrated on gray-scale ultrasound during and after the heating. The air-echo can be demonstrated on sonogram in the coagulation zone when the temperatures approached and exceeded 100°C during heating (). However, MRI showed the coagulation lesions with well-defined hypo-intensity signal on T2-weighted images (). In addition, the coagulation lesions on T2-weighted images demonstrated the similar size and shape corresponding to the gross view ().
Figure 4. The development of coagulation lesions in tissue-mimicking phantoms (pH = 4.3) during RFA. The pictures show coagulation lesions in phantoms are ivory white regions with well-defined boundary during RFA: (A) The coagulation lesion develops around the tip of the central prong in less than 20 seconds during heating (arrow); (B) More coagulation lesions develop around the prongs after 40 seconds of heating; (C) The coagulation lesions enlarge and merge after 2 minutes of heating; and (D) The coagulation lesions merge into an ellipsoid after 7 minutes of heating.
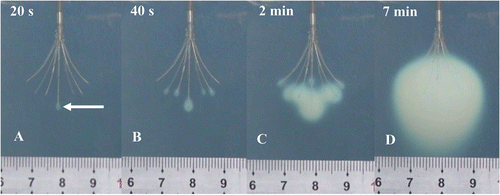
Figure 5. The coagulation lesions in tissue-mimicking phantoms after 7 minutes of RFA. The ivory white ellipsoid coagulation lesions demonstrate different temperature distribution in different pH phantoms: (A) represents approximately 60°C coagulation lesion (pH 4.7); (B) represents approximately 55°C coagulation lesion (pH 4.5); and (C) represents approximately 50°C coagulation lesion (pH 4.3).
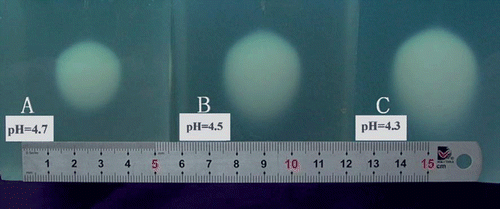
Discussion
RFA, a technique of thermal coagulation therapy, is less invasive and can sometimes offer an alternative when surgery is not feasible. The aim of tumor RFA therapy is to destroy an entire tumor using heat to kill the malignant cells in a minimally invasive fashion without damaging adjacent vital structures. This often includes the treatment of a 0.5–1 cm margin of apparently normal tissue adjacent to the lesion in order to eliminate microscopic foci of disease and the uncertainty which often exists regarding the precise location of actual tumor margins Citation[24]. In this case, accurate knowledge of the coagulated volume produced is important for optimizing treatment planning and energy delivery.
Figure 6. Gray-scale ultrasound cannot show the coagulation lesion in tissue mimicking phantom. The air in the electrode hole can shows on the sonogram (arrowhead). As the temperatures approach and exceed 100°C, gray-scale ultrasound can demonstrate the air-echo with a hyperechoic pattern in a heated volume (arrow) immediately after heating.
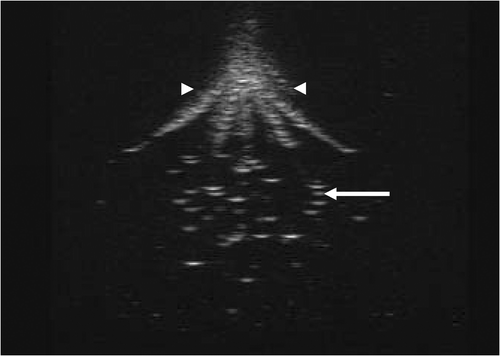
In order to investigate and develop new techniques, and also to improve those currently employed, research can call upon phantoms and clinical and experimental studies. The former are a powerful tool in this type of investigation, since they provide vital information on the electrical and thermal behavior of ablation rapidly and at low cost, quantifying the effect of various factors on the temperature distribution Citation[25]. Consequently, they facilitate the assessment of the feasibility of new electrode geometries, new protocols for delivering electrode geometries, and new protocols for delivering electrical power. Since that the results of this study might be useful not only for development and characterization of RFA devices, but also for the experimental validation of computer models Citation[25].
Figure 7. The coagulation lesion on the T2-weighted image of MRI has a similar shape and size corresponding to the gross view (pH = 4.5): (A) Coagulation lesion is an ellipsoid with hypo-intensity signal on coronal T2-weighted image of MRI; (B) Gross view of coagulation lesion (front view); (C) Coagulation lesion is a round region with a hypo-intensity signal on the transverse T2-weighted image of MRI; and (D) Gross view of the coagulation lesion (transverse-section).
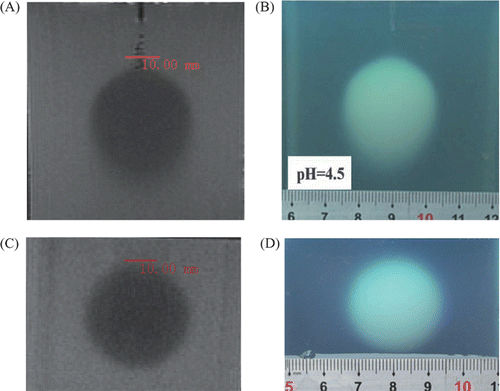
A phantom material with tissue-mimicking electrical and thermal properties is essential for the development and characterization of the RFA devices and for clinically related activities such as quality assurance, device comparisons and treatment verification. An ideal tissue-mimicking phantom for RFA should have a homogeneous texture and the physical properties, such as mass density, specific heat capacity, thermal conductivity, and electrical conductivity, have to meet the biological tissue. In the present study, the mass density, specific heat capacity, and the electrical conductivity are similar to those of the human liver. In addition, the phantom exhibits properties at room temperature which are comparable to those of the parenchymal type represented at body temperature. A polyacrylamide base is its stability at the high temperatures experienced in thermal therapy (at top of 110°C). This also was evident in the present study. The generator was operated in average mode (A mode) with the target temperature of 80°C. The temperature displays showed the temperatures around the probes of the electrode which were close to the dispersive ground pad exceeded 80°C and reached the high temperature of 100°–110°C during the 7 minutes of heating time. However the phantom didn’t melt and collapse. So the PAG phantom is more favorable for RFA study than other gel materials, such as agar or gelatin, which have lower melting temperatures. The dissolved BSA in this phantom underwent thermal coagulation at the same range of biological tissue (50°–60°C) by the adjustment of the pH (4.3–4.7), resulting in a region of irreversible thermal damage and, therefore, a permanent record of the volume where the temperature exceeded 50°–60°C. The phantom is an optical transparent solid form gel with homogeneous texture. Thus, the dynamic process of the coagulation lesion development can be recorded by digital camera or video recorder during heating (), providing a convenient way to analyze the coagulation lesions after heating.
Cell death occurs completely and rapidly above the coagulation temperature threshold, and there are various regions of distinct structural changes in tissue, including ablation, vaporization, thermal coagulation and red zone Citation[26]. A red zone appears in tissue peripheral to the coagulation boundary in the thermal lesions after RFA heating. This region is characterized by damage to the vasculature, including hemorrhage, increased blood flow, and/or hemostasis when temperature exceeded 50°C. And the extent of thermal necrosis seems to be related to the outer boundary of the red thermal damage zone in tissue Citation[26]. The ivory white coagulation lesions in the phantom demonstrate a well-defined isothermal line to assess the lesion boundary from the temperature distribution. For the pH 4.3 phantom, the coagulation lesion is enclosed by approximately 50°C isothermal surface, which represents the boundary of the red zone in the heating volume of biological tissue. As for the pH 4.7 phantom, the coagulation lesion is enclosed by approximately 60°C isothermal surface, which represents the boundary of the coagulation zone in the heating volume biological tissue. Therefore, pH 4.3–4.7 phantom can be used to evaluate the necrosis lesions produced by RFA devices.
Like RFA of biological tissue, gray-scale ultrasound cannot show the coagulation lesion in phantoms. This may result from the slender change of the acoustic properties, such as acoustic impedance, before and after the protein (BSA) coagulation. However, the air-echo can be demonstrated in a hyperechoic pattern on sonogram in coagulation zone (). This is because water vapor is formed, and steam collects in small pockets forming vacuoles in the phantom when the temperatures approach and exceed 100°C during heating. The BSA coagulation resulted in a reduction of T2 which provided sufficient contrast for the BSA coagulation resulting in a reduction in T2-weighted MR imaging of the volume of thermal coagulation with uncoagulated regions Citation[11],Citation[17]. illustrates the coagulation lesion as a hypo-intensity signal on the T2-weighted MR image, which demonstrates a similar shape and size corresponding to the gross view. Therefore, MR imaging has the capability to record the three-dimensional geometry of the coagulated volume, providing a powerful tool for developing RFA technology with adjustable power deposition, quality control and treatment verification in thermal therapy.
The thermal conductivity was not measured in this study. However, the phantom is expected to have similar properties to the egg-white PAG phantom, which was shown previously to have thermal conductivity of 0.59 ± 0.06 W m−1 °C−1 Citation[27]. This value is very similar to that of water, which has the thermal conductivity of 0.60 W m−1 °C−1 or PAG without protein (0.59 ± 0.06 W m−1 °C−1) Citation[28], since the water phantom is the most abundant ingredient (approximately 76.5% by weight). At frequencies used by RFA devices (400–500 kHz), energy is transferred primarily through electrical conduction and not the capacitive coupling. Therefore, electrical conductivity is a vital parameter for RFA and not the dielectric constant. Thus, the dielectric constant of the phantom was not measured in the present study. For higher frequencies of electromagnetic devices, such as microwave, dielectric constant is a necessary parameter and should be given.
The recipe in the present study was somewhat different than that proposed and thoroughly evaluated by McDonald et al. Citation[17], including ingredients and dosage. However, the coagulation temperatures of the phantoms were also kept within the range of the biological tissue thermal damage (50°–60°C) by adjusting the pH of the phantom (4.3–4.7). This also demonstrated that the coagulation temperatures of the phantoms were mainly dependent upon pH value Citation[17], although the addition of the other ingredients might have a minor influence upon the temperatures. Glycerol is one ingredient of the intralipid. In order to keep an ideal transparency of the phantom for dynamic visualization of coagulation lesion in RFA, glycerol was substituted for intralipid which was introduced by McDonald et al. Citation[17]. Glycerol is also a good lubricant for removing the phantom from the mould easily, keeping the phantom perfect for RFA. In addition, glycerin is hydrophilic so that the phantom can maintain homogeneity. We chose 0.2 M citrate buffer concentration for phantom fabrication because a buffer concentration of 0.2 M can hold constant as other ingredients are added Citation[17]. The citrate buffer contributes not only to lower the pH and hence the coagulation temperature of BSA but also provides the favorable electrical conductivity for RFA. And 2% BSA concentration in our study demonstrated the coagulation lesions in phantoms both good visualization in gross view and good contrast on T2-weighted MR image between the coagulated lesions and uncoagulated regions (), which is identical to the report by McDonald et al. Citation[17]. For RFA experimental investigations, the total concentrations of acrylamide and N, N methylene-bis-acrylamide and cross-linking concentration of the N, N methylene-bis-acrylamide in our study are 10% and 5%, respectively, enabling the phantoms to be hard enough to stand well on the dispersive ground pad and tolerate insertion of electrodes without cracking or splitting. Although the total concentration of acrylamide and N, N methylene-bis-acrylamide and cross-linking concentration of the N, N methylene-bis-acrylamide in the present study is higher than those introduced by McDonald et al. Citation[17] (10% vs. 7% and 5% vs. 2%, respectively), the premature coagulation did not occur in our phantom fabrication. Polymerization begins to occur in minutes following the addition of the initiator-activator pair and phantoms should remain refrigerated for a number of hours to preclude premature coagulation. Both acrylamide and N, N methylene-bis-acrylamide are moderately neurotoxic substances. Even after the polymerization, small amounts of acrylamide monomers remain in phantoms. Therefore, the phantom fabrication and experiment must be handled in a fume hood with appropriate safety attire (e.g. gloves, laboratory coat). This may be a disadvantage of the phantom in comparison with the other thermal gel materials.
Conclusions
The tissue-mimicking phantom described in this study is a useful material for the measurement of thermal coagulation patterns in RFA investigation. The coagulation temperature can be adjusted to simulate that of a specific soft tissue by changing the pH from 4.3 to 4.7, and the physical properties (density, electrical conductivity and specific heat) of the phantom are similar to those of the soft tissue, such as human liver, enabling it to be useful for RFA investigation. The phantom is transparent and the development and distribution of the coagulation lesions can be permanently recorded by video for further study. The phantom also exhibits a large reduction in T2 upon thermal coagulation, enabling the use of T2-weighted MR images to measure the three-dimensional geometry of the heated volume.
Acknowledgements
This study was supported in part by the Science Research Foundation (No. 03JC14051) from the Science and Technology Commission of Shanghai Municipality, Shanghai, China and in part by a Science and Technology Development Foundation (No. 034048) from the Shanghai Municipal Health Bureau, Shanghai, China.
Declaration of interest: The authors report no conflicts of interest. The authors alone are responsible for the content and writing of the paper.
References
- Doukas G, Samani NJ, Alexiou C, Oc M, Chin DT, Stafford PG, Ng LL, Spyt TJ. Left atrial radiofrequency ablation during mitral valve surgery for continuous atrial fibrillation: A randomized controlled trial. JAMA 2005; 294: 2323–2329
- Uehara T, Hirooka M, Ishida K, Hiraoka A, Kumagi T, Kisaka Y, Hiasa Y, Onji M. Percutaneous ultrasound-guided radiofrequency ablation of hepatocellular carcinoma with artificially induced pleural effusion and ascites. J Gastroenterol 2007; 42: 306–311
- Jacobsohn KM, Ahrar K, Wood CG, Matin SF. Is radiofrequency ablation safe for solitary kidneys?. Urology 2007; 69: 819–823
- Kojima H, Tanigawa N, Kariya S, Komemushi A, Shomura Y, Sawada S. Clinical assessment of percutaneous radiofrequency ablation for painful metastatic bone tumors. Cardiovasc Intervent Radiol 2006; 29: 1022–1026
- Thanos L, Mylona S, Pomoni M, Athanassiadi K, Theakos N, Zoganas L, Batakis N. Percutaneous radiofrequency thermal ablation of primary and metastatic lung tumors. Eur J Cardiothorac Surg 2006; 30: 797–800
- Oura S, Tamaki T, Hirai I, Yoshimasu T, Ohta F, Nakamura R, Okamura Y. Radiofrequency ablation therapy in patients with breast cancers 2 cm or less in size. Breast Cancer 2007; 14: 48–54
- Shariat SF, Raptidis G, Masatoschi M, Bergamaschi F, Slawin KM. Pilot study of radiofrequency interstitial tumor ablation (RITA) for the treatment of radio-recurrent prostate cancer. Prostate 2005; 65: 260–267
- Kim YS, Rhim H, Tae K, Park DW, Kim ST. Radiofrequency ablation of benign cold thyroid nodules: Initial clinical experience. Thyroid 2006; 16: 361–367
- Goldberg SN. Radiofrequency tumor ablation: Principles and techniques. Eur J Ultrasound 2001; 13: 129–147
- Mirza AN, Fornage BD, Sneige N, Kuerer HM, Newman LA, Ames FC, Singletary SE. Radiofrequency ablation of solid tumors. Cancer J 2001; 7: 95–102
- Bouchard LS, Bronskill MJ. Magnetic resonance imaging of thermal coagulation effects in a phantom for calibrating thermal therapy devices. Med Phys 2000; 27: 1141–1145
- Lazebnik M, Madsen EL, Frank GR, Hagness SC. Tissue-mimicking phantom materials for narrowband and ultrawideband microwave applications. Phys Med Biol 2005; 50: 4245–4258
- Lobo SM, Afzal KS, Ahmed M, Kruskal JB, Lenkinski RE, Goldberg SN. Radiofrequency ablation: Modeling the enhanced temperature response to adjuvant NaCl pretreatment. Radiology 2004; 230: 175–182
- Kuroda M, Kato H, Hanamoto K, Shimamura K, Uchida T, Wang Y, Akaki S, Asaumi J, Himei K, Takemoto M, et al. Development of a new hybrid gel phantom using carrageenan and gellan gum for visualizing three-dimensional temperature distribution during hyperthermia and radiofrequency ablation. Int J Oncol 2005; 27: 175–184
- Lafon C, Zderic V, Noble ML, Yuen JC, Kaczkowski PJ, Sapozhnikov OA, Chavrier F, Crum LA, Vaezy S. Gel phantom for use in high-intensity focused ultrasound dosimetry. Ultrasound Med Biol 2005; 31: 1383–1389
- Takegami K, Kaneko Y, Watanabe T, Maruyama T, Matsumoto Y, Nagawa H. Polyacrylamide gel containing egg white as new model for irradiation experiments using focused ultrasound. Ultrasound Med Biol 2004; 30: 1419–1422
- McDonald M, Lochhead S, Chopra R, Bronskill MJ. Multi-modality tissue-mimicking phantom for thermal therapy. Phys Med Biol 2004; 49: 2767–2778
- Graham SJ, Chen L, Leitch M, Peters RD, Bronskill MJ, Foster FS, Henkelman RM, Plewes DB. Quantifying tissue damage due to focused ultrasound heating observed by MRI. Magn Reson Med 1999; 41: 321–328
- Peters RD, Chan E, Trachtenberg J, Jothy S, Kapusta L, Kucharczyk W, Henkelman RM. Magnetic resonance thermometry for predicting thermal damage: An application of interstitial laser coagulation in an in vivo canine prostate model. Magn Reson Med 2000; 44: 873–883
- McDannold NJ, King RL, Jolesz FA, Hynynen KH. Usefulness of MR imaging-derived thermometry and dosimetry in determining the threshold for tissue damage induced by thermal surgery in rabbits. Radiology 2000; 216: 517–523
- Puccini S, Bär NK, Bublat M, Kahn T, Busse H. Simulations of thermal tissue coagulation and their value for the planning and monitoring of laser-induced interstitial thermotherapy (LITT). Magn Reson Med 2003; 49: 351–362
- Duck F. Physical properties of tissue a comprehensive reference book. Academic Press, New York 1990; 167–223
- Gabriel C, Gabriel S, Corthout E. The dielectric properties of biological tissues: I. Literature survey. Phys Med Biol 1996; 41: 2231–2250
- Goldberg SN, Gazelle GS, Mueller PR. Thermal ablation therapy for focal malignancy: A unified approach to underlying principles, techniques, and diagnostic imaging guidance. AJR Am J Roentgenol 2000; 174: 323–331
- Berjano EJ. Theoretical modeling for radiofrequency ablation: State-of-the-art and challenges for the future. Biomed Eng Online 2006; 5: 24
- Thomsen SL. Mapping of thermal injury in biologic tissues using quantitative pathologic techniques. Proceedings of SPIE. SPIE, Bellingham, WA 1999; 3594: 82–95, ETATS-UNIS
- Divkovic GW, Liebler M, Braun K, Dreyer T, Huber PE, Jenne JW. Thermal properties and changes of acoustic parameters in an egg white phantom during heating and coagulation by high intensity focused ultrasound. Ultrasound Med Biol 2007; 33: 981–986
- Davidson SR, Sherar MD. Measurement of the thermal conductivity of polyacrylamide tissue-equivalent material. Int J Hyperthermia 2003; 19: 551–562