Abstract
In this study, to better understand the molecular mechanism underlying cellular responses to mild hyperthermia, we investigated gene expression patterns and genetic networks in human myelomonocytic lymphoma U937 cells using high-density oligonucleotide microarrays and computational gene expression analysis tools. The cells were incubated at 41°C for 30 min (mild hyperthermia treatment) and then at 37°C for 0–6 h. Although the mild hyperthermia treatment of the cells did not induce apoptosis, significant increases in the protein expression levels of heat shock proteins (HSPs), namely, Hsp27, Hsp40 and Hsp70, were observed following the activation of heat shock factor-1. Of the 22,283 probe sets analyzed, 423 probe sets were up-regulated and 515 probe sets were down-regulated by >1.5-fold in the cells 3 h post-treatment. Computational gene network analysis demonstrated that the significant genetic network A that contained many HSPs such as DNAJB1, HSPA1A, and HSPA1B was associated with cellular function and maintenance, post-transcriptional modification, or protein folding. Moreover, the significant genetic network B whose core contained v-myc myelocytomatosis viral oncogene homolog (MYC) was associated with cell morphology, cell cycle, and cellular development. The expression levels of nine selected genes were comparable to those determined by microarray analysis with real-time quantitative PCR assay. The present results indicate that mild hyperthermia affects the expression of a large number of genes and provides additional novel insights into the molecular basis of mild hyperthermia in cells.
Introduction
Hyperthermia has been considered a promising approach in cancer therapy. The temperatures used in heat treatments for 30–60 min are in the range of 40°–45°C in the case of locoregional treatment, and up to 42°C in the case of whole-body hyperthermia Citation[1]. Although interest in the use of hyperthermia alone decreased mainly because of problems with heating systems, hyperthermia and its use in combination with chemotherapy and radiotherapy brought a renewed interest in the field Citation[2–5]. The rationale underlying hyperthermia is the finding that temperatures over 42.5°C are highly cytotoxic to tumor cells, particularly in an environment with a low pO2 and low pH conditions, which are typically found in tumor tissues owing to insufficient blood perfusion. Cells in this type of environment are also resistant to radiotherapy, thus making hyperthermia valuable in augmenting the therapeutic outcome of combined treatments Citation[6]. On the other hand, although mild hyperthermia in the range from 39° to 41°C alone does not induce cytotoxicity in tumor cells, mild hyperthermia is reported to show a synergism with radiotherapy and anti-cancer drugs Citation[7–9].
On the molecular aspect of hyperthermia or mild hyperthermia, heat shock proteins (HSPs) are highly conserved proteins whose syntheses are induced by a variety of stresses, particularly hyperthermia treatment. HSPs have been recognized as molecular chaperones, which are the primary cellular defense against damage to the proteome, initiating refolding of denatured proteins and regulating degradation after severe protein damage. HSPs are primordial proteins involved in cytoprotection during stress in all cellular organisms Citation[10],Citation[11]. HSPs protect cells both by limiting the effects of protein-damaging agents through protein chaperoning and refolding and by directly blocking the pathways of cell death such as apoptosis and necrosis Citation[12]. In addition, recent studies have shown the importance of HSPs in immune reactions and suggest the utilization of HSP chaperone tumor antigens for cancer immunotherapy based on hyperthermia Citation[13–15].
As mentioned above, hyperthermia induces complex cellular responses and changes in signal transduction; hence, the overall responses are difficult to examine using ordinary methods. A DNA microarray technique, ‘gene expression profiling’ Citation[16], now offers tremendous potential for characterizing gene expression patterns associated with different diseases as well as the biological responses during physical and chemical stresses in cells. There were several studies carried out utilizing this technique for identifying changes in gene expression induced by hyperthermia Citation[17–22]. Here, for a better understanding of the molecular mechanism underlying cellular responses to mild hyperthermia, we examined gene expression patterns in human leukemia cells exposed to mild hyperthermia using a GeneChip® analyzing system. Furthermore, the functional relationships among the candidate genes were examined using Ingenuity Pathway Analysis tools.
Materials and methods
Cell culture
U937 cells, a human myelomonocytic lymphoma cell line, were obtained from the Human Science Research Resource Bank, Human Science Foundation (Tokyo, Japan). The cells were grown in RPMI 1640 medium (Sigma-Aldrich, Milwaukee, WI) supplemented with 10% heat-inactivated fetal bovine serum (Invitrogen, Carlsbad, CA) at 37°C in humidified air with 5% CO2.
Mild hyperthermia treatment
Cells were collected and suspended in 3 ml of fresh medium in plastic culture tubes, and were exposed to 41°C (±0.05°C) for 30 min in a water bath (NTT-1200, Eyela, Tokyo). The temperature was monitored with a digital thermometer (No. 7563, Yokogawa, Tokyo) coupled to a thermocouple 0.8 mm in diameter during heating. After mild hyperthermia treatment, the cells were incubated for 0–6 h at 37°C in humidified air with 5% CO2.
Analysis of apoptosis
For detection of DNA fragmentation, cells were lysed with a lysis buffer (1 mM EDTA, 0.2% Triton X-100 and 10 mM Tris-HCl, pH 7.5) and centrifuged at 13,000 × g for 10 min. Subsequently, DNA in the supernatant and resuspended pellets in each sample were precipitated in 12.5% trichloroacetic acid at 4°C and quantified using diphenylamine reagent after hydrolysis in 5% trichloroacetic acid at 90°C for 20 min. The absorbance at 600 nm in each sample was determined after overnight color development using diphenylamine reagent. The percentage of fragmented DNA in each sample was calculated as the amount of DNA in the supernatant divided by that of total DNA of that sample (supernatant plus pellet) Citation[23],Citation[24]. The expression level of phosphatidylserine outside the plasma membrane of cells was determined using an Annexin V-FITC kit (Immunotech, Marseille, France). FITC-labeled annexin V and propidium iodide were added to the cell suspension. After incubation for 10 min in the dark, the cells were analyzed using a flow cytometer (Epics XL, Beckman Coulter K. K., Tokyo) Citation[23].
SDS-polyacrylamide gel electrophoresis and Western blotting
Cells were dissolved in lysis buffer (50 mM NaCl, 1% Nonidet P-40 and 50 mM Tris-HCl, pH 8.0) containing the protease inhibitor cocktail (Roche Diagonostics GmbH, Mannheim, Germany), and homogenized using an ultrasonic disruptor. SDS- polyacrylamide gel electrophoresis (PAGE) and Western blotting were carried out as described elsewhere Citation[25],Citation[26]. The primary antibodies used were as follows: a rabbit polyclonal anti-Hsp70 antibody (MBL Co., Ltd, Nagoya, Japan); a mouse monoclonal anti-Hsp40 antibody (MBL Co., Ltd); a goat polyclonal anti-Hsp60 antibody (Santa Cruz Biotechnology, Inc., CA); a rat monoclonal anti-Hsp90 antibody (MBL Co., Ltd); a rabbit polyclonal anti-Hsp27 antibody (Santa Cruz Biotechnology, Inc.); a rabbit polyclonal anti-heat shock transcription factor-1 (HSF1) antibody (Stressgen Bioreagents Co., Ann Arbor, MI); and a mouse monoclonal anti-glyceraldehyde 3-phosphate dehydrogenase (GAPDH) antibody (Organon Teknika Co., Durham, NC). Immunoreactive proteins were visualized using a luminescent image analyzer (LAS-1000 plus, Fujifilm Co., Tokyo) using an enhanced chemiluminescence detection system.
RNA isolation
Total RNA was extracted from cells using an RNeasy Total RNA Extraction kit (Qiagen, Valencia, CA) and treated with DNase I (RNase-free DNase kit, Qiagen) for 15 min at room temperature to remove residual genomic DNA.
High-density oligonucleotide microarray and computational gene expression analyses
Gene expression was analyzed using a GeneChip® system with a Human Genome U133 A array, which was spotted with 22,283 probe sets (Affymetrix, Santa Clara, CA). Samples for array hybridization were prepared as described in the Affymetrix GeneChip® Expression Technical Manual. Briefly, 5 µg of total RNA was used to synthesize double-stranded cDNA with a GeneChip® Expression 3′-Amplification Reagents One-Cycle cDNA Synthesis kit (Affymetrix). Biotin-labeled cRNA was then synthesized from the cDNA using GeneChip® Expression 3′-Amplification Reagents for IVT Labeling (Affymetrix). After fragmentation, the biotinylated cRNA was hybridized to the GeneChip array at 45°C for 16 h. The arrays were washed, stained with streptavidin-phycoerythrin, and scanned using a probe array scanner. The scanned chip was analyzed using GeneChip Analysis Suite Software (Affymetrix). The obtained hybridization intensity data were converted into a presence or an absence call for each gene, and changes in gene expression level between experiments were detected by comparison analysis. The data were further analyzed using GeneSpring (Silicon Genetics, Redwood City, CA) to extract the significant genes Citation[24],Citation[27],Citation[28].
To examine gene ontology, including biological processes, cellular components, molecular functions, and genetic networks, the obtained data were analyzed using Ingenuity Pathways Analysis tools (Ingenuity Systems, Mountain View, CA), a web-delivered application that enables the identification, visualization, and exploration of molecular interaction networks in gene expression data. The gene lists identified by GeneSpring containing Affymetrix gene ID and the natural logarithm of normalized expression signal ratios from GeneChip CEL files were uploaded into the Ingenuity Pathways Analysis system. Each gene identifier was mapped to its corresponding gene object in the Ingenuity Pathways Knowledge Base. These so-called focus genes were then used as a starting point for generating biological networks Citation[24],Citation[27],Citation[28].
Real-time quantitative PCR assay
Real-time quantitative PCR (qPCR) assay was performed on a Real-Time PCR system (Mx3000P, Stratagene Japan, Tokyo) using SYBR PreMix ExTaq (Takara Bio, Shiga, Japan) or qPCR Master Mix (for the use of TaqMan probes; Eurogentec, Seraing, Belgium) in accordance with the manufacturer's protocols. Reverse transcriptase reaction (Omniscript Reverse Transcriptase, Qiagen) was carried out with DNase-treated total RNA using an oligo (dT)16 primer. Real-time qPCR assay was performed using the specific primers listed in . Each mRNA expression level was normalized to the mRNA expression level of GAPDH Citation[17].
Table I. Nucleotide sequences of primers and a probe for target genes.
Results
Effects of mild hyperthermia on U937 cells
Our previous study demonstrated that apparent DNA fragmentation induced by hyperthermia was detected at temperatures greater than 43°C, but below this temperature, DNA fragmentation hardly increased in U937 cells, a human myelomonocytic cell line Citation[23]. When U937 cells were incubated at different temperatures for 30 min, DNA fragmentation was not observed at 41 and 42°C, but significantly increased at 43 and 44°C (). This observation is agreement with our previous study Citation[23]. Moreover, the phosphatidylserine externalization was examined using the Annexin V-FITC kit. When the cells were treated at 43°C for 30 min, the percentage of apoptotic cells was significantly increased in a time-dependent manner. On the other hand, the percentages of apoptotic cells 0–6 h after mild hyperthermia (41°C for 30 min) was very low (). These data indicated that mild hyperthermia at 41°C for 30 min induced no cell apoptosis.
Figure 1. Effects of hyperthermia on apoptosis in U937 cells. (A) U937 cells were incubated at 37°–44°C for 30 min and then at 37°C for 6 h. DNA fragmentation assay was carried out. Data are presented as mean ± S.D. (n = 3). (B) The cells were incubated at 41° or 43°C for 30 min and then at 37°C for 0–6 h. The phosphatidylserine externalization was examined using the Annexin V-FITC kit. Data are presented as mean ± SD. (n = 3–4).
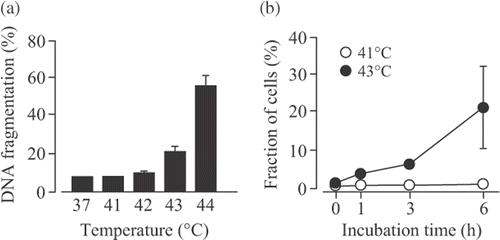
It has been reported that the increases in the protein expression levels of HSPs, Hsp27, Hsp40 and Hsp70, and the activation of HSF1 are observed in human neuroblastoma IMR-32 cells treated with mild hyperthermia (42°C for 2 h) Citation[29]. The protein expression levels of HSF1 and HSPs in U937 cells treated with mild hyperthermia were monitored by Western blotting. The treatment of the cells with mild hyperthermia (41°C for 30 min) resulted in a transient mobility shift of HSF1 0.5 and 1 h after the treatment (). The protein expression levels of Hsp70 significantly increased in the cells cultured for 3 and 6 h. The increase in the protein expression levels of Hsp27 and Hsp40 was observed from 0.5 h after the mild hyperthermia treatment. On the other hand, no significant changes in the protein expression levels of Hsp60 and Hsp90 were observed (). These observations suggest that the induction of Hsp27, Hsp40 and Hsp70 by mild hyperthermia is mediated by the activation of HSF1 as observed in IMR-32 cells Citation[29].
Identification of genes responsive to mild hyperthermia
To identify genes that are affected by mild hyperthermia, we carried out GeneChip analysis of cells treated with or without mild hyperthermia at 41°C for 30 min. Of the 22,283 probe sets analyzed, we identified 13,216 (percentage present: 58.2%) and 13,390 (59.0%) probe sets expressed in control cells and the cells treated with mild hyperthermia, respectively, indicating that the number of expressed genes was approximately constant between these samples. Genes that were up- or down-regulated by >1.5-fold were examined using GeneSpring software. When the gene expression levels were compared, the total number of probe sets that were found to be differentially expressed as induced by the mild hyperthermia treatment was 938 (423 up-regulated and 515 down-regulated probe sets); that is, 4.2% of probe sets were affected by the mild hyperthermia treatment. The top 20 of genes that were up- or down-regulated by the mild hyperthermia treatment is shown in or , respectively. The complete list of genes from all samples is available on the Gene Expression Omnibus (http://www.ncbi.nlm.nih.gov/geo/query/acc.cgi?acc = GSE10043) and our homepage (http://www.lsrc.u-toyama.ac.jp/mgrc/html/microarray.html).
Table II. The top 20 of genes that were up-regulated by heat.
Table III. The top 20 of genes that were down-regulated by heat.
Functional and pathway analyses
The functional and pathway analyses of differentially expressed genes in U937 cells treated with mild hyperthermia were carried out using the Ingenuity Pathways Knowledge Base. On the basis of the significance and number of genes, the top three molecular and cellular functional categories affected by the mild hyperthermia treatment were determined to be cellular function and maintenance, cell cycle, and cell death. The genes associated with these functional categories are listed in . Unfolded-protein-response-related genes such as those encoding DNAJA1 and DNAJB1 (Hsp40 homologs), HSPA6, HSPA1A, HSPA1B, and HSPA1L (heat shock 70 kDa proteins), HSPB1 (heat shock 27 kDa protein 1), HSPH1 (heat shock 105 kDa/110 kDa protein 1), PPP1R15A (protein phosphatase 1, regulatory (inhibitor) subunit 15 A), and SERPINH1 (serpin peptidase inhibitor, clade H (heat shock protein 47), member 1) in the category of the cellular function and maintenance were all up-regulated. On the other hand, there were many genes that were up- and down-regulated in the categories of cell cycle and cell death ().
Table IV. Genes in functional categories.
Next, to determine the biologically relevant networks and pathways of the genes identified here, pathway analysis of the differentially expressed genes was carried out using the Ingenuity Pathways Analysis Knowledge Base. Several significant genetic networks were determined or identified in the differentially expressed genes affected by mild hyperthermia. shows the significant genetic network A that contained many HSPs such as DNAJB1, HSPA1A, HSPA1B, HSP90AA1 (heat shock protein 90 kDa alpha (cytosolic), class A member 1), and HSP90AB1 (heat shock protein 90 kDa alpha (cytosolic), class B member 1) (score, 46; 35 genes). This network was associated with molecular and cellular functions including cellular function and maintenance, post-transcriptional modification, and protein folding. Moreover, the significant genetic network B whose core contained MYC (score, 46; 35 genes) was found to be associated with molecular and cellular functions including cell morphology, cell cycle, and cellular development ().
Figure 3. Genetic network A. Cells were incubated at 41°C for 30 min (mild hyperthermia treatment) and then at 37°C for 3 h. GeneChip analysis was performed. Probe sets that were differentially expressed were analyzed using Ingenuity Pathways Analysis software. The network is shown graphically as nodes (genes) and edges (the biological relationships between the nodes).
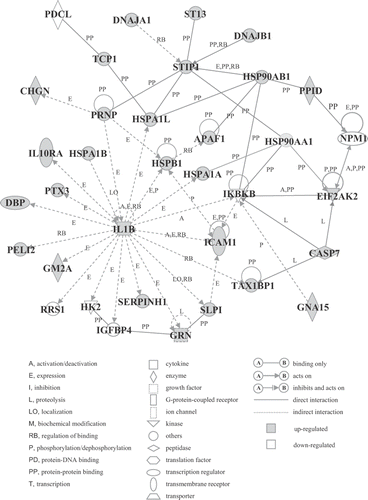
Verification of differentially expressed genes
To verify the results of the microarray experiments, real-time qPCR assay was performed. The expression levels of nine selected genes were comparable to those determined by microarray analysis, the expression levels of these genes significantly increased up to 6 h after the mild hyperthermia treatment. In addition, the peak of the expression level was reached 1 h after the treatment ().
Figure 5. Verification of microarray results by real-time qPCR assay. Cells were incubated at 41°C for 30 min (mild hyperthermia treatment) and then at 37°C for 1–6 h. Real-time qPCR assay was performed. (A) BAG3; (B) DNAJB1; (C) HSPA1A; (D) HSPA1B; (E) HSPA1L; (F) IL7R; (G) HMOX1; (H) SERPINE1; (I) SERPINH1. Each mRNA expression level was normalized to GAPDH expression level. The shadows indicate mild hyperthermia treatment. Data are presented as mean ± SD. (n = 4).
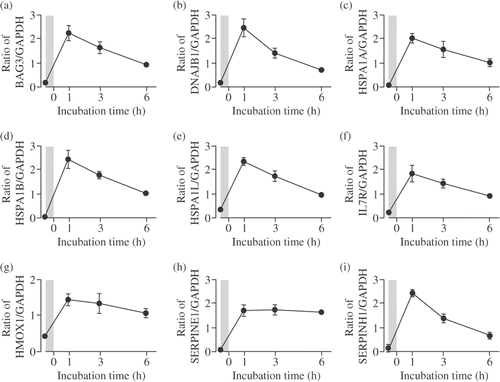
Discussion
Microarray technologies offer the opportunity to gain insights into global gene expression profiles associated with different diseases, as well as the biological responses during physical and chemical stresses in cells Citation[16–22]. Although the identification of individual genes that are differentially expressed is very important, there has recently been an increasing need to move beyond this level of analysis. In this study, we identified genes and genetic networks responsive to mild hyperthermia in human leukemia U937 cells using high-density oligonucleotide microarrays and computational gene expression analysis tools. To the best of our knowledge, this is the first report on the identification of genetic networks in cells treated with mild hyperthermia.
In this study, although the treatment of U937 cells with mild hyperthermia at 41°C for 30 min did not induce apoptosis (), a significant increase in the protein expression levels of Hsp27, Hsp40 and Hsp70 was observed following the mobility shift of HSF1 (). The mobility shift of HSF1 detected as an upward band shift by Western blotting is due to phosphorylation of HSF1, indicating the activation of HSF1 Citation[30]. Under this condition, 938 differentially expressed probe sets were identified in U937 cells treated with mild hyperthermia using a GeneChip® system with a human genome array containing 22,283 probe sets. Changes in gene expression of selected genes were confirmed by real-time qPCR (). Moreover, the functional analysis of differentially expressed genes using the Ingenuity Pathways Knowledge Base demonstrated that molecular and cellular functional categories affected by the mild hyperthermia were determined to be cell function and maintenance, cell cycle and cell death (). Mild hyperthermia up-regulated both many apoptosis-inducing genes such as apoptotic peptidase activating factor 1 (APAF1) Citation[31], caspase 7, apoptosis-related cysteine peptidase (CASP7) Citation[32] and thioredoxin interacting protein (TXNIP) Citation[33] and many apoptosis-inhibiting genes such as heme oxygenase (decycling) 1 (HMOX1) Citation[34], HSPA1B Citation[12],Citation[35] and HSPB1 Citation[12],Citation[35]. It is presumed that apoptosis was not observed in the U937 cells following the 41°C (30 min) treatment because of the up-regulation of the apoptosis-inhibiting genes.
To date, it has been demonstrated that genes that were modified by hyperthermia were identified in a wide variety of types of cell Citation[17–22]. Our previous study showed that 42 of 16,600 genes were modified in U937 cells after hyperthermia at 44°C for 10 min Citation[17]. Furthermore, the hyperthermia treatment of human cervical carcinoma HeLa cells at 44°C for 60 min induced 664 of 12,814 genes Citation[21]. Zhou et al. Citation[19] reported that 1,448 of 24,650 genes were heat-responding genes in human umbilical vein cells induced after hyperthermia treatment at 44°C for 60 min. Sonna et al. Citation[22] also reported that 395 of 12,600 sequences were identified in human peripheral blood mononuclear cells treated with hyperthermia at 43°C for 20 min. These findings commonly demonstrated that the expression levels of unfolded-protein-response-related genes such as HSPs increased in cells treated with heat. In this study, although the differentially expressed genes including unfolded-protein-response-related genes were identified as in U937 cells treated with mild hyperthermia, the majority of the genes identified here were not observed in the previous studies Citation[17–22]. This discrepancy may be because of different experimental conditions, such as the type of microarray, hyperthermia, and cell origin.
Of particular interest is our identification of networks of genes responsive to mild hyperthermia. Network A () whose core contained interleukin 1, beta (IL1B) and stress-induced phosphoprotein 1 (STIP1) was associated with molecular and cellular functions including cellular function and maintenance, post-transcriptional modification, and protein folding. Regarding this network, there are many reports concerning the relationships between HSPs and IL1B or STIP1, for example the IL1B protein increases the expression level of HSPA1A Citation[36]; the IL1B protein is involved in the expression of HSPA1L Citation[37]; the HSP90AB1 protein enhances the binding of Hsp70 proteins and the STIP1 protein Citation[38]; protein-protein binding of DNAJB1-STIP1 Citation[39]. Genetic network B () whose core contained MYC was found to be associated with molecular and cellular functions including cell morphology, cell cycle, and cellular development. It has been demonstrated that the expression of MYC was negatively regulated by docking protein 2, 56 kDa (DOK2) Citation[40]. Furthermore, the expression of cyclin D2 (CCND2) Citation[41], MAX-binding protein (MNT) Citation[42] and transferrin receptor (p90, CD71) (TFRC) Citation[43] was reported to be positively regulated by MYC. Taken together, the identified genes that were differentially expressed and/or belonged to the genetic networks may be closely associated with the hyperthermia-induced cellular responses. It has been reported that mild hyperthermia treatment potentiates the efficacies of cell killing and antitumor effects due to a synergism with radiotherapy and anti-cancer drugs Citation[7–9]. However, at present, the interaction between gene expression and the potentiation of the efficacies is not fully understood. There are important questions that need to be elucidated in future studies.
The present results indicate that mild hyperthermia affects the expression of a large number of genes and provide additional novel insights into the molecular basis of the effect of mild hyperthermia on cells. Mild hyperthermia is reported to show a synergism with radiotherapy and anti-cancer drugs.
Acknowledgement
Yoshiaki Tabuchi and Ichiro Takasaki contributed equally to this work. This study was supported in part by a Grant-in-Aid from the Japanese Ministry of Education, Culture, Sports, Science, and Technology.
Declaration of interest: The authors report no conflicts of interest. The authors alone are responsible for the content and writing of the paper.
References
- Hall EJ. Hyperthermia. Radiobiology for the radiologist, 5th, EJ Hall. Lippincott Williams & Wilkins, New York 2000; 495–520
- Overgaard J. The current and potential role of hyperthermia in radiotherapy. Int J Radiat Oncol Biol Phys 1989; 16: 535–549
- Harima Y, Nagata K, Harima K, Ostapenko VV, Tanaka Y, Sawada S. A randomized clinical trial of radiation therapy versus thermoradiotherapy in stage IIIB cervical carcinoma. Int J Hyperthermia 2001; 17: 97–105
- van der Zee J, González González D, van Rhoon GC, van Dijk JD, van Putten WL, Hart AA. Comparison of radiotherapy alone with radiotherapy plus hyperthermia in locally advanced pelvic tumours: A prospective, randomised, multicentre trial. Dutch Deep Hyperthermia Group. Lancet 2000; 355: 1119–1125
- van der Zee J, González GD. The Dutch Deep Hyperthermia Trial: Results in cervical cancer. Int J Hyperthermia 2002; 18: 1–12
- Song CW, Park HJ, Lee CK, Griffin R. Implications of increased tumor blood flow and oxygenation caused by mild temperature hyperthermia in tumor treatment. Int J Hyperthermia 2005; 21: 761–767
- Vujaskovic Z, Song CW. Physiological mechanisms underlying heat-induced radiosensitization. Int J Hyperthermia 2004; 20: 163–174
- Ahmed K, Zhao QL, Matsuya Y, Yu DY, Salunga TL, Nemoto H, Kondo T. Enhancement of macrosphelide-induced apoptosis by mild hyperthermia. Int J Hyperthermia 2007; 23: 353–361
- Shioura H, Hayashi S, Matsumoto H, Kitai R, Ohtsubo T, Nishida T, Zhang SW, Yoshida M, Ishii Y, Kano E. The effects of combined treatments with low hyperthermia and bleomycin on survivals of murine L cells. J Exp Clin Cancer Res 1997; 16: 147–152
- Lindquist S, Craig EA. The heat-shock proteins. Ann Rev Genet 1988; 22: 631–677
- Georgopoulos C, Welch WJ. Role of the major heat shock proteins as molecular chaperones. Ann Rev Cell Biol 1993; 9: 601–634
- Beere HM. ‘The stress of dying’: The role of heat shock proteins in the regulation of apoptosis. J Cell Science 2004; 117: 2641–2651
- Ménoret A, Chandawarkar R. Heat-shock protein-based anticancer immunotherapy: An idea whose time has come. Semin Oncol 1998; 25: 654–660
- Srivastava PK, Menoret A, Basu S, Binder RJ, McQuade KL. Heat shock proteins come of age: Primitive functions acquire new roles in an adaptive world. Immunity 1998; 8: 657–665
- Ito A, Honda H, Kobayashi T. Cancer immunotherapy based on intracellular hyperthermia using magnetite nanoparticles: A novel concept of ‘heat-controlled necrosis’ with heat shock protein expression. Cancer Immunol Immunother 2006; 55: 320–328
- DeRisi J, Penland L, Brown PO, Bittner ML, Meltzer PS, Ray M, Chen Y, Su YA, Trent JM. Use of a cDNA microarray to analyse gene expression patterns in human cancer. Nat Genet 1996; 14: 457–460
- Wada S, Tabuchi Y, Kondo T, Cui ZG, Zhao QL, Takasaki I, Salunga TL, Ogawa R, Arai T, Makino K, et al. Gene expression in enhanced apoptosis of human lymphoma U937 cells treated with the combination of different free radical generators and hyperthermia. Free Rad Res 2007; 41: 73–81
- Hirano H, Tabuchi Y, Kondo T, Zhao Q-L, Ogawa R, Cui Z-G, Feril LB, Jr, Kanayama S. Analysis of gene expression in apoptosis in human lymphoma U937 cells induced by heat shock and the effects of α-phenyl N-tertbutylnitrone (PBN) and its derivatives. Apoptosis 2005; 10: 331–340
- Zhou M, Zhang A, Lin B, Liu J, Xu LX. Study of heat shock response of human umbilical vein endothelial cells (HUVECs) using cDNA microarray. Int J Hyperthermia 2007; 23: 225–258
- Narita N, Noda I, Ohtsubo T, Fujieda S, Tokuriki M, Saito T, Saito H. Analysis of heat-shock related gene expression in head-and-neck cancer using cDNA arrays. Int J Radiat Oncol Biol Phys 2002; 53: 190–196
- Kato N, Kobayashi T, Honda H. Screening of stress enhancer based on analysis of gene expression profiles: Enhancement of hyperthermia-induced tumor necrosis by an MMP-3 inhibitor. Cancer Science 2003; 94: 644–649
- Sonna LA, Gaffin SL, Pratt RE, Cullivan ML, Angel KC, Lilly CM. Effect of acute heat shock on gene expression by human peripheral blood mononuclear cells. J App Phys 2002; 92: 2208–2220
- Kameda K, Kondo T, Tanabe K, Zhao QL, Seto H. The role of intracellular Ca2+ in apoptosis induced by hyperthermia and its enhancement by verapamil in U937 cells. Int J Radiat Oncol Biol Phys 2001; 49: 1369–1379
- Salunga TL, Tabuchi Y, Takasaki I, Feril LB, Jr, Zhao QL, Ohtsuka K, Tsuneyama K, Kondo T. Identification of genes responsive to paeoniflorin, a heat shock protein-inducing compound, in human leukemia U937 cells. Int J Hyperthermia 2007; 23: 529–537
- Laemmli UK. Cleavage of structural proteins during the assembly of the head of bacteriophage T4. Nature 1970; 227: 680–685
- Towbin H, Staehelin T, Gordon J. Electrophoretic transfer of proteins from polyacrylamide gels to nitrocellulose sheets: Procedure and some applications. Proc Nat Acad Sci USA 1979; 76: 4350–4354
- Tabuchi Y, Takasaki I, Doi T, Ishii Y, Sakai H, Kondo T. Genetic networks responsive to sodium butyrate in colonic epithelial cells. FEBS Lett 2006; 580: 3035–3041
- Tabuchi Y, Takasaki I, Kondo T. Identification of genetic networks involved in the cell injury accompanying endoplasmic reticulum stress induced by bisphenol A in testicular Sertoli cells. Biochem Biophys Res Commun 2006; 345: 1044–1050
- Yan D, Saito K, Ohmi Y, Fujie N, Ohtsuka K. Paeoniflorin, a novel heat shock protein-inducing compound. Cell Stress Chaperones 2004; 9: 378–389
- Sarge KD, Murphy SP, Morimoto RI. Activation of heat shock gene transcription by heat shock factor 1 involves oligomerization, acquisition of DNA-binding activity, and nuclear localization and can occur in the absence of stress. Mol Cell Biol 1993; 13: 1392–1407
- Jia L, Srinivasula SM, Liu FT, Newland AC, Fernandes-Alnemri T, Alnemri ES, Kelsey SM. Apaf-1 protein deficiency confers resistance to cytochrome c-dependent apoptosis in human leukemic cells. Blood 2001; 98: 414–421
- Le XF, Marcelli M, McWatters A, Nan B, Mills GB, O'Brian CA, Bast RC, Jr. Heregulin-induced apoptosis is mediated by down-regulation of Bcl-2 and activation of caspase-7 and is potentiated by impairment of protein kinase C alpha activity. Oncogene 2001; 20: 8258–8269
- Junn E, Han SH, Im JY, Yang Y, Cho EW, Um HD, Kim DK, Lee KW, Han PL, Rhee SG, et al. Vitamin D3 up-regulated protein 1 mediates oxidative stress via suppressing the thioredoxin function. J Immunol 2000; 164: 6287–6295
- Ke B, Shen XD, Zhai Y, Gao F, Busuttil RW, Volk HD, Kupiec-Weglinski JW. Heme oxygenase 1 mediates the immunomodulatory and antiapoptotic effects of interleukin 13 gene therapy in vivo and in vitro. Hum Gene Ther 2002; 13: 1845–1857
- Bruneel A, Labas V, Mailloux A, Sharma S, Royer N, Vinh J, Pernet P, Vaubourdolle M, Baudin B. Proteomics of human umbilical vein endothelial cells applied to etoposide-induced apoptosis. Proteomics 2005; 5: 3876–3884
- Evans MJ, Lai K, Shaw LJ, Harnish DC, Chadwick CC. Estrogen receptor alpha inhibits IL-1beta induction of gene expression in the mouse liver. Endocrinology 2002; 143: 2559–2570
- Cardozo AK, Heimberg H, Heremans Y, Leeman R, Kutlu B, Kruhøffer M, Ørntoft T, Eizirik DL. A comprehensive analysis of cytokine-induced and nuclear factor-kappa B-dependent genes in primary rat pancreatic beta-cells. J Biol Chem 2001; 276: 48879–48886
- Hernández MP, Sullivan WP, Toft DO. The assembly and intermolecular properties of the hsp70-Hop-hsp90 molecular chaperone complex. J Biol Chem 2002; 277: 38294–38304
- Gooljarsingh LT, Fernandes C, Yan K, Zhang H, Grooms M, Johanson K, Sinnamon RH, Kirkpatrick RB, Kerrigan J, Lewis T, et al. A biochemical rationale for the anticancer effects of Hsp90 inhibitors: Slow, tight binding inhibition by geldanamycin and its analogues. Proc Natl Acad Sci USA 2006; 103: 7625–7630
- Suzu S, Tanaka-Douzono M, Nomaguchi K, Yamada M, Hayasawa H, Kimura F, Motoyoshi K. p56(dok-2) as a cytokine-inducible inhibitor of cell proliferation and signal transduction. EMBO J 2000; 19: 5114–5122
- Grandori C, Cowley SM, James LP, Eisenman RN. The Myc/Max/Mad network and the transcriptional control of cell behavior. Annu Rev Cell Dev Biol 2000; 16: 653–699
- Menssen A, Hermeking H. Characterization of the c-MYC-regulated transcriptome by SAGE: identification and analysis of c-MYC target genes. Proc Natl Acad Sci USA 2002; 99: 6274–6279
- O'Donnell KA, Yu D, Zeller KI, Kim JW, Racke F, Thomas-Tikhonenko A, Dang CV. Activation of transferrin receptor 1 by c-Myc enhances cellular proliferation and tumorigenesis. Mol Cell Biol 2006; 26: 2373–2386