Abstract
Hyperthermia, the procedure of raising the temperature of tumor-loaded tissue to 40°–43°C, has been applied to various established cancer treatments. Although the mechanism of hyperthermia in cancer treatment is well-known, there are few or no studies regarding the effect of hyperthermia on the tumor-supportive stroma. Mesenchymal stem cells (MSCs) display the potential for differentiation into various tissues. MSCs are also reported to play a role as potential precursors for tumor stroma in providing a favorable environment for tumor progression. Here, we investigated the effects of hyperthermia-treated MSCs on the viability and growth of cancer cells. Culture supernatants from non-shocked or heat-shocked MSCs (NS-MSCs or HS-MSCs) were added to MCF7 cells. Morphological analysis and cell proliferation assay showed the reduced viability and growth of MCF7 cells by addition of culture medium conditioned by HS-MSCs. Additionally, exposure to the conditioned medium by HS-MSCs induced cell cycle arrest at G2/M phase, increased MHC class I, Fas receptor, and TNF-R expressions, and decreased MDR1 expression in the MCF7 cells. In particular, the conditioned medium of HS-MSCs accelerated the inhibition of tumor cell growth by several chemotherapeutic drugs. These data present new aspects of hyperthermia in cancer treatment, suggesting that hyperthermia can enable tumor stroma provide a sensitizing environment for tumor cells to undergo cell death.
Introduction
Hyperthermia is a therapeutic procedure that raises the temperature of tumor-loaded tissue Citation[1],Citation[2]. Cancer cells are vulnerable to hyperthermia, particularly because of their high acidity caused by the inability to properly expel waste created by anaerobic metabolism Citation[3],Citation[4]. Hyperthermia can attack acidic cells by disrupting the stability of cellular proteins Citation[5] or act as an anti-angiogenic strategy by suppressing tumor-derived vascular endothelial growth factor (VEGF) production, thereby inhibiting endothelial cell proliferation and extracellular matrix remodeling in blood vessels Citation[6]. Such therapy has been used effectively for breast cancer, malignant melanoma, head and neck lymph node (LN) metastasis, glioblastoma, and cervical cancer Citation[7],Citation[8],Citation[9]. The effectiveness of hyperthermia can be strengthened by combination with radiotherapy and chemotherapy Citation[10]. In addition, hyperthermia can promote immunologic host defenses in tumor tissue Citation[11].
Mesenchymal stem cells (MSCs), a subset of non-hematopoietic cells in the marrow stroma, display a potential for self-renewal, proliferation and differentiation into precursors for bone, cartilage, connective and muscular tissue Citation[12]. MSCs isolated from bone marrow (BM) can be characterized mostly by their surface proteins Citation[12],Citation[13]. Nevertheless, specific markers for identifi-cation or evaluation of MSCs have not been clearly defined until now, and studies related to this issue are still needed Citation[14].
Interestingly, there is accumulating evidence that MSCs contribute to tumor stroma which play a fundamental role in tumor growth, invasion and dissemination by interacting with carcinoma cells Citation[15],Citation[16]. During tumor progression, stromagenesis occurs in parallel with tumorigenesis and predisposes to the development of tumor metastasis by generating new vessels Citation[15],Citation[17],Citation[18]. As MSCs have been shown to migrate to the tumor site and potentially become tumor stroma Citation[19], several lines of studies showed that MSCs may favor tumor propagation or dissemination either by promoting invasiveness of tumor cells or by preventing them from recognition by the immune system Citation[20–24]. Specifically, a very recent study reported that MSCs within tumor stroma promote breast cancer metastasis Citation[17]. These studies implicated the potential relationship between MSCs and tumor cells in the tumor microenvironment Citation[25],Citation[26].
Although the effects of hyperthermia on cancer cells are well documented, the influence of hyperthermia on the tumor stromal tissue or cells that interact with cancer cells has not been studied. Here, we evaluated the effects of the culture medium conditioned by heat-shocked MSCs (HS-MSCs) on the viability and growth of MCF7 (human breast adenocarcinoma cell line) cells which involved the physiological and molecular alterations. Thereby, we provide the evidence that hyperthermia is associated with the production of soluble factors secreted from mesenchymal tumor stromal cells that sensitize the tumor cells to undergo cell death, which presents a new aspect of hyperthermia in cancer treatment.
Materials and methods
Preparation and culture of human MSCs
Human MSCs were obtained by aspiration from bone marrow of healthy donors who gave consent after full information at the Hospital of Korea University. Bone marrow cells were carefully overlaid on Ficoll solution (Gibco, CA, USA) and centrifuged to separate the monocytic layer. MSCs were cultured in Dulbecco's Modified Eagle's Medium (DMEM) (Gibco) with 10% fetal bovine serum (FBS) (Gibco) and 1% 10,000 unit penicillin and streptomycin (Gibco) at 37°C in a CO2 incubator. MSCs after 3–4 passages were utilized throughout this study.
Heat treatment of MSCs and conditioned culture condition of MCF7 cells
MSCs were plated at 5 × 105 cells/150 mm2 culture dish and cultured in 25 ml of the culture media. When the confluency reached to 80% (normally about 1.5 ∼ 2 × 105 cells/dish), the MSCs were heat-shocked at 43°C for 45 min, followed by incubation for 24 h at 37°C. Then the culture supernatants were filtered through a 0.2 µm pore filter (Sartorius) and stored at −20°C until use, or immediately transferred to MCF7 cells, which were subsequently cultured for 24, 48 and 72 h. The MSC conditioned medium was regenerated when needed.
Immunophenotyping by flow cytometry
Cells were resuspended in flow cytometry buffer (FCB; 0.1% BSA in PBS) and incubated in FCB containing phycoerythrin (PE)-conjugated anti-CD45, −44, −90, −34 and −105 antibodies. For detection of MHC class I molecules and FAS expression, the purified anti-MHC class I and anti-FAS antibodies and PE-conjugated secondary antibodies (BD Pharmingen) were used. The images were analyzed by FACS Calibur (Beckton-Dickinson [BD]).
Cell proliferation assay
Wst-1 reagent (Roche Diagnostics) was mixed with media at 1:10 ratio, and then added to MCF7 cells. After 1 h, the optical density was measured by ELISA reader at 450 nm. For the combination therapy, 20 µM of 5-aza-cytidine (in acetic acid plus water), 5 µg/ml of 5-FU (in dimethyl sulfoxide [DMSO]), 100 µM of cisplatin (in sodium chloride), or 40 µM of genistein (in DMSO) were used. Statistical analysis was performed using the Student's t-test of Sigmaplot 2001 and P-values of less than 0.05 were regarded as statistically significant.
Analysis of cell cycle
Cells were fixed with ice-cold 70% ethanol for 4 h. The cells were then washed with phosphate-buffered saline (PBS), and incubated with PBS containing 20 µl of RNase A for 30 min at 37°C. After incubation, the cells were stained with 500 µl of 1 mg/ml Propidium Iodide staining solution (BD Pharmingen). The result was analyzed by FACS Calibur (BD).
RT-PCR
RNA was extracted from the cells using the Trizol (Invitrogen, CA, USA) method. Complementary DNA was synthesized from 3 µg of total RNA using MMLV reverse transcriptase (Promega). The cDNA obtained was used as a template for subsequent PCR amplification using Taq polymerase premix (Takara) with 35 cycles of 1 min at 90°C, 1 min at 55°C and 1 min at 72°C. The primers used in this study are followed: VEGF 5′-tcttgggtgcattggagcctc-3′ (sense), 5′-agctcatctctcctatgtgc-3′ (anti-sense); TGF-β 5′-gacatcaacgggttcactaccgg-3′ (sense), 5′-gaggcagaagttggcatggtagc-3′ (anti-sense); MDR1 5′-acaggagataggctggtttg-3′ (sense), 5′-gttgccattgactgaaagaa-3′ (anti-sense); TNF-R 5′- tcagtcccgtgcccagttccacctt-3′ (sense), 5′-ctgaagggggttggggatggggtc-3′ (anti-sense); β-actin 5′-gtcctctcccaagtccacac-3′ (sense), 5′-gggagaccaaaagccttcat-3′ (anti-sense).
Results
Inhibition of tumor cell growth by conditioned medium of heat-treated MSCs
MSC cultured over 3–4 passages were heat-treated as described in materials and methods. Compared to non-shocked MSCs (NS-MSCs), no noticeable changes on cellular morphology or expressions of several surface proteins were observed in heat-shocked MSCs (HS-MSCs) ( and ).
Figure 1. Microscopic analysis of untreated and heat-treated MSCs. MSCs were untreated (no shock) or were heat-treated at 43°C for 45 min, followed by incubation at 37°C for 24 h (heat shock). Microscopic observation was performed by phase-contrast microscopy with 10 (left) or 40 (right) fold of magnification. A representative image is displayed from three independent experiments.
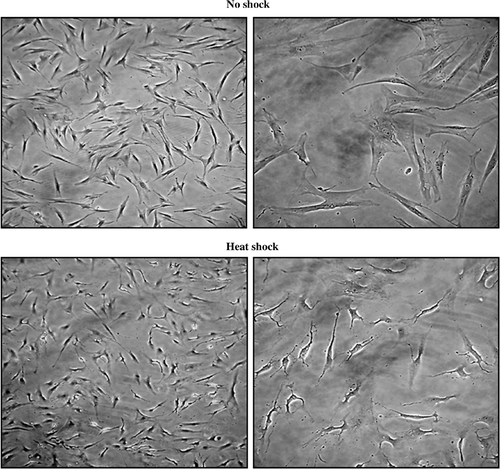
To determine whether tumor stroma given local hyperthermia influences on tumor cells, MCF7 tumor cells were cultured for 24, 48 or 72 h in the medium conditioned by NS-MSCs or HS-MSCs. As observed by phase-contrast microscopy (), the increased detachment of MCF7 cells from substrate was detected after cultured with supernatants of HS-MSCs compared to those cultured with NS-MSCs. Along with this observation, the cell proliferation assay showed the prominent inhibition (maximally about 2 fold) of the growths of MCF7 tumor cells by the conditioned culture medium from HS-MSCs while the conditioned medium from NS-MSCs mildly suppressed the MCF7 cell proliferation (). The same settings of the experiment using another tumor cell line MDA-MB-231 also demonstrated the strong growth-inhibitory effect of the conditioned medium from HS-MSCs compared to the conditioned medium from NS-MSCs (). To examine whether the reduced tumor cell viability could be obtained by different types of stromal cells, primary fibroblast cells were utilized in the identical manner as the MSCs. The result showed no distinguishable differences in the growths of the tumor cells cultured by the conditioned medium from non-shocked or heat-shocked fibroblasts (). These data suggest that the soluble factors secreted from MSCs may exert growth-inhibitory effects on tumor cells, and hyperthermia may facilitate their production and/or secretion from MSCs.
Figure 2. Immunophenotypes of normal and heat-treated MSCs. PE-conjugated anti-CD45, -CD44, -CD90, -CD34, or -CD105 antibodies were reacted with MSCs that were untreated (A, no shock) or incubated for 24 h after heat shock (B, heat shock). The result was obtained by analysis using flow cytometry. A representative image is displayed from three independent experiments.
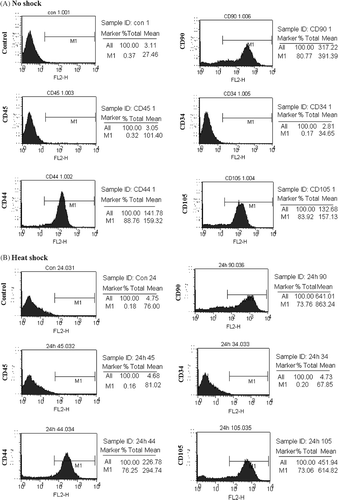
Cell cycle arrest by conditioned medium of heat-treated MSCs
As the proliferation of MCF7 cells was shown to be inhibited by conditioned medium of HS-MSCs (), we further evaluated the cell cycle status of MCF7 cells. After 24, 48, or 72 h incubation with each conditioned medium, MCF7 cells were stained with propidium iodide which can bind intracellular DNA. As shown in , in control MCF7 cells which were incubated with the conditioned medium of NS-MSCs, 56.95%, 49.15%, and 62.79% of cells were retained in G0/G1 phase while 16.57%, 27.14%, and 14.44% of cells were in G2/M phase after 24, 48, and 72 h, respectively. In comparison with the control cells, the percentage of cells in G2/M transition was increased to 24.03%, 40.42% and 30.36% after incubation with conditioned medium of HS-MSCs for 24, 48, and 72 h, respectively. These data imply that the medium conditioned by HS-MSCs exerted suppressive effects on tumorigenesis or growth of tumor cells by cell cycle arrest.
Figure 3. Proliferation of MCF7 cells cultured in the conditioned medium obtained from non-treated or heat-treated MSCs. MCF7 were incubated in the conditioned medium obtained from MSCs that were non-shocked (no shock) or heat-shocked (heat shock). (A) Cellular morphology was observed by phase-contrast microscopy after incubation for the indicated time (24, 48 or 72 h). Bars; 50 µm. (B) Cell proliferation was measured with Wst-1 reagent by reading the optical density at 450 nm after incubation for the indicated time (24, 48 or 72 h). The experiments were performed three times and demonstrated similar results. Statistical significance: *. P < 0.05.
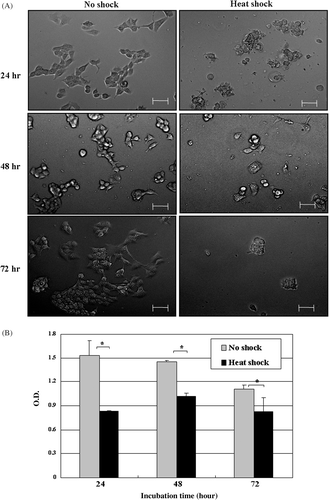
Changes in the expressions of tumor-associated molecules
Based on the above data, we hypothesized that the medium conditioned by HS-MSCs could sensitize tumor cells to undergo cell death. In this aspect, we considered two different possibilities. One possibility was that HS-MSCs could increase the expression of anti-tumorigenic proteins in tumor cells and render the tumor cells susceptible to combined treatment with other cancer therapy like immuno- or chemotherapy. The other possibility was that HS-MSCs could decrease the expression of pro-tumorigenic factors in tumor cells.
Figure 4. Cell cycle analysis of MCF7 cells which were cultured under the conditioned medium from no-shocked or heat-shocked MSCs. MCF7 cells were incubated in the conditioned medium obtained from MSCs that were no-shocked (No shock) or heat-shocked (Heat shock). After incubation for 24, 48 or 72 h, the cells were stained with propidium iodide solution. The image obtained by analysis using flow cytometry was displayed with the percentage at each phase of cell cycle. Similar data were obtained from two independent experiments.
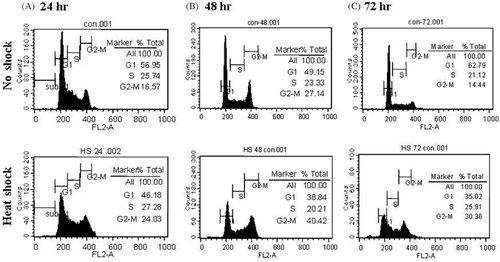
To test the possibilities, first we immunostained the tumor cells cultured in each condition with antibodies against MHC class I molecules or FAS (). As MHC class I molecules are the targets recognized by tumor-specific cytolytic T cells, their expressions are critical for therapeutic outcome of immunotherapy such as cancer vaccine Citation[27]. FAS, one of TNF-R family, are important for transmitting exogenous death signals caused by chemotherapeutic drugs into the cells Citation[28]. Therefore, we examined the expressions of those molecules on MCF7 cells cultured under each condition. There were 1.2 and 1.35 fold increases in the surface expressions of MHC class I molecules and FAS on MCF7 cells, respectively.
Figure 5. Analysis of molecular changes of MCF7 cells which were cultured in the conditioned medium from non-shocked or heat-shocked MSCs. MCF7 cells were incubated in the conditioned medium obtained from MSCs that were non-shocked (no shock) or heat-shocked (heat shock). (A) Flow cytometric analysis of surface proteins. MCF7 cells cultured for 48 h were stained either with anti-MHC class I molecules or anti-FAS antibodies, and subsequent PE-conjugated secondary antibodies. The mean fluorescence intensity was obtained by flow cytometry and converged into the graph. One representative result is displayed from two independent experiments. (B) Analysis of mRNA expressions for tumor-associated molecules by RT-PCR. MCF7 cells cultured in each condition for 24, 48 or 72 h were collected to extract RNA which was subsequently reverse-transcribed to cDNA. PCR was performed with each pair of the indicated primers and β-actin as a control. The image was obtained using gel documentation system after electrophoresis and staining the gel with ethidium bromide. Similar data were obtained from two independent experiments.
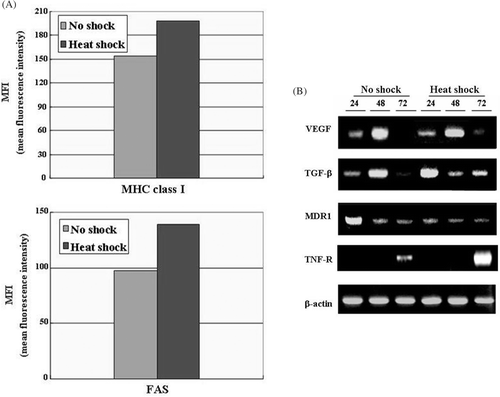
Secondly, mRNA expressions of several pro-tumorigenic factors such as TGF-β, VEGF, MDR1 and pro-apoptotic molecule TNF-R in MCF7 cells cultured under each condition were determined by RT-PCR (). TGF-β and VEGF are soluble proteins which mediate tumor invasiveness and immune suppression Citation[29]. MDR1 (Multi-drug resistance protein) is a membrane protein that provides resistance to various drugs by facilitating excretion of chemotherapeutic molecules out of the cells Citation[30]. TNF-R is a plasma membrane protein which delivers exogenous cell death signals into the cells Citation[31]. The result showed that mRNA level of MDR1 was greatly decreased and mRNA level of TNF-R was markedly increased in MCF7 cells after incubation with the conditioned medium of HS-MSCs (). These results suggest that local hyperthermia on tumor stroma can provide the anti-tumorigenic microenvironment which render tumor cells effectively mediate exogenous cell death signals into the cells and overcome the drug resistance.
A synergistic anti-cancer effect by combination therapy with anti-cancer drugs
Data shown in suggests that HS-MSCs could elevate the effectiveness of chemotherapeutic drug therapy. To investigate potential synergistic effects with chemotherapy, we treated MCF7 cells in each conditioned medium with several chemotherapeutic drugs. Because HS-MSCs caused G2/M phase arrest as shown in , we selected DNA-associated chemotherapeutic drugs (cisplatin, 5-aza-cytidine, 5-FU, genistein) (). In all cases, treatment of the chemotherapeutic drugs with media or the conditioned medium of NS-MSCs exerted little effect on the viability of MCF7 cells. However, the combined treatment of the chemotherapeutic drugs with the conditioned medium of HS-MSCs resulted in the highly efficient inhibitory effects on the tumor cell growth (). This result indicates that hyperthermia on tumor stroma can lead to increased clinical outcome of cancer chemotherapy by overcoming the limited cancer chemotherapy.
Figure 6. Combined effects of the conditioned medium from non-shocked or heat-shocked MSCs with chemotherapeutic drugs. MCF7 cells were cultured in the conditions containing each indicated drug (5-aza-cytidine, 5-FU, cisplatin, genistein) either with media only or the conditioned medium obtained from MSCs that were non-shocked (no shock) or heat-shocked (heat shock). After 48 h incubation, the cell proliferation was assayed with Wst-1 reagent, and the optical density at 450 nm was displayed. The experiments were performed three times and demonstrated similar results. Statistical significance: **. P < 0.01.
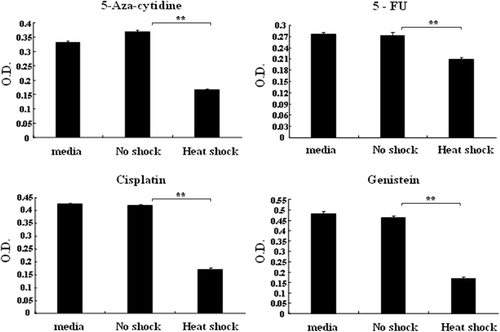
Discussion
MSCs have ‘tropism migration’ toward wound, injury and inflammation by various paracrine and endocrine signals. Interestingly, MSCs can migrate to tumor sites and can create tumor stroma Citation[16]. A study demonstrated that the exposure to tumor cell-conditioned medium promoted migration of MSCs with the increased SDF-1 protein production Citation[32]. Tumor stroma play a supporting role in tumor propagation or dissemination by producing pro-tumorigenic cytokines Citation[33]. Importantly, a recent study demonstrated that MSCs within tumor stroma promote breast cancer metastasis by CCL5 signaling through the chemokine receptor CCR5 expressed in tumors Citation[17]. Therefore, ideas of how to treat tumor stroma are needed to accomplish the more practical efficiency in cancer treatment. In this aspect, our study provides the importance of dealing with tumor stroma, and presents the greater potential of hyperthermia on tumor environment in which stromal cells and cancer cells are interacted.
Considering the interaction between MSCs and tumor cells, we cultured MCF7 cells with the MSC conditioned medium and observed how the tumor cells could be affected by soluble factors produced from the stromal stem cells. As hyperthermia on tumor cells switched the immunosuppressive property of tumor cells toward immunogenic status Citation[34], we expected that hyperthermia on MSCs may be able to provide immunogenic or anti-tumorigenic environments which can result in quiescence of tumor propagation or dissemination. In this study, the culture medium conditioned by HS-MSCs induced the cell cycle arrest, inhibited the cell growth, decreased the expression of pro-tumorigenetic proteins and increased the expression of pro-apoptotic proteins. All of these data indicated that the pro-tumorigenic environment can be switched to anti-tumorigenic nature by local hyperthermia.
showed that the cell cycle of MCF7 cells was mostly blocked in G2/M phase by incubation with the conditioned medium of HS-MSCs. G2/M phase arrest can occur when cell division is not ready due to incomplete DNA replication Citation[35]. Therefore, our data from the cell cycle analysis implied that heat shock induced the enrichment and the secretion of strong DNA damaging factors in MSCs. This implication was tested by investigating the combined effects of the conditioned medium of HS-MSCs with DNA-related chemotherapeutic drugs such as cisplatin, 5-FU, 5-aza-cytidine and genistein. Although genistein is well-known as an antioxidant, it can also induce DNA damage by affecting the activity of DNA topoisomerase Citation[36]. As expected, the combined treatment exerted the synergistic effects on inhibition of growth in MCF7 cells as shown in .
The result obtained from RT-PCR analysis in provided a clue about why hyperthermia can be synergistically effective in combination of chemotherapy. After repeated chemotherapy, tumor cells often become resistant to chemotherapeutic drugs because of increased expression of transporters involved in the drug resistance such as MDR1 Citation[37]. Considering the fact that tumor cells could not be completely cured without destroying tumor stroma which supports relapse tumor cells Citation[38], this study showed that additional local hyperthermia at the tumor site may be very helpful for complete cancer therapy.
The fact that MHC class I molecules were increased after incubation with the conditioned medium of HS-MSCs indicated that the effects of cancer-specific immunotherapy could be highly improved by combination with hyperthermia on tumor stroma. Although cancer-specific cytolytic T lymphocytes (CTLs) are generated by cancer vaccines, tumor cells tend to escape the immune surveillance by down-regulating their surface expression of MHC class I molecules, which render themselves not to be detected by CTLs Citation[39]. Our upcoming study of gene expression profiling analysis showed that MSCs after heat shock expressed higher levels of CIITA: MHC class II transactivator Citation[40] and CD80 Citation[41] which are both immunostimulatory molecules, and IL-16, a lymphocyte-attracting cytokine Citation[42]. Also, hyperthermia on MSCs induced the expression of TNF-α which triggers cell apoptosis via TNF-R Citation[43]. Collectively, when hyperthermia was given on tumor-loaded tissue, tumor stromal cells may express CIITA and CD80, making themselves immunostimulatory, and secrete IL-16 and TNF-α, making the local area inflammatory. Additionally, IL-16 helps recruit effective immune cells which can attack and kill tumor cells and later become memory immune cells against the tumor cells. Locally released TNF-α can present apoptotic signals to tumor cells which highly express TNF-R on their surface as shown in . Accordingly, the tumor microenvironment may become highly immunogenic, making cancer cells more sensitive to attack by immune cells which are recruited to tumor sites and result in negative effects on tumor cell growth.
Taken together, we can expect that heat treatment on MSCs may exert ‘two advantages at one move’ which can support the collapse of tumor cells by transduction of apoptotic signals as well as the prevention of tumor relapse by generation of tumor-specific effector and memory immune cells. Therefore, this study presents the important evidence that hyperthermia on MSCs within tumor stroma can be beneficial for cancer immunotherapy. Even though our present study could not clearly decide the exact mechanism elicited by HS-MSCs on suppression of tumor cells, the issue about the soluble factors from heat-treated MSCs is now under investigation.
Although MSCs are considered, and can be modified by introduction of the candidate genes, as a vehicle of therapeutic Citation[16], this study showed that epigenetic modification targeting MSCs within tumor stroma can be another reasonable approach for cancer therapy.
Acknowledgements
Ho Park and Jung-Ah Cho contributed equally to this work. This study was supported by the NRL (National Research Lab) program, the Korea Science and Engineering Foundation (KOSEF), Republic of Korea (No. R0A-2007-000-20086-0).
Declaration of interest: The authors report no conflicts of interest. The authors alone are responsible for the content and writing of the paper.
References
- Wust P, Hildebrandt B, Sreenivasa G, Rau B, Gellermann J, Riess H, Felix R, Schlag PM. Hyperthermia in combined treatment of cancer. Lancet Oncol 2002; 3: 487–497
- von Ardenne M, Kruger W. Combined whole-body and local hyperthermia for cancer treatment: CMT selectotherm technique. Prog Clin Biol Res 1982; 107: 705–713
- Overgaard J, Bichel P. The influence of hypoxia and acidity on the hyperthermic response of malignant cells in vitro. Radiology 1977; 123: 511–514
- Kang MS, Song CW, Levitt SH. Role of vascular function in response of tumors in vivo to hyperthermia. Cancer Res 1980; 40: 1130–1135
- Ohtsubo T, Igawa H, Saito T, Matsumoto H, Park HJ, Song CW, Kano E, Saito H. Acidic environment modifies heat- or radiation-induced apoptosis in human maxillary cancer cells. Int J Radiat Oncol Biol Phys 2001; 49: 1391–1398
- Sawaji Y, Sato T, Takeuchi A, Hirata M, Ito A. Anti-angiogenic action of hyperthermia by suppressing gene expression and production of tumour-derived vascular endothelial growth factor in vivo and in vitro. Br J Cancer 2002; 86: 1597–1603
- Murata T, Akagi K, Imamura M, Nasu R, Kimura H, Nagata K, Tanaka K. Studies on hyperthermia combined with arterial therapeutic blockade for treatment of tumors: (Part III) Effectiveness of hyperthermia combined with arterial chemoembolization using degradable starch microspheres on advanced liver cancer. Oncol Rep 1998; 5: 709–712
- Ben-Yosef R, Vigler N, Inbar M, Vexler A. Hyperthermia combined with radiation therapy in the treatment of local recurrent breast cancer. Isr Med Assoc 2004; J6: 392–395
- Falk MH, Issels RD. Hyperthermia in oncology. Int J Hyperthermia 2001; 17: 1–18
- Hegewisch-Becker S, Hossfeld DK. [Addition of hyperthermia. Heat potentiates cancer therapy]. MMW Fortschr Med 2001; 143: 28–32
- Roberts NJ, Jr. Impact of temperature elevation on immunologic defenses. Rev Infect Dis 1991; 13: 462–472
- Conget PA, Minguell JJ. Phenotypical and functional properties of human bone marrow mesenchymal progenitor cells. J Cell Physiol 1999; 181: 67–73
- Mageed AS, Pietryga DW, DeHeer DH, West RA. Isolation of large numbers of mesenchymal stem cells from the washings of bone marrow collection bags: Characterization of fresh mesenchymal stem cells. Transplantation 2007; 83: 1019–1026
- Anokhina EB, Burakova LB. [Heterogeneity of stromal precursor cells isolated from rat bone marrow]. Tsitologiia 2007; 49: 40–47
- Fierro FA, Sierralta WD, Epunan MJ, Minguell JJ. Marrow-derived mesenchymal stem cells: Role in epithelial tumor cell determination. Clin Exp Metastasis 2004; 21: 313–319
- Studeny M, Marini FC, Dembinski JL, Zompetta C, Cabreira-Hansen M, Bekele BM, Champlin RE, Andreeff M. Mesenchymal stem cells: Potential precursors for tumor stroma and targeted-delivery vehicles for anticancer agents. J Natl Cancer Inst 2004; 96: 1593–1603
- Karnoub AE, Dash AB, Vo AP, Sullivan A, Brooks MW, Bell GW, Richardson AL, Polyak K, Tubo R, Weinberg RA. Mesenchymal stem cells within tumour stroma promote breast cancer metastasis. Nature 2007; 449: 557–563
- Hung SC, Deng WP, Yang WK, Liu RS, Lee CC, Su TC, Lin RJ, Yang DM, Chang CW, Chen WH, et al. Mesenchymal stem cell targeting of microscopic tumors and tumor stroma development monitored by noninvasive in vivo positron emission tomography imaging. Clin Cancer Res 2005; 11: 7749–7756
- Schichor C, Birnbaum T, Etminan N, Schnell O, Grau S, Miebach S, Aboody K, Padovan C, Straube A, Tonn JC, et al. Vascular endothelial growth factor A contributes to glioma-induced migration of human marrow stromal cells (hMSC). Exp Neurol 2006; 199: 301–310
- Detmar M, Velasco P, Richard L, Claffey KP, Streit M, Riccardi L, Skobe M, Brown LF. Expression of vascular endothelial growth factor induces an invasive phenotype in human squamous cell carcinomas. Am J Pathol 2000; 156: 159–167
- Alberti C. Prostate cancer progression and surrounding microenvironment. Int J Biol Markers 2006; 21: 88–95
- Kopfstein L, Christofori G. Metastasis: Cell-autonomous mechanisms versus contributions by the tumor microenvironment. Cell Mol Life Sci 2006; 63: 449–668
- Aggarwal S, Pittenger MF. Human mesenchymal stem cells modulate allogeneic immune cell responses. Blood 2005; 105: 1815–1822
- Zhu W, Xu W, Jiang R, Qian H, Chen M, Hu J, Cao W, Han C, Chen Y. Mesenchymal stem cells derived from bone marrow favor tumor cell growth in vivo. Exp Mol Pathol 2006; 80: 267–274
- Hombauer H, Minguell JJ. Selective interactions between epithelial tumour cells and bone marrow mesenchymal stem cells. Br J Cancer 2000; 82: 1290–1296
- Hall B, Andreeff M, Marini F. The participation of mesenchymal stem cells in tumor stroma formation and their application as targeted-gene delivery vehicles. Handb Exp Pharmacol 2007; 180: 263–283
- Knutson KL, Disis ML. Tumor antigen-specific T helper cells in cancer immunity and immunotherapy. Cancer Immunol Immunother 2005; 54: 721–728
- Marsters SA, Pitti RA, Sheridan JP, Ashkenazi A. Control of apoptosis signaling by Apo2 ligand. Recent Prog Horm Res 1999; 54: 225–234
- Gaspar NJ, Li L, Kapoun AM, Medicherla S, Reddy M, Li G, O'Young G, Quon D, Henson M, Damm DL, et al. Inhibition of transforming growth factor beta signaling reduces pancreatic adenocarcinoma growth and invasiveness. Mol Pharmacol 2007; 72: 152–161
- Jiang EZ, Chang YJ, Lee JW, Lee WK, Kim JS, Sohn SK, Lee KB, Suh JS. Multi-drug resistance (MDR1) gene expression in de novo acute leukemia cells: Correlations with CD surface markers and treatment outcome. J Korean Med Sci 1998; 13: 617–622
- Kitson J, Raven T, Jiang YP, Goeddel DV, Giles KM, Pun KT, Grinham CJ, Brown R, Farrow SN. A death-domain-containing receptor that mediates apoptosis. Nature 1996; 384: 372–375
- Menon LG, Picinich S, Koneru R, Gao H, Lin SY, Koneru M, Mayer-Kuckuk P, Glod J, Banerjee D. Differential gene expression associated with migration of mesenchymal stem cells to conditioned medium from tumor cells or bone marrow cells. Stem Cells 2007; 25: 520–528
- Rowley DR. What might a stromal response mean to prostate cancer progression?. Cancer Metastasis Rev 1998; 17: 411–419
- Baronzio G, Gramaglia A, Fiorentini G. Hyperthermia and immunity. A brief overview. In vivo 2006; 20: 689–695
- Brown EJ, Baltimore D. Essential and dispensable roles of ATR in cell cycle arrest and genome maintenance. Genes Dev 2003; 17: 615–628
- Salti GI, Grewal S, Mehta RR, Das Gupta TK, Boddie AW, Jr, Constantinou AI. Genistein induces apoptosis and topoisomerase II-mediated DNA breakage in colon cancer cells. Eur J Cancer 2000; 36: 796–802
- Hille S, Rein DT, Riffelmann M, Neumann R, Sartorius J, Pfutzner A, Kurbacher CM, Schondorf T, Breidenbach M. Anticancer drugs induce mdr1 gene expression in recurrent ovarian cancer. Anticancer Drugs| 2006; 17: 1041–1044
- Vincent T, Mechti N. Extracellular matrix in bone marrow can mediate drug resistance in myeloma. Leuk Lymphoma 2005; 46: 803–811
- Bubenik J. Tumour MHC class I downregulation and immunotherapy (Review). Oncol Rep 2003; 10: 2005–2008
- Marten A, Ziske C, Schottker B, Weineck S, Renoth S, Buttgereit P, Schakowski F, Konig S, von Rucker A, Allera A, et al. Transfection of dendritic cells (DCs) with the CIITA gene: Increase in immunostimulatory activity of DCs. Cancer Gene Ther 2001; 8: 211–219
- Chaperot L, Jacob MC, Molens JP, Manches O, Bensa JC, Plumas J. From the study of tumor cell immunogenicity to the generation of antitumor cytotoxic cells in non-Hodgkin's lymphomas. Leuk Lymphoma 2000; 38: 247–263
- Rumsaeng V, Cruikshank WW, Foster B, Prussin C, Kirshenbaum AS, Davis TA, Kornfeld H, Center DM, Metcalfe DD. Human mast cells produce the CD4+ T lymphocyte chemoattractant factor, IL-16. J Immunol 1997; 159: 2904–2910
- Song HL, Lu S, Liu P. [Tumor necrosis factor alpha and enterocyte apoptosis in mice with fulminant hepatic failure.]. Zhonghua Gan Zang Bing Za Zhi 2005; 13: 290–293
Supplementary Figure 1 Proliferation of MDA-MB-231 cells cultured in the conditioned medium obtained from non-treated or heat-treated MSCs. MDA-MB-231 were incubated in the conditioned medium obtained from MSCs that were non-shocked (no shock) or heat-shocked (heat shock). Cell proliferation was measured with Wst-1 reagent by reading the optical density at 450 nm after incubation for the indicated time (24, 48 or 72 h). The experiments were performed three times and demonstrated similar results. Statistical significance: **. P < 0.01.
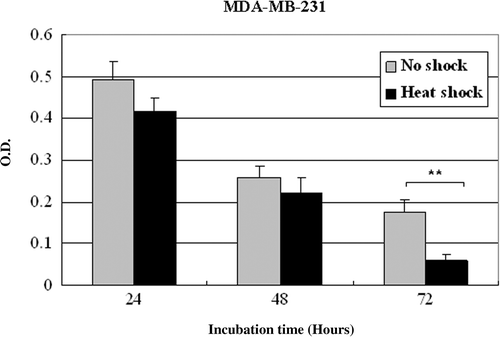
Supplementary Figure 2 Proliferation of MCF7 and MDA-MB-231 cells cultured in the conditioned medium obtained from non-treated or heat-treated fibroblasts. MCF7 or MDA-MB-231 were incubated in the conditioned medium obtained from primary fibroblast cells that were non-shocked (no shock) or heat-shocked (heat shock). Cell proliferation was measured with Wst-1 reagent by reading the optical density at 450 nm after incubation for the indicated time (24, 48 or 72 h). The experiments were performed three times and demonstrated similar results.
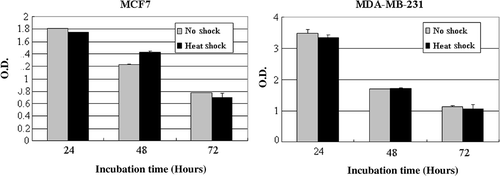