Abstract
The dependence of the thermal enhancement ratio after a sequential action of heat and ionizing radiation on the dose and dose rate of ionizing radiation as well as on the temperature and duration of its application was studied for yeast cells. The combined effect of heat and ionizing radiation on cell killing depended on both the sequence of application (i.e. whether heat is applied prior to or following irradiation) and the temperature. The effectiveness of treatment with heat and ionizing radiation was greatly dependent on the duration of heat exposure. For an equal amount of cell killing from heat alone, long action of heat (50°C) was more effective for radiosensitization than a short acute action of high heat (58°C). For heating at 50°C, heating after irradiation produced more radiosensitization than heating before irradiation. However, high heating at 58°C before irradiation gave the same radiosensitization as heating after irradiation. These data confirm similar observations for mammalian cells. The results were interpreted by means of a mathematical model in which the synergistic effect of the sequential application of heat and ionizing radiation results from the additional lethal damage arising from the interaction of sublesions induced by both agents. These sublesions are not lethal after the action of these modalities, each taken alone. The model appears to be appropriate and the conclusions are valid.
Introduction
It is a well known fact that the synergistic interaction of hyperthermia with ionizing radiation and other agents is widely used in hyperthermic oncology Citation[1]. It has long been considered that the mechanism of interaction of hyperthermia and ionizing radiation may be brought about by an inhibition of the repair from sublethal and potentially lethal damage at a cellular level Citation[2–5]. However, recently it has been shown for heat combined with either ionizing radiation Citation[6] or UV light Citation[7] that the recovery constant defining the probability of recovery was independent of thermal load, while the portion of irreversibly damaged cells gradually increased as a function of heat treatment duration after a sequential treatment of these agents and as a function of the exposure temperature after simultaneous action of these agents. It was concluded on this basis that the synergistic interaction of ionizing or UV radiations and hyperthermia is not related to the impairment of cell recovery capacity itself and that the observed decrease in the rate and the extent of recovery after combined action of these modalities may be attributed to the increased yield of irreversible damage. A simple mathematical model of the synergistic interaction of two agents acting simultaneously has been proposed Citation[13]. The model was shown to provide a description of observed dose rate and temperature dependences after the simultaneous action of hyperthermia with ionizing radiation Citation[8], UV light radiation Citation[9], ultrasound Citation[10] and some chemical compounds Citation[11]. However, the model has not been applied to predict the degree of interaction of heat and ionizing radiation after their sequential action. Extension of the model to the sequential treatment of heat and ionizing radiation seems to be of interest for theoretical and practical reasons. Experimental data obtained with diploid yeast cells demonstrated the significance of the exposure rate in the synergistic interaction of heat simultaneously combined with ionizing or ultraviolet radiation Citation[12]. At the same time the influence of the dose rate of ionizing radiation to the effectiveness of heat and ionizing radiation interaction has been imperfectly understood Citation[13] for their sequential treatment. Thus, the purposes of the present work can be formulated as follows: (1) to study experimentally the effectiveness of interaction of heat and ionizing radiation after their sequential treatment; (2) to suggest the simplest mathematical model which would be able to account for the results obtained and the currently available experimental information.
Materials and methods
In the experiments described here yeast cells were adopted as a model system of eukaryotic cells, not only to offer a practical advantage but also because it is possible to maintain them over a long period in a certain phase of cell cycle. Moreover, the simpler the biological system studied, the fewer complicated biological factors are involved. The wild-type diploid yeast Saccharomyces cerevisiae, strain XS800, was used in this study. Yeast cells were incubated for 5 days at 30°C on a complete nutrient agar layer before they were irradiated. Homogeneous cell populations were treated in the stationary phase of growth. Final suspensions contained 106 cells per ml.
Two 60Co γ-ray sources were used in this study: Gammacell 220 (Atomic Energy of Canada Ltd) and Issledovatel (Radon, Russia). The γ-ray dose rates, estimated by ferrous sulphate dosimetry and by a Siemens calibrated ionization chamber, were 2 and 80 Gy/min.
Hyperthermia (50° and 58°C) was administered in a water bath in which the desired temperature ±0.1°C was maintained. At the end of the treatment, the samples were rapidly cooled to room temperature. The time interval between heating and irradiation was 0.5–1.0 min.
After the treatments, a known number of cells were plated so that 150–200 colonies per dish would be formed by the surviving yeast cells after 5–7 days of incubation at 30°C. Each point was calculated from the colony numbers of triplicate dishes. All experimental series were repeated 3–5 times, and the mean survival values were calculated from at least three independent experiments. Error bars are standard deviations of the means. The exponential parts of survival curves were constructed from data points using the method of least squares; details are described elsewhere Citation[6],Citation[12].
Results
shows the survival curves for yeast cells irradiated with graded doses of γ-rays (2 Gy/min) applied alone or combined with heat exposure (50°C). Cells were subjected to ionizing radiation before or after heat exposure. reveals some conclusions. Postheating and preheating cells markedly increased cell sensitivity to ionizing radiation. The amount of radiosensitization was quantitatively estimated by the thermal enhancement ratio (TER), calculated as the ratio of slopes. The degree of radiosensitization tends to increase with an increasing heat exposure. shows the dependence of the TER on the duration of heat exposure applied after (open circle) or before irradiation (closed circles). The increase in the TER values shows a progressive increase in radiation sensitivity to increasing duration of heat exposure. It is also evident that in both cases there is a value of heat exposure duration (about 2–3 hours) after which no further increase in the cell radiosensitivity can be observed. Note that the sequence of the combination is very important. Ionizing radiation applied before heating was more effective than radiation after heating, i.e. a maximum of TER of 3.0 versus 1.7.
Figure 1. Survival curves for diploid yeast cells of Saccharomyces cerevisiae, strain XS800. Cells were exposed to a sequential treatment with ionizing radiation (60Co γ-ray, 2 Gy/min) and hyperthermia (50°C) and the reverse order of these agents. Different lines are labelled with the duration of heat exposure (hours).
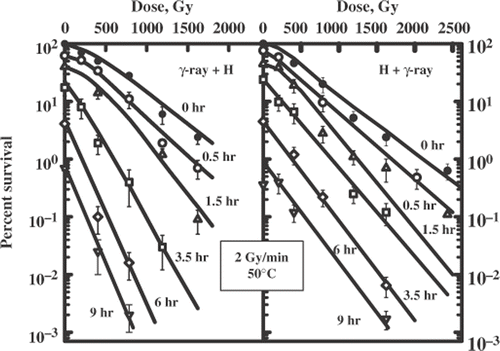
shows the survival curves for the yeast cells irradiated with graded doses of γ-rays delivered at high dose rate (80 Gy/min) and applied alone or combined with the heat exposure (50°C). Here again, hyperthermia after irradiation was much more effective than hyperthermia before irradiation. also includes these data showing the dependence of the TER on the duration of heat exposure applied after or before irradiation. Again, the maximum TER was 3.0 for heating after irradiation versus 1.7 for heating before irradiation. Also, shows the TER was the same for 2 Gy/min and 80 Gy/min.
Figure 2. The dependence of the thermal enhancement ratio on the duration of heat exposure (50°C) after the sequential action of ionizing radiation (60Co γ-rays) and hyperthermia on diploid yeast cells.
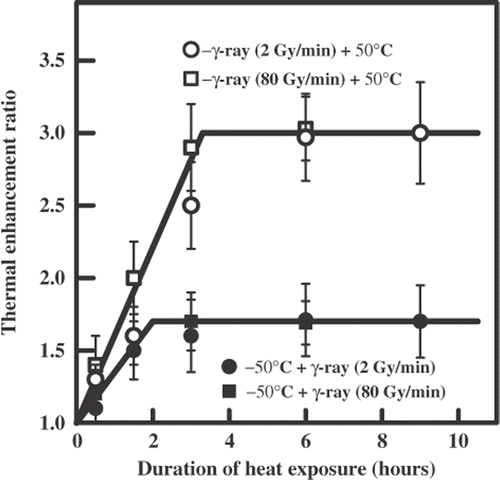
Figure 3. Survival curves for diploid yeast cells exposed to a sequential treatment with ionizing radiation (60Co γ-ray, 80 Gy/min) and hyperthermia (50°C) and the reverse order of these agents. Different lines are labelled with the duration of heat exposure (hours).
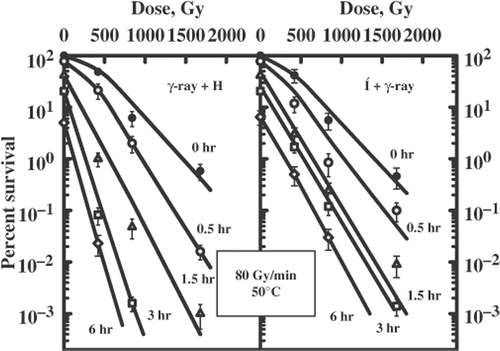
exhibits a set of survival curves for yeast cells irradiated with γ-rays (2 Gy/min) alone or combined with heat exposure (58°C). It is evident that cells treated for 0.5 and 1.0 min after ionizing radiation induced a small amount of radioresistance. These results suggest that a certain level of cytotoxicity from hyperthermia alone is required to achieve a radioenhanced cell killing after combination of ionizing radiation at 2.0 Gy/min and acute hyperthermia (58°C). The amount of the induced radiosensitization and radioresistance was estimated by TER.
Figure 4. Survival curves for diploid yeast cells exposed to a sequential treatment with ionizing radiation (60Co γ-ray, 2 Gy/min) and hyperthermia (58°C) and the reverse order of these agents. Different lines are labelled with the duration of heat exposure (minutes).
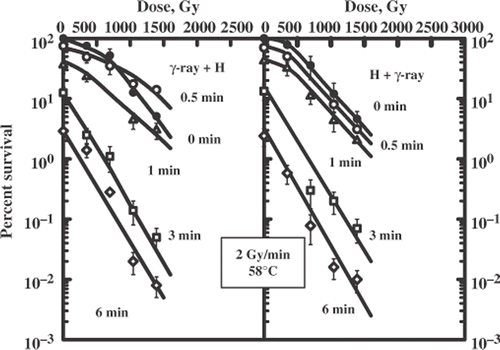
shows the dependence of TER on the duration of heat exposure. The limit values of the TER were 1.5 independently of the sequence of combination. shows the survival curves for the yeast cells irradiated with γ-rays (80 Gy/min) applied alone or combined with heat exposure (58°C). also includes these data. Here again, the limit values of TER were identical for treatment with γ-ray + hyperthermia and the reverse order of treatment but their values were larger (TER = 1.9) than for low dose rate (TER = 1.5). The sequence of the combination was important for the rate of TER increase which was greater for the sequence 58°C + γ-ray (80 Gy/min). Note that the combination of ionizing radiation either at a low or high dose rate with high temperature was never as effective as that for the greatest effect (TER = 3.0) obtained at either dose rate of radiation followed by a low temperature.
Mathematical model and its validation
An understanding of the reason and magnitude of the interactive effects obtained in this study is important both for a practical and theoretical point of view. As was demonstrated before Citation[6], the synergistic interaction of ionizing radiation and hyperthermia is not related to an impairment of the recovery capacity itself and that the observed decrease in the rate and the extent of recovery after combined action of these modalities may be attributed to the increased yield of the irreversible damage. We suggest that the synergistic interaction of ionizing radiation and hyperthermia might be expected to result from the additional lethal damage arising from the interaction of sublesions induced by both agents. Let N1 and N2 be the yield of some hypothetical lethal damage produced by ionizing radiation and hyperthermia, respectively. It might be reasonable to assume that some additional effective damage, responsible for the interaction of these agents, has arisen during the sequential action of both modalities. We hypothesize that the additional damage may be formed due to the interaction of sublesions induced by both agents. These sublesions are thought to be ineffective when each agent is applied separately. Let p1 and p2 be the mean numbers of the sublesions that arise for one effective damage induced by ionizing radiation and heat, respectively. Then the number of additional lethal damages (N3) responsible for a thermal radiosensitization may be given by
According to this equation, the amount of additional damage is determined by the minimal value of the sublesions produced by ionizing radiation (p1N1) and hyperthermia (p2N2). This equation has two parameters (p1 and p2) to be estimated from the experimental data. The whole number of lethal damage (NΣ) after a combined action will be determined by
The effectiveness of cell radiosensitivity by hyperthermia was characterized in this study by the value of TER which was defined as the ratio of slope of the survival curves obtained after combined action to the slope after ionizing radiation applied alone. Inasmuch as the slope is determined by the amount of effective damage, we can write
Combining equations 1 and 3, one can deduceIt follows from equation 4 that if p1N1 > p2N2 we have
Equation 5 shows that the value of TER should increase with an increase in N2, i.e. the duration of heat exposure, until the inequality p1N1 > p2N2 holds. This prediction is in accordance with the data obtained in this study ( and ). Equation 5 also shows that the increase in TER growth should be greater for increased values of p2 parameter. It follows from equation 4 that if p1N1 < p2N2, we have
i.e. the value of TER is a constant value from the moment when the inequality p1N1 < p2N2 occurs, as was observed in this study ( and ) at the point when the plateau in TER is reached, i.e.
This equation shows that this point corresponds to an equal number of sublesions produced by radiation and heat.
The basic parameters of the model (p1 and p2) can be estimated in the following way. If the inequality p1N1 < p2N2 is correct, we have from equation 2 thatOn the contrary, if the inequality p1N1 > p2N2 holds, we have from equation 2 that
From target theory based on a Poisson distribution of random lethal events Citation[14],Citation[15], cell survival (S) is determined from the number of lethal events, N, by the expressionwhere N can be any number of the lethal events in equations 1–9. It enables the determination of the basic model parameters p1 (equation 8) and p2 (equation 9) and allows for the prediction of the TER (equation 5) until the inequality p1N1 > p2N2 holds, i.e. the highest value of the TER (equation 6) and the condition (equation 7) at which it becomes apparent.
Thus, this model would permit the interpretation of biological data in terms of the numbers of hypothetical sublesions responsible for the synergistic interaction of heat and ionizing radiation. This method was applied to the experimental data presented above. includes the estimated basic model parameters and the greatest TER after different conditions of the sequential action of ionizing radiation and hyperthermia obtained in the experiments and predicted by the model described. A comparison between the theoretically predicted highest values of TER and the experimentally determined values shows a good correspondence in all cases.
Table I. The dependence of the basic model parameters (p1, p2) and the greatest TER after different conditions of the sequential action of ionizing radiation (γ-rays) and hyperthermia (H).
Discussion
The present studies provide information for yeast cells on the dependence of the thermal enhancement ratio after the sequential action of heat and ionizing radiation on the dose and dose rate of ionizing radiation as well as on the temperature and duration of heating. The results obtained were described and interpreted by means of a mathematical model in accordance with which the synergistic interaction of heat and ionizing radiation is expected to result from the additional lethal damage arising from the interaction of the sublesions induced by both agents. In accordance with the definition, these sublesions are not lethal after the action of these modalities, each taken alone. The idea of sublesion interaction is widely used in theoretical conceptions Citation[16–18]. Since the magnitude of synergistic effect is directly dependent on the number of sublesions available, temporal effect such as a sublesion repair would contribute to a change in the effectiveness of interaction; therefore, a simultaneous exposure should be more effective than sequential one Citation[18], as was shown for mammalian Citation[19] and yeast cells Citation[8],Citation[11]. The decrease of synergy, when the effect of one agent predominates, simply reflects a small number of sublesions produced by other agent.
In the model described here, the synergistic interaction is given by min {p1 N1 ;p2 N2}. This means that one sublesion from ionizing radiation interacts with one sublesion caused by heat. This process is assumed to proceed until the very last sublesion of the less frequent type is used up. To estimate the basic parameters p1 and p2 we have used the real experimental results, and the model does not consider the molecular nature of sublesions or the mechanism of their interaction, which is beyond the main aim of the study.
The major point to be inferred from the experimental data presented is that the extent of thermal radiosensitization was the greatest when the prolonged heat exposure (50°C) was applied after irradiation than when applied before irradiation. The effectiveness of thermal radiosensitization at high dose rate was the same (TER = 3) as at a forty-fold lower dose rate. These observations suggest a longer preservation of sublesions produced by ionizing radiation than sublesions produced by hyperthermia. These observations suggest the elimination and/or repairing of thermal sublesions during the protracted heat exposure, and that similar elimination of thermal sublesions was not observed when the cells were exposed to ionizing radiation followed by a prolonged heat exposure, since the produced thermal sublesions could immediately interact with the long-lived sublesions produced by ionizing radiation.
The existence of the plateau in the curve describing the TER dependence on the duration of heat exposure ( and ) may be brought about by an exhaustion of the ionizing radiation sublesions. It is worth noting that several studies have shown that there was an upper limit to the synergistic interaction of different agents acting sequentially Citation[1],Citation[19–21].
Acute heat exposure (58°C) with ionizing radiation delivered at 2 Gy/min or 80 Gy/min resulted in a decrease in the highest values of TER (1.5–1.9) for various sequences of heat and ionizing radiation. The decrease of this value in comparison with the highest values (TER = 3.0) obtained for post-heating at 50°C would be a reflection of fewer heat sublesions produced for thermal damage at 58°C (). Hyperthermia at 58°C could produce a greater number of severe thermal lesions, but a smaller number of sublesions. The p1 parameter was greater when ionizing radiation at any dose rate was followed by a protracted action of heat (50°C) in comparison with the heat at 58°C (1.9 versus 0.5–0.8). The most likely explanation for these results is that the decrease in both the TER and p1 after the acute thermal exposure (58°C) might be attributed to a limitation of time needed for an interaction of the sublesions. A moderate decrease in the highest value TER for the acute heat action (58°C) and the low dose rate compared with the high dose rate (1.9 versus 1.5) may be partly related to an antagonistic interaction of these agents clearly observed after small duration of heat (). This effect known as a thermal induction of radiation resistance was described in literature Citation[22],Citation[23] and is beyond the main aim of this study.
It is worth noting that our data for yeast cells support studies with mammalian cells Citation[20],Citation[21]. Specifically for high temperature, where there is more killing from heat alone than for lower temperature where there is less killing from heat alone, cell killing for heat combined with ionizing radiation is independent of the order of treatment, i.e. heat before irradiation gives the same killing as heat after irradiation. Our data make it clear that for the lower temperature the sequence γ-ray + heat gives more cell killing than the inverse order of treatment, with the amount of cell killing for heat after exposure to ionizing radiation being greater for 50°C than for 58°C. Similar inferences were made for mammalian cells Citation[20],Citation[21]. It was concluded in these papers that when the lethal effect from hyperthermia alone is minimal, lethality from combined action of ionizing radiation and thermal treatment is greater when hyperthermia follows irradiation than when hyperthermia precedes irradiation. It indicates the general responses of yeast and mammalian cells to the combined action of heat and ionizing radiation.
Overall, we can conclude that in spite of the approximations used in the simplified model considered, the experimental results seem to be in reasonable agreement with the model predictions. The results of this study demonstrate the possibility of a reconcilable explanation and prediction of experimental data on the sequential action of heat and ionizing radiation with yeast cells. One can expect the applicability of the model to predict the effectiveness of interaction of different agents applied sequentially to living cells of various origin.
Acknowledgements
This work was supported in part by Russian Funds of Fundamental Researches, Humanity Sciences and Administration of Kaluga Region (grant numbers 07-04-96424 and 08-06-59609), the Brain Pool Program and the National R&D Program by the Ministry of Education, Science and Technology (MEST) of Korea.
Declaration of interest: The authors report no conflicts of interest. The authors alone are responsible for the content and writing of the paper.
References
- Streffer C, Vaupel P, Hahn G. Biological Basis of Oncologic Thermotherapy. Springer, Berlin, Heidelberg, New York, London, Paris, Tokyo, Hong Kong 1990
- Ben-Hur E, Elkind MM, Bronk BV. Thermally enhanced radioresponse of cultured Chinese hamster cells: Inhibition of repair of sublethal damage and enhancement of lethal damage. Radiat Res 1974; 58: 38–51
- Gerweck LE, Gillette EL, Dewey WC. Effect of heat and radiation on synchronous Chinese hamster cells: Killing and repair. Radiat Res 1975; 64: 611–623
- Li GC, Evans RG, Hahn GM. modification and inhibition of repair of potentially lethal X-ray damage by hyperthermia. Radiat Res 1976; 67: 491–501
- Raaphorst GP, Azzam EI, Feeley M. Potentially lethal radiation damage repair and its inhibition by hyperthermia in normal hamster cells, mouse cells, and transformed mouse cells. Radiat Res 1988; 113: 171–182
- Petin VG, Kim JK. Survival and recovery of yeast cells after combined treatment with ionizing radiation and heat. Radiat Res 2004; 161: 56–63
- Kim JK, Petin VG, Tkhabisimova MD. Survival and recovery of yeast cells after simultaneous treatment of UV light radiation and heat. Photochem Photobiol 2004; 79: 349–355
- Petin VG, Komarov VP. Mathematical description of synergistic interaction of hyperthermia and ionizing radiation. Mathem Biosci 1997; 146: 115–130
- Petin VG, Kim JK, Zhurakovskaya GP, Rassokhina AV. Mathematical description of synergistic interaction of UV light and hyperthermia for yeast cells. J Photochem Photobiol B:Biol 2000; 55: 74–79
- Petin VG, Zhurakovskaya GP, Komarova LN. Mathematical description of combined action of ultrasound and hyperthermia on yeast cells. Ultrasonics 1999; 37: 79–83
- Petin VG, Zhurakovskaya GP, Kim JK. Synergistic effects of different pollutants and equidosimetry. Equidosimetry – Ecological Standardization and Equidosimetry for Radioecology and Environmental Ecology, F Brechignac, G Desmet. Springer, Dordrecht 2005; 207–222
- Petin VG, Kim JK, Zhurakovskaya GP, Dergacheva IP. Some general regularities of synergistic interaction of hyperthermia with various physical and chemical agents. Int J Hyperthermia 2002; 18: 40–49
- Little JB. Low-dose radiation effects: Interaction and synergism. Health Phys 1990; 59: 49–55
- Lea DE. Actions of Radiation on Living Cells, 2nd. Cambridge University Press, London and New York 1955
- Haynes RH. The interpretation of microbial inactivation and recovery phenomena. Radiat Res 1966; Suppl 6: 1–29
- Chadwick KH, Leenhouts HP. The Molecular Theory of Radiation Biology. Springer, Berlin, Heidelberg, New York 1981
- Harder D, Virsik-Peuckert RP, Bartels ER. Theory of intratrack pairwise lesion interaction. Radiat Prot Dosimetry 1994; 52: 13–16
- Zaider M, Rossi HH. The synergistic effects of different radiations. Radiat Res 1980; 83: 732–739
- Sapareto SA, Hopwood LE, Dewey WC. Combined effects of X-irradiation and hyperthermia on CHO cells for various temperatures and orders of application. Radiat Res 1978; 73: 221–233
- Martignoni KD, Smith KC. The synergistic action of ultraviolet and X-radiation on mutants of Escherichia coli K-12. Photochem Photobiol 1973; 18: 1–8
- Dewey WC, Hopwood LE, Sapareto SA, Gerweck LE. Cellular responses to combinations of hyperthermia and radiations. Radiology 1977; 123: 463–474
- Mitchell REJ, Morrison DP. Heat-shock induction of ionizing radiation resistance in Saccharomyces cerevisiae, and correlation with stationary growth phase. Radiat Res 1982; 90: 284–291
- Bresler SE, Beketova AG, Noskin LA, Stepanova IM. Temperature-induced radioresistance of Escherichia coli cells. Radiobiology 1984; 24: 579–583, (in Russian)