Abstract
Purpose: Hyperthermia is useful in the treatment of human head and neck cancers, because it is relatively easy to regulate temperatures when compared to tumors located in deep organs. In this study, attention was focused on p53 as a possible predictive indicator for the efficacy of hyperthermic cancer therapy.
Methods: Two kinds of cell lines were used. These were derived from a human squamous cell carcinoma (SAS) and had identical genetic backgrounds except for their p53 gene status. It was previously reported that the heat sensitivity and frequency of apoptosis in wild-type p53 cells (SAS/neo) were clearly elevated when compared with mutated p53 cells (SAS/mp53). In order to study the expression of apoptosis related proteins after heat treatment, protein microarray analysis was used.
Results: The expression of apoptosis inhibitory proteins such as Bcl-2, Bcl-xL, NF-κB, COX2, STAT3, IL-6, and IKKα/1 was seen to increase after heat treatment in SAS/mp53 cells, but not in SAS/neo cells.
Conclusion: The result of these observations indicates that apoptosis inhibitory proteins (such as Bcl-2, Bcl-xL, IL-6, etc.) were highly induced in SAS/mp53 cells after heat treatment when compared to control SAS/neo cells.
Introduction
The tumor suppressor gene p53 is located in chromosome 17p13 and prevents the malignant degeneration of a normal cell by regulating cell-cycle progression, apoptosis and DNA repair. An abnormality in the p53 gene introduces genetic instabilities and raises probabilities for the occurrence of carcinogenesis and teratogenesis Citation[1]. In particular, p53 mutations are seen at a high frequency in most human tumors; such p53 mutations are seen in about half of all malignant tumors in human oral epidermoid cancers Citation[2],Citation[3]. p53 molecules possess multiple functional activities, including activity as a sequence-specific transcription factor. p53 molecules are phosphorylated in response to cell stresses, and this event directly affects downstream genes Citation[4]. When the p53 gene is normal, it has been reported that the p53 protein is activated, not only by genotoxic agents, but also by other types of stresses, such as heat, cold, low pH, or hypoxia Citation[4].
In the present study, attention was focused on the role of p53 as a predictive indicator for the hyperthermia treatment of cancer. Hyperthermia now plays an important role in therapy for human malignant tumors, particularly when used in conjunction with recombinant adenovirus-p53 Citation[5], radiotherapy Citation[6–9] or chemo-radiotherapy Citation[10–14]. Hyperthermia treatment is suitable for human head and neck cancers because it is relatively easy to regulate local temperatures in these locations when compared to deep organs such as the liver and pancreas. It has already been reported that p53 gene status affects heat sensitivity in human cancer cells. In particular, wild-type p53 (wtp53) containing cells are heat sensitive when compared to mutated p53 (mp53) containing cells. This was demonstrated by using two cell lines with identical genetic backgrounds except for their p53 gene status Citation[15]. To date, studies have been reported concerning the expression of apoptosis-related genes after irradiation and hyperthermia treatment using cDNA array analysis Citation[15],Citation[16]. However, there has been no study concerning the details of apoptosis-related protein expression after hyperthermia treatment. Therefore, in order to confirm any p53 association with heat-induced apoptosis, the work described here followed the expression of each apoptosis-related protein after hyperthermia using protein microarray analysis. This analysis was done on tongue squamous cell carcinomas containing normal and mutated versions of the p53 gene.
Antibody-based protein microarrays are a new technology which can measure quantitative differences in polypeptide expression directly by allowing fluorescently labeled protein mixtures from cell extracts to bind onto glass slides spotted with different monoclonal antibodies (mAbs) which are specific for specific human proteins. The goal of this study was to identify sets or groups of proteins which could provide a tool or indicator to monitor, or possibly to predict, thermoresistance associated with p53-dependent signal transduction pathways involved in heat-induced apoptosis.
Materials and methods
Cells
Human squamous cell carcinomas were used; these cell lines are called SAS/neo and SAS/mp53 Citation[17]. SAS/neo cells have a wtp53 phenotype in response to heat-, X-ray- or CDDP-induced signal transduction, although these cells do have a point mutation in codon 336 of exon 10 in the p53 gene Citation[17],Citation[18]. SAS/mp53 cells produce a dominant negative mp53 protein Citation[19]. Prior to experiments, cells were cultured at 37°C in Dulbecco's modified Eagle's medium containing 10% (v/v) fetal bovine serum, penicillin (50 U/ml), streptomycin (50 µg/ml), and kanamycin (50 µg/ml) (DMEM-10).
Heat treatment
Exponentially growing cells were cultured in 75-cm2 flasks containing DMEM-10, and then immersed for 40 min in a water bath (Model EX-B, TAITEC Co. Ltd., Saitama, Japan) at a temperature of 44 ± 0.1°C.
Survival rate
Cell survival was measured using a standard colony forming assay. Three replicate flasks were used and three independent experiments were performed for each survival point. Colonies obtained after two weeks of growth were fixed with methanol and stained with a 2% Giemsa solution. Microscopic colonies composed of more than approximately 50 cells were counted as having grown from surviving cells.
Apoptosis rate
Attached and detached cells were collected by centrifugation and trypsinization at 48 h after heating (44°C, 40 min). Cells were fixed with 1% glutaraldehyde (Nacalai Tesque, Kyoto, Japan) in PBS at 4°C, washed with PBS, stained with 0.2 mM Hoechst 33342 (Sigma Chemical Co., St Louis, MO), and then observed under a fluorescence microscope. Induction of apoptosis was scored by the detection of nuclear and cytoplasmic condensation with blebbing leading to the formation and release of apoptotic bodies.
Protein microarray procedure
The Ab Microarray (BD Clontech, Palo Alto, CA) detects a wide variety of proteins (both cytosolic and membrane bound) which represent a broad range of biological functions, including signal transduction, cell-cycle regulation, gene transcription and apoptosis. The microarray contains 512 highly specific and sensitive mAbs constructed against human polypeptides. The complete list of the arrayed antibodies, including the Swiss-Prot ID numbers of the target antigens, is available elsewhere (http://bioinfo2.clontech.com/abinfo/array-list-action.do).
Protein extraction
Cells were harvested 12 h after heating (44°C, 40 min). Cells were cultured at 37°C in a conventional humidified 5% CO2 incubator following heating. Approximately 50 to 150 mg of material was obtained from subconfluent cells in 75-cm2 flasks. Total protein was extracted with freeze-thaw cycling of the cell pellet in liquid nitrogen followed by homogenization in the manufacturer's extraction/labeling buffer (BD Clontech), which contains non-denaturing detergents to maintain protein solubility and to emulsify membrane-bound proteins. After measurement of the total protein concentration with the bicinchoninic acid method (Pierce, Rockford, IL), each sample was diluted to a final total protein concentration of 1.1 mg/ml.
Fluorescent labeling with green fluorescent (Cy3) and red fluorescent (Cy5) dyes
From each heated and control sample, a 900 µl volume of the total protein preparation was labeled with a 100 µl volume of the labeling dyes, either cyanin 3 (Cy3) or cyanin 5 (Cy5) (Amersham Biosciences, Piscataway, NJ). The dual-fluorescence detection method is designed so that inherent variations in dye labeling do not affect the outcomes of experiments. After a 90 min incubation and 30 min of blocking, the unbound dye was removed using PD-10-desalting columns (Amersham Biosciences). Protein concentrations were determined using the bicinchoninic acid method. The average number of dye molecules covalently coupled to each protein was measured as described in the user manual, and usually ranged from 2 to 4 molecules of Cy3 or Cy5 per molecule of protein.
Clontech microarray slides
These arrays are printed on standard-size (75 × 25 × 1 mm) glass slides with 512 antibodies in duplicate on each slide. Two slides were used so that reverse color labeling could be used on one slide to allow normalization of the samples. Protein samples from SAS/neo or SAS/mp53 cells labeled with Cy5 were mixed with protein samples from heat-treated cells labeled with Cy3 and incubated with slide 1. For slide 2, the protein samples from control cells were labeled with Cy3 and the proteins from heat-treated cells were labeled with Cy5. Labeling the proteins from both the control and heat-treated cells with Cy3 and Cy5 allows the microarray to detect differences in specific protein abundances between the heat-treated sample and the control sample for each experiment and compensates for any potential difference in the binding of specific proteins to the Cy3 or Cy5 dyes. A sample of the total protein (20 µg) from each cell preparation was added to each slide and incubated at room temperature for 30 min before a series of washes. The slides were dried and scanned according to the instructions provided by the supplier using ScanArray Express™ version 2.0 (Perkin Elmer, Whaltham, MA). There were two ratios generated from the spot images: heat-treated-Cy5/control-Cy3 (slide 1) and control-Cy5/heat-treated-Cy3 (slide 2), for each protein array.
Data analysis and clustering
The mean of the ratios of Cy5/Cy3 for each polypeptide on both slides was analyzed using Clontech Array-Specific Analysis workbook software which was developed specifically to be used with each microarray lot by the manufacturer. The two ratios were used to calculate an internally normalized ratio (INR), or ratio of ratios, for each spot on the array. This calculation normalizes for differences due to labeling efficiency and antibody-antigen binding affinity, greatly enhancing the precision and accuracy of the assay. The replicate values within each slide were then averaged, and an INR value was calculated where INR = , and ratios 1 and 2 correspond to values obtained from slides 1 and 2. Ratio 1 equals control-Cy5 relative fluorescent units/heat-treated-Cy3 relative fluorescent units, and Ratio 2 equals heat-treated-Cy5 relative fluorescent units/control-Cy3 relative fluorescent units. The average INR was calculated for each antibody, and duplicate INR values which varied by more than 30% were discarded. INR values that varied by a factor of more than 1.5 or less than 0.8 were considered as valid indicators of changes in protein abundance in this study. These thresholds were determined by taking the global INR value for each experiment (i.e. calculated average INR of all spots in an experiment) and multiplying by 1.5 and 0.8 to get the specific threshold INR values for each subsequent microarray value. Clustering analysis was performed with DNASIS Stat® (Hitachi Software Engineering Co. Ltd., Tokyo, Japan) software using single slide normalization.
Results
The data for surviving fractions and apoptosis after heat treatment in SAS/neo cells and SAS/mp53 cells are shown in Citation[15]. SAS/neo cells were more heat sensitive and had a high frequency of heat-induced apoptosis when compared to SASmp53 cells.
Table I. Survival and apoptosis rates in SAS/neo and SAS/mp53 cells after heating (44°C, 40 min) Citation[15].
The data obtained from the protein array assays were analyzed initially with ScanArray Express™ version 2.0, and then with Excel and DNASIS Stat® to calculate the INR values. The overall protein expression patterns for the two samples were analyzed with hierarchical clustering. This algorithm orders proteins on the horizontal axis of a graph based on the similarity of their expression profiles (). Proteins were analyzed which had at least a 1.5-fold higher increase in expression or at least a 0.8-fold decrease in expression when compared with non-treated cells. Fifty proteins were up-regulated in the heat treated SAS/neo cells, and 359 proteins were up-regulated in the heat treated SAS/mp53 cells. Furthermore, apoptosis-related proteins were analyzed when they were seen to have valid changes in expression, and 7 proteins in the heat-treated SAS/neo cells and 44 proteins in the heat-treated SAS/mp53 cells were observed to be up-regulated. These proteins were analyzed with hierarchical clustering () and the protein expression levels are shown in . In the clusters are shown almost completely as green (i.e. with relatively low score expression changes). The reason for this is that most proteins showing valid changes are distributed primarily in the low signal area, because there was one protein (NF-ATc1: nuclear factor of activated T cells 1) which displayed an exceptionally high INR value of 10.37. To compensate for this effect, shows a larger range of colors in the clustering. This difference was observed from the exclusion of NF-ATcl from the clustering, and from the selection of apoptosis-related proteins which showed valid changes in their clustering.
Figure 1. Unsupervised hierarchical clustering analysis of 512 proteins in heat treated cells compared with non-treated cells. The graph indicates relative protein expression levels. Lower signal values are indicated by green, and higher signal values are indicated by red.
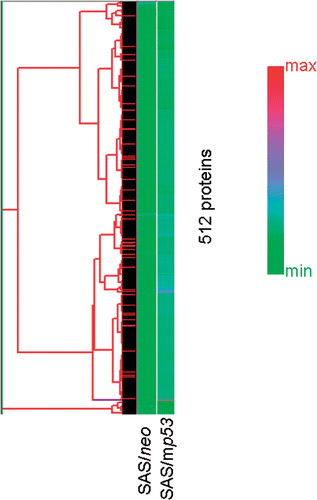
Figure 2. Hierarchical clustering analysis of 44 selected apoptosis-related proteins which showed valid changes in heat treated cells when compared to non-treated cells. The graph indicates protein expression levels with lower signal values shown in green and higher signal values shown in red.
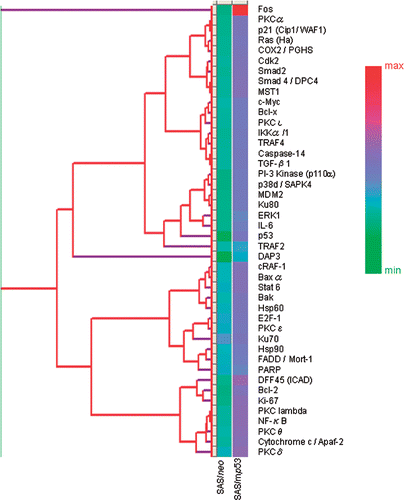
Figure 3. INRs for apoptosis-related proteins which were obtained from protein microarray analysis. The central line is the average value for INRs as measured from the expression of 44 selected apoptosis-related proteins which showed valid changes in response to heat treatment.
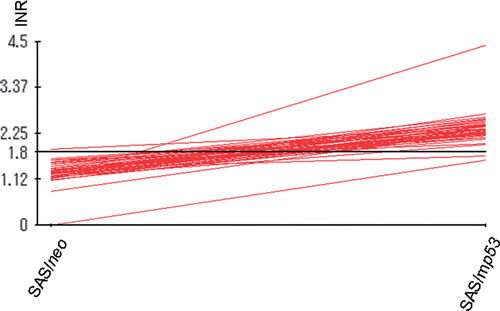
As shown in , among the apoptosis-inductive proteins, FADD (Fas-associating protein with death domain)/Mort-1 (mediator of receptor-induced toxicity-1), Ku70, PKC (Protein kinase C)δ, PKCε and E2F-1 (expression of transcription factor–1) were up-regulated; these proteins are considered to play as apoptosis mediators, in SAS/neo cells.
Table II. Apoptosis-related proteins with more than a 1.5-fold increase in expression in SAS/neo cells.
In , among the proteins which inhibit apoptosis, Bcl-2 (B–cell lymphoma/leukemia-2), COX (cyclooxygenase)-2, PKCα, PKCβ, DFF45 (DNA fragmentation factor-45), PKCθ, Bcl-x (B-cell CLL/lymphoma x the long isoform), MDM2 (murine double minute 2), NF-κB (nuclear factor κB), Rb2 (retinoblastoma protein 2), STAT3 (signal transducer and activator of transcriptional 3), TRAF2 (tumor necrosis factor receptor associated factor 5), IL-6 (interleukin 6), PKCλ, PKCτ and IKK-α/1 (I-κ-B kinase-α/1) were up-regulated in SAS/mp53 cells. FADDα/Mort-1, Ku70, PKCδ, PKCε and E2F-1 were up-regulated in SAS/mp53 cells as well as in SAS/neo cells. Other apoptosis-inducing proteins were also up-regulated in SAS/mp53 cells. These were Baxα (Bcl-2 associated x protein α), cRAF-1 (V-raf-1 marine leukemia vial oncogene homolog 1), TGF-β1(transforming growth factor β1), cytochrome c/Apaf-2 (apoptotic protease activating factor 2), c-Myc (cellular homologue of avian myelocytomatosis virus oncogene), Bak (Bcl-2 homologous antagonist/killer), DAP3 (death-associated protein kinase 3), Smad2 (Sma- and Mad-related protein 2) and Smad4/DPC4 (Sma- and Mad-related protein 4/deleted in pancreatic cancer locus 4). The level of induced Hsp90 (heat shock protein 90) in SAS/mp53 cells was higher than that induced in SAS/neo cells.
Table III. Apoptosis-related proteins with more than a 1.5-fold increase in expression in SAS/mp53 cells.
Discussion
Previous reports Citation[15] have indicated that (1) heat sensitivity in SAS/neo cells was higher than that in SAS/mp53 cells, and (2) heat-induced apoptosis was more frequently observed in SAS/neo cells than SAS/mp53 cells. These results lend support for the existence of a p53-dependent apoptosis process which, in part, regulates the heat sensitivity of SAS cells with different types of p53 gene status. To obtain further insights into p53-dependent heat-induced signal transduction leading to apoptosis, the expression pattern of apoptosis-related proteins was analyzed after exposure to heat using a protein microarray method.
Protein microarray analysis showed that FADD/Mort-1, Ku70, PKCδ, PKCε, E2F-1 and PARP (poly (ADP-ribose) polymerase) expression increased after exposure to heat in both SAS/neo and SAS/mp53 cells. Elsewhere, it has been reported that FADD is induced by heat Citation[20] and that the over-expression of FADD results in the induction of apoptosis Citation[20]. Ku70, PKCδ, PKCε and E2F-1 are known to be involved in apoptosis Citation[21–24]. Based on these reports, it would appear that FADD/Mort-1, Ku70, PKCδ, PKCε, E2F-1 and PARP are involved in heat-induced p53-independent apoptosis in SAS/neo and SAS/mp53 cells. It was observed that apoptosis-inhibitory proteins such as NF-κB, Bcl-2, Bcl-xL, COX2, STAT3 and IL-6 were up-regulated in SAS/mp53 cells after heating. IL-6 has been reported to be down-regulated by heat Citation[25] to an even greater extent in SAS/neo cells Citation[26]. Bcl-2, Bcl-xL and IKKα (an apoptosis-inhibitory protein) are also down-regulated preferentially in SAS/neo cells Citation[27–29]. From these results it could be expected that it would be difficult to induce apoptosis after heating in SAS/mp53 cells when compared to SAS/neo cells. Moreover, it is known that Hsp90 inhibits apoptosis Citation[30], and this corresponds with its observed induction to higher levels in SAS/mp53 cells than in SAS/neo cells ().
The up-regulation of Bcl-2 and MDM2 in SAS/mp53 cells was consistent with a previous study using DNA array analysis Citation[15]. Other comparable results with this previous study were not possible, because the DNA array analysis consisted of observations of the cDNA from only 198 genes, and the type of genes and proteins analyzed differed between the DNA array analysis and the current protein microarray analysis. However, the expression profile of mRNAs does not always agree with the profile for protein expression, and a correlation coefficient of 0.48 has been obtained between mRNA and protein abundances Citation[31].
From these results it appears that apoptosis-inhibitory proteins, such as NF-κB, Bcl-2, Bcl-xL, COX2, STAT3, IL-6 and IKKα, were strongly induced after heat treatment in SAS/mp53 cells when compared to induction in SAS/neo cells. From these observations, a possible heat-induced p53-dependent signal transduction pathway for apoptosis can be proposed, as shown in . Heat shock activates a subset of apoptosis-related proteins. The findings in the present study show that p53 gene status is closely associated with heat-induced apoptosis, and with the expression of apoptosis-inhibitory proteins, such as Bcl-2, Bcl-xL and IL-6. It is assumed that heat-resistance in mp53 cells may result from the up-regulation of these apoptosis-inhibitory proteins. The present study confirms previous suggestions that the gene status of p53 might serve as a good predictive indicator for developing more effective cancer therapies. These results also suggest that heat-induced apoptosis might be suppressed by the increased expression of apoptosis-inhibitory proteins, such as Bcl-2, Bcl-xL and IL-6, in mp53 cells (SAS/mp53) but not in wtp53 cells (SAS/neo). Although there might be a limitation in this experiment because only one set of clones were used, these results provide support for the idea that p53 gene status could serve as a useful candidate for a predictive indicator of the effectiveness of hyperthermic therapy.
Conclusion
The results reported here show that apoptosis-inhibitory proteins (such as Bcl-2, Bcl-xL, IL-6, and others) were strongly induced by heat treatment in SAS/mp53 cells when compared to SAS/neo cells containing a WT p53.
Acknowledgements
This work was supported by Grants-in-Aid from the Ministry of Education, Science, Sports, Culture and Technology of Japan. This work was also funded in part by a grant from the Central Research Institute of the Electric Power Industry of Japan and by a grant for Exploratory Research for Space Utilization from the Japan Space Forum.
Declaration of interest: The authors report no conflicts of interest. The authors alone are responsible for the content and writing of the paper.
References
- Wang B. Involvement of p53-dependent apoptosis in radiation teratogenesis and in the radioadaptive response in the late organogenesis of mice. J Radiat Res 2001; 42: 1–10
- Friend S. p53: A glimpse at the puppet behind the shadow play. Science 1994; 265: 334–335
- Hollstein M, Sidransky D, Vogelstein B, Harris CC. p53 mutations in human cancers. Science 1991; 253: 49–53
- Wang X, Ohnishi T. p53-dependent signal transduction induced by stress. J Radiat Res 1997; 38: 179–194
- Zhang S, Xu G, Liu C, Xian S, Sun Y, Su X, Cai Y, Li D, Xu B. Clinical study of recombinant adenovirus-p53(Adp53) combined with hyperthermia in advanced cancer. A report of 15 cases. Int J Hyperthermia 2005; 21: 631–636
- Hurwitz MD, Kaplan ID, Hansen JL, Prokopios-Davos S, Topulos GP, Wishnow K, Manola J, Bornstein BA, Hynynen K. Hyperthermia combined with radiation in treatment of locally advanced prostate cancer is associated with a favorable toxicity profile. Int J Hyperthermia 2005; 21: 649–656
- Franckena M, Stalpers LJ, Koper PC, Wiggenraad RG, Hoogenraad WJ, van Dijk JD, Wárlám-Rodenhuis CC, Jobsen JJ, van Rhoon GC, van der Zee J. Long-term improvement in treatment outcome after radiotherapy and hyperthermia in locoregionally advanced cervix cancer: An update of the Dutch Deep Hyperthermia. Int J Radiat Oncol Biol Phys 2008; 70: 1176–1182
- Aktas M, de Jong D, Nuyttens JJ, van der Zee J, Wielheesen DH, Batman E, Burger CW, Ansink AC. Concomitant radiotherapy and hyperthermia for primary carcinoma of the vagina: A cohort study. Eur J Obstet Gynecol Reprod Biol 2007; 133: 100–104
- Horsman MR, Overgaard J. Hyperthermia: A potent enhancer of radiotherapy. Clin Oncol 2007; 19: 418–426
- Westermann AM, Jones EL, Schem BC, van der Steen-Banasik EM, Koper P, Mella O, Uitterhoeve AL, de Wit R, van der Velden J, Burger C, et al. First results of triple-modality treatment combining radiotherapy, chemotherapy, and hyperthermia for the treatment of patients with stage IIB, III, and IVA cervical carcinoma. Cancer 2005; 104: 763–770
- Kamisawa T, Tu Y, Ishiwata J, Karasawa K, Matsuda T, Sasaki T, Funata N, Tsuruta K, Okamoto A, Takahashi T. Thermo-chemo-radiotherapy for advanced gallbladder carcinoma. Hepatogastroenterol 2005; 52: 1005–1010
- Kamisawa T, Tu Y, Egawa N, Karasawa K, Matsuda T, Tsuruta K, Okamoto A. Thermo-chemo-radiotherapy for advanced bile duct carcinoma. World J Gastroenterol 2005; 11: 4206–4209
- Xia H, Karesawa K, Hanyu N, Chang TC, Okamoto M, Kiguchi Y, Kawakami M, Itazawa T. Hyperthermia combined with intra-thoracic chemotherapy and radiotherapy for malignant pleural mesothelioma. Int J Hyperthermia 2006; 22: 613–621
- Milani V, Pazos M, Issels RD, Buecklein V, Rahman S, Tschoep K, Schaffer P, Wilkowski R, Duehmke E, Schaffer M. Radiochemotherapy in combination with regional hyperthermia in preirradiated patients with recurrent rectal cancer. Strhlenther Onkol 2008; 184: 163–168
- Yasumoto J, Kirita T, Takahashi A, Ohnishi K, Imai Y, Yuki K, Ohnishi T. Apoptosis-related gene expression after hyperthermia in human tongue squamous cell carcinoma cells harboring wild-type or mutated-type p53. Cancer Lett 2004; 204: 41–51
- Yasumoto J, Imai Y, Takahashi A, Ohnishi K, Yuki K, Kirita T, Ohnishi T. Analysis of apoptosis-related gene expression after X-ray irradiation in human tongue squamous cell carcinoma cells harboring wild-type or mutated p53 gene. J Radiat Res 2003; 44: 41–45
- Ota I, Ohnishi K, Takahashi A, Yane K, Kanata H, Miyahara H, Ohnishi T, Hosoi H. Transfection with mutant p53 gene inhibits heat-induced apoptosis in a head and neck cell line of human squamous cell carcinoma. Int J Radiat Oncol Biol Phys 2000; 47: 495–501
- Jia LQ, Osada M, Ishioka C, Gamo M, Ikawa S, Suzuki T, Shimodaira H, Niitani T, Kudo T, Akiyama M, et al. Screening the p53 status of human cell lines using a yeast functional assay. Mol Carcinog 1997; 19: 243–253
- Kern SE, Pietenpol JA, Thiagalingam S, Seymour A, Kinzler KW, Vogelstein B. Oncogenic forms of p53 inhibit p53-regulated gene expression. Science 1992; 256: 827–830
- Kim PK, Wang Y, Gambotto A, Kim TM, Weller R, Zuckerbraun BS, Hua Y, Watkins SC, Billiar TR. Hepatocyte Fas-associating death domain protein/mediator of receptor-induced toxicity (FADD/MORT1) levels increase in response to pro-apoptosis stimuli. J Biol Chem 2002; 277: 38855–38862
- Sawada M, Sun W, Hayes P, Leskov K, Boothman DA, Matsuyama S. Ku70 suppresses the apoptotic translocation of Bax to mitochondria. Nat Cell Biol 2003; 5: 320–329
- Bharti A, Kraeft SK, Gounder M, Pandey P, Jin S, Yuan ZM, Lees-Miller SP, Weichselbaum R, Weaver D, Chen LB, et al. Inactivation of DNA-dependent protein kinase by protein kinase Cδ: Implications for apoptosis. Mol Cell Biol 1998; 18: 6719–6728
- Chen N, Ma W, Huang C, Dong Z. Translocation of protein kinase Cipsilon and protein kinase Cδ to membrane is required for ultraviolet B-induced activation of mitogen-activated protein kinases and apoptosis. J Biol Chem 1999; 274: 15389–15394
- DeGregori J, Leone G, Miron A, Jakoi L, Nevins JR. Distinct roles for E2F proteins in cell growth control and apoptosis. Proc Natl Acad Sci USA 1997; 94: 7245–7250
- van Malle W, Wielodkx B, Mahieu T, Takada M, Taniguchi T, Sekikawa K, Libert C. HSP70 protects against TNF-induced lethal inflammatory shock. Immunity 2002; 16: 685–695
- Margulies L, Sehgal PB. Modulation of the human interleukin-6 promoter (IL-6) and transcription factor C/EBP beta (NF-IL6) activity by p53 species. J Biol Chem 1993; 268: 15096–15100
- Budhram-Mahadeo V, Morris PJ, Smith MD, Midgley CA, Boxer LM, Latchman DS. p53 suppresses the activation of the Bcl-2 promoter by the Brm-3a POU family transcription factor. J Biol Chem 1999; 274: 15237–15244
- Sugars KL, Budhram-Mahadeo V, Packham G, Latchman DS. A minimal Bcl-x promoter is activated by Brn-3a and repressed by p53. Nucleic Acid Res 2001; 29: 4530–4540
- Gu L, Zhu N, Findley HW, Woods WG, Zhou M. Identification and characterization of the IKKα promoter: Positive and negative regulator by ETS-1 and p53, respectively. J Biol Chem 2004; 279: 52141–52149
- Ohnishi K, Yuki K, Ohnishi T. Hyperthermic cancer therapy combined with inhibitors targeted against heat-induced signaling factors. Jpn J Hyperthermic Oncol 2004; 20: 143–159
- Anderson L, Seilhamer J. A comparison of selected mRNA and protein abundances in human liver. Electrophoresis 1997; 18: 533–537