Abstract
Objective: To study the effect of dextran magnetic fluid hyperthermia on growth, vascular endothelial growth factor (VEGF) expression and apoptosis of the grafted H22 tumors in mice.
Methods: Mice with grafted H22 tumor were randomly divided into four groups. The treatment group was intratumorally injected with dextran magnetic fluid and subsequently exposed to a 55 kHz 20 kA/m alternating magnetic field for 10 min. The three control groups were respectively injected with physiological saline and no magnetic field; with physiological saline and exposed to 55 kHz 20 kA/m alternating magnetic field for 10 min; and with dextran magnetic fluid only. The inhibitive ratios were calculated. Other mice with grafted tumor were randomly divided into two groups, respectively injected with dextran magnetic fluid or physiological saline and exposed to a 55 kHz 20 kA/m alternating magnetic field for 10 min. The VEGF expression and apoptosis in tumor tissues were analyzed at 1 h, 5 h, 24 h, 48 h and 336 h after treatment.
Results: The inhibitive ratio of treated group was 59.74%, 52.51% and 40.35% respectively. Necrotic areas in tumor tissue were mainly distributing around the magnetic fluid and became larger with the lengthening of treatment. The VEGF expression and apoptosis of the treated group did not show obvious difference from that of the control group at different time points.
Conclusion: Single time magnetic fluid hyperthermia significantly inhibited growth of grafted H22 tumor in mice, which mainly resulted from inducing necrosis while not inhibiting VEGF expression or inducing apoptosis.
Introduction
The use of local hyperthermia for tumor therapy can be traced back thousands of years. Today, common heat therapies used clinically include microwave, radiofrequency, laser and ultrasound. Magnetic fluid hyperthermia is based on the principle that magnetic nanoparticles exposed to an alternating magnetic field can be heated to curable temperatures. The feasibility of magnetic fluid hyperthermia in tumor therapy has already been validated by some reports Citation[1–3], but the molecular mechanism and route by which magnetic fluid hyperthermia inhibits tumor growth requires further research. It was previously reported that the home-made water-based dextran-coated magnetic fluid (dextran magnetic fluid) used in vivo was tolerable, safe and biocompatible Citation[4]. In this paper, we observed effects of magnetic fluid hyperthermia on the growth of grafted H22 tumor and the change on vascular endothelial growth factor (VEGF) expression and apoptosis with time after heat treatment. This study enhances the understanding of the mechanism of tumor growth inhibition under magnetic fluid hyperthermia.
Materials and methods
Animal and cell line
BALB/c mice (about 20 g, male, specific-pathogen free) were purchased from the Laboratory Animal Center of Shanghai. The use and management of animals was legally acquired and conducted in compliance with the principles expressed in the Guide for the Care and Use of Laboratory Animals of China. The mouse hepatoma cells (H22) were offered by the Department of Laboratory Animal of Hebei Medical University.
Grafted tumor growth
1 × 107/0.1 ml of H22 cells were subcutaneously injected into the flank of the mice. After four days, the mice were randomly divided into four groups with eight mice in each group. The average volume of tumors was 115.65 ± 30.23 mm3. The mice were anesthetized with pentobarbital sodium before treatment. The treatment group was injected at five different points within the tumor with 0.2 ml of dextran magnetic fluid (including 24.16 mg ferrite particles with an average diameter of about 15 nm Citation[4]. The mice were subsequently exposed to an alternating magnetic field generated from a designed device, once with the maximum magnetic intensity of 55 kHz 20 kA/m for 10 min. The three control groups were respectively: intratumorally injected with 0.2 ml physiological saline; with 0.2 ml physiological saline and exposed to 55 kHz 20 kA/m alternating magnetic field once for 10 min; and with 0.2 ml dextran magnetic fluid. Three mice were chosen randomly from each group to detect real-time intratumoral temperature by fiber-optic detection (FOT-M fiber-optic sensors and FTI-10 signal conditioners, FISO technologies Inc., Canada), while another three were selected to examine the rectal temperature. Mice were sacrificed after two weeks. Tumor mass was weighed to calculate the ratio of inhibition. Ratio of inhibition (%) = (tumor weight of control group − tumor weight of treatment group)/tumor weight of control group × 100.
VEGF expression and apoptosis
Other mice were inoculated as the above and divided into two groups. At the fourth day after inoculation, mice of the treatment group were injected intratumorally with 0.2 ml dextran magnetic fluid and mice of the control group were injected with physiological saline. Both groups were exposed to a 55 kHz 20 kA/m alternating magnetic field for 10 min. Animals of both groups were sacrificed at 0 h, 1 h, 5 h, 24 h, 48 h and 336 h after treatment. Histology, VEGF expression and apoptosis in the tumor tissue were analyzed in three mice at each time point. Tumor tissues injected but not exposed to the magnetic field were used as a 0 h control.
Flow cytometry analysis
Cell suspension was made from fresh tumor tissue by using phosphate buffer saline (PBS) as medium, and centrifuged at low speed (1000 rpm) in order to mostly remove the fragment cause by necrotic cells or operation. Cell concentration was 1 × 106/ml. A 1- ml cell suspension was centrifuged and re-suspended with 0.1 ml PBS, then added with 2 µg (10 µl) rabbit anti-mouse VEGF antibody (Youyi Zhonglian Biotechnology Co. Ltd) for 1 h, washed three times with PBS, added with FITC-labeled goat-anti-rabbit IgG (Sino-American Biotechnology Co.) for 30 min, washed with PBS. Cell filtrate was analyzed using FACS Aria cell cytometry (BD Co., USA, excitation light wave length 488 nm).
Another 1 ml cell suspension was centrifuged and resuspended with 0.1 ml PBS, and then dyed with 5 mg/ml propidium iodide (PI) for 30 min. Cells were washed with PBS and filtrate and analyzed using FACS Aria cell cytometry.
Histological and immunohistochemical detection
Some tumor tissue was fixed with formaldehyde, embedded with paraffin and then sliced into sections. The histological detection was conducted after those sections were stained with hematoxylin-eosin (HE). VEGF expression of tissue sections was detected by the ABC method according to the instructions in the ABC kit user manual (purchased from Huamei Biotechnology Co. Ltd). Rabbit anti-mouse VEGF antibody (purchased from Youyi Zhonglian Biotechnology Co. Ltd) was used as the primary antibody. The biotin-labeled goat-anti-rabbit IgG (Sino-American Biotechnology Co.) was used as the second antibody. PBS instead of anti-VEGF antibody was used as the control. 3,3′-diaminobenzidine (DAB) was used as the substrate. The results were analyzed by image-analysis system (HPIAS-1000 image-analysis system, product of Tongji Medical University, China). Samples with an optical absorbency larger than the control by at least the mean value plus twice the standard deviation (SD) were defined as positive.
In situ apoptosis detection
Slices were examined by terminal deoxynucleotidyl transferase (TdT)-mediated dUTP nick-end labeling (TUNEL) method to show apoptosis in tissue sections. The TUNEL assay kit was purchased from Sino-American Biotechnology Co. and applied according to the instructions in the user manual.
Statistical analysis
Data was reported as the mean ± SD and analyzed with Microsoft Excel. Statistical difference between treatment groups and control groups was determined by a two-tailed t test. Statistical significance was designated as P < 0.05.
Results
Effect on grafted tumor growth
The tumor weight of the treatment group was less than that of the three control groups; the ratios of inhibition were all above 30%, but only the difference between the first control and treated group has statistical significance (P < 0.05). Tumor weights of the grafted tumors are shown in .
Table I. The effect of hyperthermia with dextran magnetic fluid on grafted tumor growth.
Intratumoral temperature and rectal temperature
Treating the tumor with dextran magnetic fluid and then exposing it to an alternating magnetic field resulted in intratumoral temperatures rising to 41°C within 2–3 min. The intratumoral temperatures were able to be kept at or above 41°C until the end of treatment. The intratumoral temperature could reach as high as 48°C in the treatment group, whereas the temperature in the control groups remained fairly unchanged during exposure to the magnetic field. However, rectal temperatures in the treated group were almost the same as those in control groups during the entire treatment. Intratumoral and rectal temperature curves for a representative mouse are shown in .
Histological detection
Intratumoral injection allows the dextran magnetic fluid to infiltrate into the tumor tissue, although not with a uniform distribution. Necrotic areas in tumor tissue became larger with the lengthening of treatment. 48 h after treatment with a magnetic field, the necrotic area in the treated group tumor tissue was larger than that of the control group, and mainly distributed around the magnetic fluid. Representative tissue sections stained by HE are shown in .
Figure 2. The tumor tissues stained by hematoxylin-eosin (HE). (A) 1 h after injecting with physiological saline; (B) 1 h after injecting with magnetic fluid; (C) 1 h after injecting with physiological saline and exposed to magnetic field; (D) 1 h after injecting with magnetic fluid and exposed to magnetic field; (E) 48 h after injecting with physiological saline and exposed to magnetic field; (F) 48 h after injecting with magnetic fluid and exposed to magnetic field. (The black points in B, D and F were the magnetic fluid. The scale bars represent 50 µm. A little necrosis was shown near magnetic fluid in D. Compared with A, B, C, D and E, more nucleus disaggregation and cell broken were shown around magnetic fluid in F. The necrosis in E is near the center of the tissue, however, the necrosis in f is distributed around magnetic fluid.)
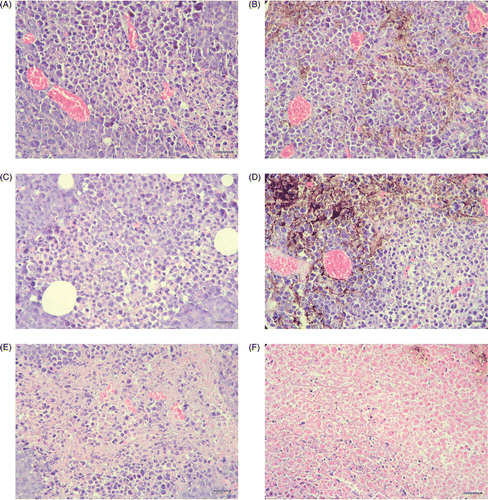
VEGF expression
Through immunohistochemical analysis, VEGF expression in the tumor tissue at different time points post-treatment did not show obvious differences between the treated and control groups. The VEGF expression of tumor tissues at 24 h detected by immunohistochemistry is shown in .
Figure 3. The vascular endothelial growth factor (VEGF) expression of tumor tissues at 24 h after treating with magnetic field stained by immunohistochemical method. (A) injected with physiological saline; (B) injected with magnetic fluid. (The scale bars represent 50 µm. VEGF was distributing in cytoplasm and intercellular substance. The intensity in B seemed higher than in A.)
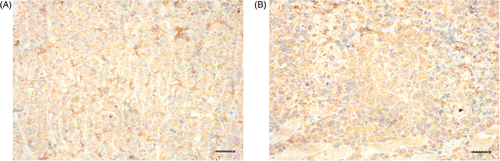
At 0 h, 1 h, 5 h, 24 h, 48 h, and 336 h after treatment, the VEGF expression of tumor tissues was analyzed by flow cytometry analysis. The results are shown in . No statistical significant differences were found between subsequent time points or between treated and control groups at the individual time points.
Apoptosis
Examined by in situ apoptosis detection, the numbers of apoptotic cells at different time-points after treatment were similar. The apoptotic situations of representative tissue sections at 48 h after treatment are shown in .
Figure 5. In situ apoptosis detection of tumor tissues at 48 h after treating with magnetic field. (A) injected with physiological saline; (B) injected with dextran magnetic fluid. The scale bars represent 50 µm.
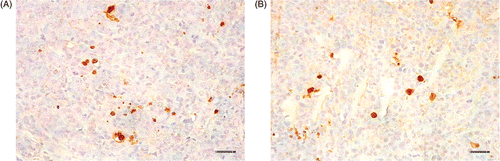
The apoptotic ratio was analyzed by flow cytometry analysis. It was shown that apoptotic cell numbers of the treated and control groups both increased and reached the apogee at 5 h after treatment. However, there was no statistical significance between different time points and different groups. The results of the flow cytometry analysis are shown in .
Discussion
It has been reported that magnetic fluid hyperthermia can be used in tumor therapy. Johannsen et al. reported that the temperature in rat prostate tumors can reach 70°C after having been injected with magnetic fluid and exposed to a magnetic field of 100 kHz 18 kA/m for 30 min. After being exposed to a magnetic field of 12.6 kA/m for 30 min, tumor growth in the treated group was inhibited compared to that of the control group, with an inhibitive ratio of 44–51% Citation[3]. The results in this paper also prove that single magnetic fluid hyperthermia can inhibit the growth of grafted tumors in mice.
Data show that local hyperthermia treatment can induce an immunology reaction Citation[5], tissue necrosis Citation[6] and cell apoptosis Citation[7], increase vessel permeability Citation[8],Citation[9], and inhibit tumor cell infiltration and migration Citation[10]. In short, it has various effects on tumor growth, but the molecular mechanism of local hyperthermia as well as magnetic fluid hyperthermia still remains uncertain. Vertrees et al. treated normal human bronchus epithelium cell BEAS2-B and its corresponding malignant cell BZR-T33 in 43°C for 180 min, during which it could be seen that nucleic acid polymerase degraded, tumor necrosis (TNF)-related apoptosis-inducing ligand (TRAIL) increased by 5–7 times, and percentage of apoptotic malignant cells increased. Compared with normal cells, malignant cells had lower viability and were difficult to cancerogenesis Citation[7]. But Komata et al. reported that to human glioblastoma U251-MG and U87-MG, hyperthermia only caused arrest and autophagy of cells rather than apoptosis Citation[11]. Nakahata et al. dealt with several kinds of normal and tumor cells at 43°C for 2 h, which showed that abnormal centrosomes appeared in tumor cells; with time passing, the number of cells with multiple nucleoli increased. The nuclear morphology of those cells looked like that of apoptotic cells, but on the fourth day after treatment no DNA ladder could be detected and no apoptotic signal appeared in the in-situ apoptosis detection. Those results suggested that the death of those tumor cells was not caused by apoptosis, but rather by the disruption of mitosis Citation[12]. In our experiments, the apoptotic situation of treated and control tissues did not show statistical difference two weeks after magnetic fluid hyperthermia treatment. The apoptotic situation of treated and control tissues at different time points after hyperthermia also did not show any obvious differences. According to these results, apoptosis was not an inevitable reaction to the magnetic fluid hyperthermia treatment. Inducing apoptosis was not the main reason for inhibition of grafted tumor growth following magnetic fluid hyperthermia.
A previous report shows that treatment at 41°C for 90 min made VEGF secretion in endothelial cells decrease Citation[13]. In vivo, hyperthermia increases vessel permeability Citation[8],Citation[9]. Therefore, hyperthermia represses angiogenesis to inhibit grafted tumor growth. But the VEGF expression of tumor tissues was examined at different times after hyperthermia treatment, and there was no significant change between the treated group and matched control group. VEGF is a particular promoter for angiogenesis. These results suggest that anti-angiogenesis was not the reason for hyperthermia-inhibited grafted tumor growth.
Local thermotherapy causes tissue damage, which has two stages: (1) direct injury caused by heating; (2) indirect injury after heating; necrosis of tumor tissues is mainly caused by direct injury Citation[14]. Eikesdal et al. treated rat BT4An tumors at 44°C for 60 min. Compared with the control group, single treatment induced delay of tumor growth; 25–50% of blood vessels in the tumor were destroyed, especially in the center of the tumor Citation[15]. Nikfarjam et al. treated normal liver and colorectal liver metastases by Neodymium-Yttrium-Aluminium Garnet Laser (Nd-YAG) with 2 W 100 J for 50 s. Necrosis, apoptosis, and vascular injury were analyzed at 24 h, 48 h, 72 h, 120 h, and 168 h after treatment. Their results showed that heat treatment induces damage to the microvessels and tissue immediately, and increases apoptosis in the tissue Citation[16]. After heat treatment, vascular and tissue injury still increase gradually, with vascular injury appearing earlier than tissue injury Citation[9], but apoptosis is stable Citation[16], which is consistent with the tendency that the volume of necrosis in tumor tissue increases with time. Consequently, the temporal decrease of VEGF in the experiment might be caused by necrosis of the vessels and tissue. Necrosis is the main reason for magnetic fluid hyperthermia inhibition of grafted tumor growth.
Furthermore, experiments have shown that, in vitro, magnetic particles inhibit proliferation of human fibroblast cells; decrease the survival rate of the cells Citation[17], induce Hep-6 cell apoptosis Citation[18], and cause cell disruption Citation[19]. Although in the experiment, magnetic particles were not seen inside the cell, the difference of tumor growth between the group injected with magnetic fluid and the group injected with saline has no statistical significance, but it could not be entirely denied that magnetic particles or the magnetic field itself could also inhibit tumor growth. Therefore, further lengthy observations are needed to evaluate the effect of magnetic particles on tumor tissues and cells, and further research is needed to reveal the mechanism of magnetic fluid hyperthermia on tumor growth.
Conclusion
Single time magnetic fluid hyperthermia is able to inhibit grafted tumor growth in the H22 mouse model significantly. Induced necrosis, while not inhibiting VEGF expression or inducing apoptosis, is the main reason for magnetic fluid hyperthermia inhibited grafted tumor growth.
Acknowledgements
The work was supported by the 863 Hi-Tech Research and Development Program of China (2002AA302210) and Shanghai NanoProgram in China (0249 nm071).
Declaration of interest: The authors report no conflicts of interest. The authors alone are responsible for the content and writing of the paper.
References
- Johannsen M, Gneveckow U, Thiesen B, Taymoorian K, Cho CH, Waldöfner N, Scholz R, Jordan A, Loening SA, Wust P. Thermotherapy of prostate cancer using magnetic nanoparticles: Feasibility, imaging, and three-dimensional temperature distribution. Eur Urol 2007; 52: 1653–1661
- Maier-Hauff K, Rothe R, Scholz R, Gneveckow U, Wust P, Thiesen B, Feussner A, von Deimling A, Waldoefner N, Felix R, et al. Intracranial thermotherapy using magnetic nanoparticles combined with external beam radiotherapy: Results of a feasibility study on patients with glioblastoma multiforme. J Neurooncol 2007; 81: 53–60
- Johannsen M, Thiesen B, Jordan A, Taymoorian K, Gneveckow U, Waldofner N, Scholz R, Koch M, Lein M, Jung K, et al. Magnetic fluid hyperthermia (MFH) reduces prostate cancer growth in the orthotopic dunning R3327 rat model. Prostate 2005; 64: 283–292
- Yu Z, Xiaoliang W, Xuman W, Hong X, Hongchen G. Acute toxicity and irritation of water-based dextran-coated magnetic fluid injected in mice. J Biomed Mater Res 2008; 85A: 582–587
- Suzuki M, Shinkai M, Honda H, Kobayashi T. Anticancer effect and immune induction by hyperthermia of malignant melanoma using magnetite cationic liposomes. Melanoma Res 2003; 13: 129–135
- Nikfarjam M, Malcontenti-Wilson C, Christophi C. Focal hyperthermia produces progressive tumor necrosis independent of the initial thermal effects. J Gastrointest Surg 2005; 9: 410–417
- Vertrees RA, Das GC, Coscio AM, Xie JW, Zwischenberger JB, Boor PJ. A mechanism of hyperthermia-induced apoptosis in ras-transformed lung cells. Mol Carcinog 2005; 44: 111–121
- Gnant MF, Noll LA, Terrill RE, Wu PC, Berger AC, Nguyen HQ, Lans TE, Flynn BM, Libutti SK, Bartlett DL, et al. Isolated hepatic perfusion for lapine liver metastases: Impact of hyperthermia on permeability of tumor neovasculature. Surgery 1999; 126: 890–899
- Nikfarjam M, Muralidharan V, Malcontenti-Wilson C, Christophi C. Progressive microvascular injury in liver and colorectal liver metastases following laser induced focal hyperthermia therapy. Lasers Surg Med 2005; 37: 64–73
- Sato T, Sawaji Y, Matsui N, Sato H, Seiki M, Mori Y, Ito A. Heat shock suppresses membrane type 1-matrix metalloproteinase production and progelatinase A activation in human fibrosarcoma HT-1080 cells and thereby inhibits cellular invasion. Biochem Biophys Res Commun 1999; 265: 189–193
- Komata T, Kanzawa T, Nashimoto T, Aoki H, Endo S, Nameta M, Takahashi H, Yamamoto T, Kondo S, Tanaka R. Mild heat shock induces autophagic growth arrest, but not apoptosis in U251-MG and U87-MG human malignant glioma cells. J Neurooncol 2004; 68: 101–111
- Nakahata K, Miyakoda M, Suzuki K, Kodama S, Watanabe M. Heat shock induces centrosomal dysfunction, and causes non-apoptotic mitotic catastrophe in human tumour cells. Int J Hyperthermia 2002; 18: 332–343
- Gnant MF, Turner EM, Alexander HR, Jr. Effects of hyperthermia and tumour necrosis factor on inflammatory cytokine secretion and procoagulant activity in endothelial cells. Cytokine 2000; 12: 339–347
- Nikfarjam M, Muralidharan V, Christophi C. Mechanisms of focal heat destruction of liver tumors. J Surg Research 2005; 127: 208–223
- Eikesdal HP, Bjorkhaug ST, Dahl O. Hyperthermia exhibits anti-vascular activity in the s.c. BT4An rat glioma: Lack of interaction with the angiogenesis inhibitor batimastat. Int J Hyperthermia 2002; 18: 141–152
- Nikfarjam M, Muralidharan V, Malcontenti-Wilson C, Christophi C. The apoptotic response of liver and colorectal liver metastases to focal hyperthermic injury. Anticancer Res 2005; 25(2B)1413–1419
- Berry CC, Wells S, Charles S, Curtis ASG. Dextran and albumin derivatised iron oxide nanoparticles: Influence on fibroblasts in vitro. Biomaterials 2003; 24: 4551–4557
- Lai SX, Xiao XS, Lai HW, Xian J, Yang J, Wang JT, Su ZG. Experimental study of inductive apoptosis of Hep-6 in liver tumor cells line by magnetic nanoparticle encapsulated epirubicin. Chinese J Clin Rehabil 2003; 7: 2812–2813
- Guedes MHA, Sadeghiani N, Peixoto DLG, Coelho JP, Barbosa LS, Azevedo RB, Kuckelhaus S, Silva MFD, Morais PC, Lacava ZGM. Effects of AC magnetic field and carboxymethyldextran-coated magnetite nanoparticles on mice peritoneal cells. J Magn Magn Mater 2005; 293: 283–286