Abstract
The analysis of chromosomal aberrations by premature chromosome condensation (PCC) induced by Calyculin A (Cal) is feasible in tumor biopsies from patients and has the potential to predict sensitivity to radiotherapy. As hyperthermia (HT) improves radiotherapy outcome in certain tumor sites, it was investigated whether PCC induction is still possible after temperatures reached in the clinic. Human cervical carcinoma (CaSki) and lung carcinoma (SW-1573) cells were incubated with Cal to induce PCC immediately after 1 h treatment at temperatures ranging from 41°C to 43°C and after recovery for up to 24 h after treatment with 43°C. Levels of phosphorylated Cdc2 (at the Tyr15 residue), histone H3 (at the Ser10 residue) and Cyclin B1 were investigated by immunoblotting. The amount of cells positive for phosphorylated histone H3 was determined by flow cytometry. Temperatures ≥42.5°C inhibited the induction of PCC by Cal, while recovery of PCC-induction was observed at >20 h after treatment in both cell lines. The phosphorylation status of Cdc2 as well as of histone H3 in cells treated with Cal directly after HT at 43°C was similar to that of cells treated with Cal alone or treated with Cal 24 h after HT at 43°C. HT alone did not affect the levels of phosphorylated Cdc2, while phosphorylation levels of histone H3 were increased as compared with control status of these two proteins. Phosphorylated and total Cyclin B1 levels were not influenced by any of the treatments. Flow cytometric analysis confirmed that HT at 43°C did not interfere with phosphorylation of histone H3. Our data indicate that HT transiently inhibits PCC induction by Cal in a temperature-dependent manner. Therefore, an interval of at least 24 h after HT should be applied before taking tumor biopsies for karyogram analysis of patients treated with temperatures above 42.5°C.
Introduction
The analysis of chromosomal aberrations in tumor biopsies with the potential to predict radiation treatment outcome is feasible in colorectal Citation1 and cervical carcinoma Citation2. Premature chromosome condensation followed by fluorescent in situ hybridization (FISH) enables the direct visualization of chromosomal aberrations by the induction of chromosome condensation in interphase cells Citation1–4. PCC induction can be accomplished by fusion of cells with mitotic cells or by using chemicals, such as Calyculin A Citation3,Citation4.
Cell-cycle independent induction of PCC by Cal is thought to be caused by inhibition of type-1 (PP1) and type-2 phosphatases (PP2A) Citation3–7. These phosphatases are involved in the physiological regulation of chromosome condensation during mitosis by regulating the activity of the phosphatase Cdc25 and the maturation/mitosis promoting factor (MPF), a complex of p34cdc2 (Cdc2) and Cyclin B Citation7–11. In the inactive form of MPF, the Cdc2 protein is phosphorylated at Tyr15 (Cdc2-PTyr15) and Thr14 residues Citation12. Dephosphorylation of Cdc2-PTyr15 by Cdc25 is important for entry of cells into mitosis Citation11 and has been associated with chromosome condensation Citation13,Citation14. In mitotic cells, phosphorylation of the histone H3 at the Ser10 residue (H3-PSer10) is described as occurring at high levels and correlates with mitotic chromosome condensation Citation15,Citation16. H3-PSer10 is used as a marker for mitotic cells and promotes the recruitment of chromosomal condensation factors necessary for normal mitotic assembly and segregation Citation17. Enhanced H3-PSer10 is also associated with PCC induction after incubation of cells with Cal Citation18–21. Also, cyclin B1 levels may play a role in the studied effects and hyperthermia induced chromosome condensation and increases in cellular cyclin B1 levels by hyperthermia have been reported by Mackey et al. Citation22.
Recently, we have shown that chromosome fragments have the potential to predict radiosensitization by hyperthermia (HT) at temperatures reached in the clinic Citation23. Iliakis et al. have reported previously that HT (8–15 minutes at 45.5°C) reduces the ability of chromosomes to condense after fusion with mitotic cells and suggested that accumulation of proteins on the chromatin might prevent protein phosphorylation necessary for chromatin condensation Citation24. It has already been shown that HT can affect the phosphorylation status of histone proteins, such as H2AX Citation25–27. The protein accumulation in the nucleus might be due to denaturation of proteins Citation28, when cells are treated at temperatures at or above 42.5°C. Since clinically more relevant temperatures below 42.5°C might not result in protein denaturation Citation28–30, the present study investigates the temperature-dependent induction of PCC by Cal and the phosphorylation status of two key regulators of chromosome condensation in two human tumor cell lines.
Materials and methods
Cell culture
The human cervical cancer cell line CaSki was grown in Dulbecco's modified Eagle medium (DMEM) with 4500 mg/L glucose (GIBCO-BRL Life Technologies, Breda, the Netherlands) supplemented with 10% heat-inactivated fetal bovine serum (FBS) and 2 mM glutamine and kept at 37°C in a humidified incubator with a 10% CO2/90% air atmosphere. The SW-1573 cell line was grown in Leibowitz-15 (GIBCO-BRL Life Technologies, Breda, the Netherlands) supplemented with 10% FBS and glutamine, penicillin and streptomycin. The cells were maintained at 37°C in an incubator with humidified air without additional CO2. Both cell lines were maintained as monolayers in tissue culture flasks (Costar Europe Ltd, Badhoevedorp, the Netherlands). The cultures were passaged twice a week to ensure exponential growth.
Processing of human biopsies
After surgical resection from patients, tumour biopsies were placed in phosphate buffered saline. The tumour samples were cut in small pieces and digested for 1–4 h at 37°C in high glucose DMEM supplemented with 0.14 units/ml collagenase/dispase enzyme solution (Blendzyme I, Roche, Indianapolis, IN, USA). After the digestion, samples were dislodged by pipetting the tissue up and down and centrifuged for 5 min at 68 g. The supernatant was removed and the pellet was resuspended in a wash medium containing high glucose DMEM supplemented with 5% FCS, 100 U/ml penicillin, 100 mg/ml streptomycin and 2 mM glutamine. The samples were centrifuged for 5 min at 68 g, and by removing the supernatant mainly loose cells and bacteria were removed. This washing step was repeated once. The remaining organoids were transferred to CellBIND® (Corning, Lowell, MA, USA) 6-well plates in RPMI supplemented with 10% FCS, 100 U/ml penicillin, 100 mg/ml streptomycin, 25 μg/ml gentamycin, 1 μg/ml fungizone and 2 mM glutamine and incubated at 37°C, 5% CO2 for 1–2 days before hyperthermia treatment.
Hyperthermia treatment
Cultures consisting of approximately 49% G1, 34% S and 17% G2/M (CaSki) or 45% G1, 46% S and 10% G2/M (SW-1573) as determined by BrdU labeling and flow cytometry were treated. Hyperthermia treatment was performed by submerging the Petri dishes in a thermostatically controlled water-bath for 1 h. The atmosphere of the water-bath was adjustable by a connection with air and CO2 supplies. CaSki cells were heated in a 10% CO2/90% air atmosphere with an air inflow of 2 L/min. SW-1573 cells did not receive additional CO2.
Premature chromosome condensation
To induce premature chromosome condensation (PCC), 80 nM of Calyculin A (Santa Cruz Biotechnology, CA) was added for 1 h immediately after HT at temperatures ranging from 41°C to 43°C Citation3. To test whether the effect of HT at 43°C on PCC induction was transient, cells were incubated for 0 h to 24 h at 37°C after HT treatment before the addition of Cal. Cells were collected and treated according to standard cytogenetic procedures to obtain chromosome spreads. Briefly, cell pellets were resuspended in a hypotonic solution of 75 mM KCl, incubated for 16 min at 37°C and fixated in glacial acetic acid/methanol (1:3 v/v). Chromosome spreads were obtained by dropping the fixated cells on to pre-cleaned slides and air dried. Chromosomes were stained using 10% Giemsa (Merck, Darmstadt, Germany) solution and investigated by light microscopy (Leica, Rijswijk, the Netherlands). The percentage of PCC spreads of G1 and G2 phase cells (G1 + G2 PCC index) was determined by counting 200 nuclei per slide. For each sample, at least two slides were scored. Experiments were repeated three times.
Immunoblotting
Whole-cell lysates were prepared in Laemmli buffer. Protein concentration was determined using the BCA Protein Assay Reagent (Pierce, Rockford, IL, USA). Samples were diluted in 3 × reduced buffer before loading on to 10–15% polyacrylamide gels. Separated proteins were transferred to polyvinylidene difluoride membranes and hybridized overnight at 4°C with antibodies recognizing Cdc2-PTyr15, P-Cyclin B1 and total H3 (rabbit polyclonals 9111, 4131 and 9715, Cell Signaling Technology, Danvers, MA, USA), H3-PSer10 (rabbit polyclonal 23136, Upstate Biotechnology, Lake Placid, NY, USA), total Cdc2 and Cyclin B1 (mouse monoclonal C12720 and 610219, BD transduction laboratories, Franklin Lakes, NJ, USA) or β-Actin (mouse monoclonal AC-15, Sigma-Aldrich, Zwijndrecht, the Netherlands). Membranes were probed with secondary IgG HRP-linked antibodies anti-rabbit or anti-mouse (Cell Signaling Technology, Boston, MA), incubated with Enhanced Chemiluminescence Plus (Amersham Biosciences, Little Chalfont, UK) and visualized using a ChemiDoc XRS system with Quantity One (version 4.4) software (BioRad Laboratories, Hercules, CA, USA).
Flow cytometric analysis of phosphorylation of histone H3
Cells were harvested, fixated in 70% ethanol and incubated at −20°C for at least 24 h. For flow cytometric detection of H3PSer10, cells were permeabilized using 0.25% Triton X-100 and incubated on ice for 15 min. Cell pellets were then resuspended in rabbit polyclonal H3-PSer10 (23136) followed by 50 µg/ml FITC-conjugated goat anti-rabbit secondary antibody (Jackson Immuno Research Laboratories, Suffolk, UK). As isotype (negative) controls, cell pellets were resuspended in rabbit α-Helicobacter Pylori primary antibody (Dako, Glostrup, Denmark). Cells were then stained using 25 µg/ml of propidium iodide (PI) containing 0.1 mg/ml RNase A. Experiments were repeated three times. To define H3-PSer10 positivity, a positive control consisting of mitotic cells obtained by shake-off was used as a reference by determination of a tetraploid (4N) H3-PSer10 positive population. The cut-off level was used for determination of H3-PSer10 positive cells in HT and/or Cal treated cultures. In addition to H3-PSer10 positivity, cell cycle distribution was determined by measurement of PI content in CaSki and SW-1573 cultures. Analysis of flow cytometry data was performed by using WinMDI version 2.9.
Statistics
Data on the induction of PCC were analyzed using SPSS 14.0 (Chicago, IL) statistical software using the non-parametric Mann-Whitney test.
Results
Transient inhibition of PCC-induction by Cal in a temperature-dependent manner
To investigate the effect of HT on PCC induction by Cal, cells were incubated with Cal immediately after temperatures in the range of 41°C to 43°C. In control cultures, the total PCC-index (S-, G1- and G2-phase PCC) was 23% ± 4% (SEM) for CaSki cells and 19% ± 5% (SEM) for SW-1573 cells. The majority (for both cell lines around 60%) of the nuclei showing PCC consisted of S-phase nuclei, recognizable by their pulverized appearance (). From the remaining PCC, the vast majority (±98%) were G2-phase cells with two distinct chromatids (), whereas ±2% were G1-phase cells with one chromatid ().
Figure 1. (A–C) Typical examples of S-phase (A), G2-phase (B) and G1-phase (C) PCC in SW-1573 cells. Magnification: 1,000 x. (D) Total PCC induction, and (E) induction of PCC in G1 and G2 phase cells by Cal in cells immediately after hyperthermia treatment at various temperatures for 1 h and in thermotolerant (TT) cells treated at the indicated temperature. Means with standard errors of three independent experiments. (F–G) Representative examples of SW-1573 nuclei after incubation of cells with Cal for 1 h to induce PCC. (F) untreated cells: two G2 phase PCC spreads and 6 non-condensed nuclei are visible, and (G) cells treated with 43°C hyperthermia (1 h), arrows: condensation of nuclei not resulting in chromosomal spreads. Magnification: 200 x.
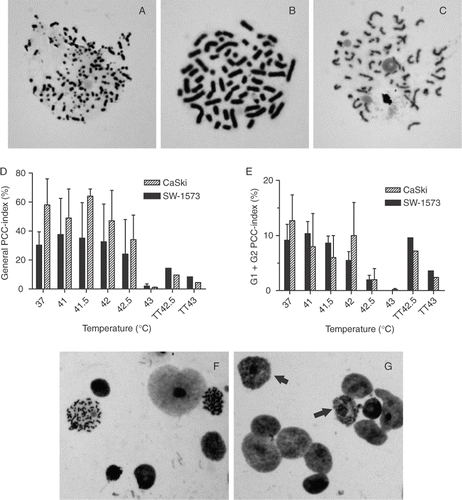
Only in cells treated at temperatures ≥42.5°C PCC induction was significantly decreased (p = 0.04 for 42.5°C and p = 0.02 for 43°C) compared to untreated cells (). Representative examples of nuclei after incubation of SW-1573 cells with Cal without and with prior HT at 43°C are shown in respectively. In HT-treated cells, condensation of nuclei was visible, but it did not result in chromosome spreads (). In cells derived from biopsies of cervical tumors from several patients after in vitro treatment we found 1% scorable PCCs directly after HT at 43°C while in untreated biopsy-derived cells this is between 10% and 15%. These results indicate that HT at ≥42.5°C inhibits the induction of PCC by Cal.
Whether cells were able to recover from this inhibition of PCC-induction was investigated by incubating cells with Cal at various time points after HT at 43°C (). The results show that the inhibitory effect on PCC-induction persisted for 16 h after treatment in both cell lines. In addition, we observed that the inhibition of PCC-induction by HT at ≥42.5°C was reduced in cells, which were made thermotolerant by HT for 1 h at 41°C 6 h prior to the challenging HT treatment.
Phosphorylation status of Cdc2, H3 and Cyclin B1 does not correlate with PCC induction by Cal without or with HT at 43°C
Since dephosphorylation of Cdc2-PTyr15 and phosphorylation of H3-PSer10 are implicated in chromosome condensation Citation14–17, it was investigated whether HT at 43°C had an effect on the phosphorylation status of both proteins. As compared to control cells, dephosphorylation of Cdc2-PTyr15 and phosphorylation of H3-PSer10 was observed in SW-1573 cells treated with Cal similar to the levels found in mitotic cells (). These changes in phosphorylation were also found in cells treated with Cal both directly and at 24 h after HT at 43°C. Total Cdc2 levels were slightly reduced 24 h after HT treatment at 43°C only. Similar results were obtained for CaSki cells (data not shown), suggesting that the overall phosphorylation status directly after HT at 43°C could not explain the observed inhibition of PCC-induction by Cal. Phosphorylated and total Cyclin B1 levels were not influenced by any of the treatments ().
Cell cycle dependent phosphorylation of H3-PSer10 by Cal is not influenced by HT at 43°C
To establish whether HT at 43°C influenced the phosphorylation status of H3-PSer10 on a single cell basis, bivariate flow cytometric analysis was performed. In untreated CaSki cultures, most of the cells were in G0/G1 without H3-PSer10-positivity (). In mitotic cell suspensions obtained by shake-off, a tetraploid (4N) H3-PSer10 positive mitotic population was clearly visible (). When cells were incubated for 1 h with Cal, the total amount of H3-PSer10 positive cells (both 2N, G0/G1 phase and 4N, G2/M phase) was increased (). Treatment of cells for 1 h with HT at 43°C did not affect Cal-induced H3-PSer10 (). The quantification of 2N and 4N of both CaSki and SW-1573 cells positive for H3-PSer10 are shown in .
Figure 4. Phosphorylation of H3-PSer10 is not associated with Cal induced PCC inhibition by 1 h hyperthermia treatment at 43°C. Representative examples of three independent experiments are shown for CaSki cells (A–E) y-axis: H3-PSer10fluorescence, x-axis: DNA content propidium iodide fluorescence; (A) Untreated, (B) mitotic shake-off cell suspension, (C) 1 h Cal, (D) Cal 0 h after HT at 43°C, (E) Cal 24 h after HT at 43°C. Regions are defined as H3-PSer10 negative (R1), H3-PSer10 positive 2N (R2) and H3-PSer10 positive 4N (R3). Quantification of H3-PSer10 positive 2N (F) and 4N (G) CaSki and SW-1573 cells.
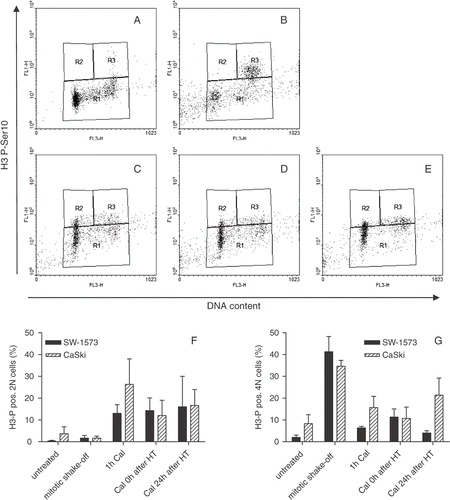
Discussion
In the present study we show that HT for 1 h transiently inhibits PCC induction by Cal at temperatures above 42.5°C. Apparently there is a temperature threshold for inhibition of proteins or structures important for PCC induction by Cal. Inhibition of PCC induction by the PEG-mediated cell fusion technique after HT at 43°C and at 45.5°C has been reported earlier for Chinese hamster ovary cells Citation24. The ability of chromatin to condense by fusion with mitotic (unheated) cells decreased with increasing heating time and reached a plateau after 20 min of heating at 45.5°C. HT at 43°C totally inhibited induction of PCC after a 70 min heating period Citation24.
Our data indicate that the inhibition of PCC induction by HT at 43°C was transient in both cell lines. The recovery of PCC index was observed between 16 and 20 h and was complete at 24 h after HT. These findings are in line with the results of Iliakis et al. Citation24, who observed a recovery period for PCC index of 12 h after HT at 45.5°C for 8 min and over 24 h after HT at 45.5°C for 15 min. The recovery of PCC index over time might be related to the expression of heat shock proteins that protect proteins from denaturation by heat Citation31,Citation32. This hypothesis is underscored by our observation that the inhibition of PCC-induction by HT above 42.5°C was reduced in thermotolerant cells. A similar recovery time of heat-induced changes in chromatin structure and enzyme activities of 12–24 h after HT treatment ≥45°C, for 15–17 min has been reported by several others Citation33–35. However, the induction of heat shock proteins and of thermo-tolerance has been reported to occur earlier Citation31,Citation36 than the recovery of the PCC index. Therefore, mechanisms other than thermotolerance must play a role.
Nuclear proteins appear to be particularly sensitive to HT-induced protein denaturation Citation37–40. Denaturation of thermolabile proteins results in the aggregation and biological inactivation of important proteins Citation28, affecting important processes like DNA repair and synthesis Citation41, and inducing chromatin alterations Citation27. Since dephosphorylation of the Tyr15 residue of Cdc2 has been associated with activation of the MPF and entry of cells into mitosis and/or chromosomal condensation Citation42, we reasoned that HT at 43°C would interfere with this process. However, our results indicate Cdc2 phosphorylation is not associated with artificial chromosome condensation. This may be explained by observations that entry of cells into mitosis do not require Cdc2 activity Citation43. The authors suggest that other proteins, such as histone H1 Citation44 and Cdc25C (independent from its function in Cdc2 kinase activation), might also be involved in chromosome condensation and G2/M transition.
A further downstream event in chromosome condensation is the phosphorylation of H3-PSer10, which has been associated with PCC induction after incubation of cells with Cal Citation18–20. Cells treated with Cal alone showed H3-PSer10 positive cells in all phases of the cell cycle, which explains the occurrence of G1, S as well as G2 phase PCCs in our study and is in agreement with others Citation19. Surprisingly, however, we could not find an effect of HT at 43°C on the phosphorylation status of H3-PSer10 in whole cell lysates nor in single-cell-based flow cytometry. Phosphorylation of H3-PSer10 has been found to occur at transcriptionally activated heat shock loci implying a role for H3-PSer10 in the regulation of gene expression in addition to its role in chromosome condensation Citation17. However, H3-PSer10 phosphorylation associated with transcriptional activation after heat has been shown to be a rapid and transient event and affects a cell population distinct from that normally detected in mitotic cells Citation45. In contrast to transcription-associated H3-PSer10 phosphorylation, condensed chromosomes exhibit very strong H3-PSer10 right across chromosomes Citation46.
The appearance of some condensed nuclei after Cal treatment preceded by HT indicates that a certain level of DNA condensation can take place without formation of chromosomal spreads. This suggests that a late stage in the process of PCC is inhibited by HT. Iliakis et al. suggested that heat-induced alterations in the chromatin, nucleolus and the nuclear membrane might be involved in PCC inhibition as these structures serve as substrates for enzymatic reactions necessary for PCC Citation23. According to our results, the effect of HT should be downstream of the phosphorylation of H3-PSer10. The HT-induced aggregation of proteins in the nuclear matrix can result in inhibition of DNA replication Citation38. This might be another explanation for inhibition of PCC by HT as a recent study shows the tight coupling of chromosome condensation to DNA replication by drug-induced PCC Citation47.
In summary, the analysis of chromosomal aberrations in tumor biopsies from patients is currently feasible and has the potential to predict radiation treatment outcome both alone and in combination with HT at temperatures reached in the clinic Citation1,Citation2,Citation23. In this report we show that HT for 1 h at temperatures ≥42.5°C inhibited PCC induction by Cal. This inhibition was transient, as recovery to the control levels was complete in a period of 24 h. Therefore, we conclude that an interval of at least 24 h after HT should be applied, when tumor biopsies are taken from patients treated with temperatures near or above 42.5°C.
Acknowledgements
The Dutch Cancer Foundation KWF is gratefully acknowledged for financial support (project number UVA 2008/4019). The Nijbakker Morra and Maurits and Anna de Kock foundations are acknowledged for sponsoring laboratory equipment.
Declaration of interest: The authors report no conflicts of interest. The authors alone are responsible for the content and writing of the paper.
References
- Bezrookove V, Smits R, Moeslein G, Fodde R, Tanke HJ, Raap AK, Darroudi F. Premature chromosome condensation revisited: A novel chemical approach permits efficient cytogenetic analysis of cancers. Genes Chromosomes Cancer 2003; 38: 177–186
- Bergs JWJ. Hyperthermia, cisplatin and radiation trimodality treatment: In vitro studies on interaction mechanisms. Thesis. University of Amsterdam. 2007
- Gotoh E, Asakawa I, Kosaka H. Inhibition of protein serine/threonine phosphatases directly induces premature chromosome condensation in mammalian somatic cells. Biomed Res 1995; 16: 63–68
- Kawata T, Ito H, Uno T, Saito M, Yamamoto S, Furusawa Y, Durante M, George K, Wu H, Cucinotta FA. G2 chromatid damage and repair kinetics in normal human fibroblast cells exposed to low- or high-LET radiation. Cytogen Genome Res 2004; 104: 211–215
- Alsbeih G, Raaphorst GP. Differential induction of premature chromosome condensation by Calyculin A in human fibroblast and tumor cell lines. Anticancer Res 1999; 19: 903–908
- Kanda R, Eguchi-Kasai K, Hayata I. Phosphatase inhibitors and premature chromosome condensation in human peripheral lymphocytes at different cell-cycle phases. Somat Cell Mol Gen 1999; 25: 1–8
- Gotoh E, Durante M. Chromosome condensation outside of mitosis: Mechanisms and new tools. J Cell Physiol 2006; 209: 297–304
- Maller J, Gautier J, Langan TA, Lohka MJ, Shenoy S, Shalloway D, Nurse P. Maturation-promoting factor and the regulation of the cell cycle. J Cell Sci 1989; 12: 53–63
- Dunphy WG, Brizuela L, Beach D, Newport J. The Xenopus cdc2 protein is a component of MPF, a cytoplasmic regulator of mitosis. Cell 1988; 54: 423–431
- Gautier J, Norbury C, Lohka M, Nurse P, Maller J. Purified maturation-promoting factor contains the product of a Xenopus homolog of the fission yeast cell cycle control gene cdc2+. Cell 1988; 54: 433–439
- Norbury C, Nurse P. Animal cell cycles and their control. Ann Rev Biochem 1992; 61: 441–470
- Lew DJ, Kornbluth S. Regulatory roles of cyclin dependent kinase phosphorylation in cell cycle control. Curr Opin Cell Biol 1996; 8: 795–804
- Janssens V, Goris J. Protein phosphatase 2A: A highly regulated family of serine/threonine phosphatases implicated in cell growth and signalling. Biochem J 2001; 353: 417–439
- Moreno S, Hayles J, Nurse P. Regulation of p34Cdc2 protein kinase during mitosis. Cell 1989; 58: 361–372
- Hendzel MJ, Wei Y, Mancini MA, Van Hooser A, Ranalli T, Brinkley BR, Bazett-Jones DP, Allis CD. Mitosis-specific phosphorylation of histone H3 initiates primarily within pericentromeric heterochromatin during G2 and spreads in an ordered fashion coincident with mitotic chromosome condensation. Chromosoma 1997; 106: 348–360
- Wei Y, Mizzen CA, Cook RG, Gorovsky MA, Allis CD. Phosphorylation of histone H3 at serine 10 is correlated with chromosome condensation during mitosis and meiosis in Tetrahymena. Proc Nat Acad Sci USA 1998; 95: 7480–7484
- Johansen KM, Johansen J. Regulation of chromatin structure by histone H3S10 phosphorylation. Chromosome Res 2006; 14: 393–404
- Huang X, Kurose A, Tanaka T, Traganos F, Dai W, Darzynkiewicz Z. Sequential phosphorylation of Ser-10 on histone H3 and ser-139 on histone H2AX and ATM activation during premature chromosome condensation: Relationship to cell-cycle phase and apoptosis. Cytometry 2006; 69: 222–229
- Guo XW, Th'ng JP, Swank RA, Anderson HJ, Tudan C, Bradbury EM, Roberge M. Chromosome condensation induced by fostriecin does not require p34Cdc2 kinase activity and histone H1 hyperphosphorylation, but is associated with enhanced histone H2A and H3 phosphorylation. EMBO J 1995; 14: 976–985
- Ajiro K, Yoda K, Utsumi K, Nishikawa Y. Alteration of cell cycle-dependent histone phosphorylations by okadaic acid. Induction of mitosis-specific H3 phosphorylation and chromatin condensation in mammalian interphase cells. J Biol Chem 1996; 271: 13197–13201
- Bui HT, Yamaoka E, Miyano T. Involvement of histone H3 (Ser10) phosphorylation in chromosome condensation without Cdc2 kinase and mitogen-activated protein kinase activation in pig oocytes. Biol Reprod 2004; 70: 1843–1851
- Mackey MA, Zhang XF, Hunt CR, Sullivan SJ, Blum J, Laszlo A, Roti Roti JL. Uncoupling of M-phase kinase activation from the completion of S-phase by heat shock. Cancer Res Apr 15, 1996; 56(8)1770–1774
- Bergs JW, Cate RT, Haveman J, Medema JP, Franken NA, Bree CV. Chromosome Fragments have the Potential to Predict Hyperthermia-induced Radio-sensitization in Two Different Human Tumor Cell Lines. J Radiat Res (Tokyo) Sep, 2008; 49(5)465–72, Epub 2008 Apr 15
- Iliakis GE, Pantelias GE. Effects of hyperthermia on chromatin condensation and nucleoli disintegration as visualized by induction of premature chromosome condensation in interphase mammalian cells. Cancer Res 1989; 49: 1254–1260
- Takahashi A, Matsumoto H, Nagayama K, Kitano M, Hirose S, Tanaka H, Mori E, Yamakawa N, Yasumoto J, Yuki K, et al. Evidence for the involvement of double-strand breaks in heat-induced cell killing. Cancer Res 2004; 64: 8839–8845
- Kaneko H, Igarashi K, Kataoka K, Miura M. Heat shock induces phosphorylation of histone H2AX in mammalian cells. Biochem Biophys Res Comm 2005; 328: 1101–1106
- Hunt CR, Pandita RK, Laszlo A, Higashikubo R, Agarwal M, Kitamura T, Gupta A, Rief N, Horikoshi N, Baskaran R, et al. Hyperthermia activates a subset of ataxia-telangiectasia mutated effectors independent of DNA strand breaks and heat shock protein 70 status. Cancer research 2007; 67: 3010–3017
- Lepock JR. How do cells respond to their thermal environment?. Int J Hyperthermia 2005; 21: 681–687
- Hall EJ. Radiobiology for the radiologist. Lippincott Company, Philadelphia 1988
- Overgaard J. Effect of hyperthermia on malignant cells in vivo. A review and a hypothesis. Cancer 1977; 39: 2637–2646
- Li GC, Petersen NS, Mitchell HK. Induced thermal tolerance and heat shock protein synthesis in Chinese hamster ovary cells. Int J Radiat Oncol Biol Phys 1982; 8: 63–67
- Ellis RJ, van der Vies SM. Molecular chaperones. Ann Rev Biochem 1991; 60: 321–347
- Clark EP, Dewey WC, Lett JT. Recovery of CHO cells from hyperthermic potentiation to X-rays repair of DNA and chromatin. Radiat Res 1981; 85: 302–313
- Weissenborn U, Obe G. Modification of X-ray induced chromosome aberration frequency by pre- and postirradiation hyperthermia of human peripheral lymphocytes. Int J Radiat Biol 1991; 59: 973–984
- Tomasovic SP, Turner GN, Dewey WC. Effect of hyperthermia on nonhistone proteins isolated with DNA. Radiat Res 1978; 73: 535–552
- Diller KR. Stress protein expression kinetics. Ann Rev Biomed Eng 2006; 8: 403–424
- Lepock JR, Frey HE, Heynen ML, Senisterra GA, Warters RL. The nuclear matrix is a thermolabile cellular structure. Cell Stress Chaperones 2001; 6: 136–147
- Roti Roti JL, Kampinga HH, Malyapa RS, Wright WD, vanderWaal RP, Xu M. Nuclear matrix as a target for hyperthermic killing of cancer cells. Cell Stress Chaperones 1998; 3: 245–255
- Lepock JR. Role of nuclear protein denaturation and aggregation in thermal radiosensitization. Int J Hyperthermia 2004; 20: 115–130
- Lepock JR, Frey HE, Ritchie KP. Protein denaturation in intact hepatocytes and isolated cellular organelles during heat shock. J Cell Biol 1993; 122: 1267–1276
- Hildebrandt B, Wust P, Ahlers O, Dieing A, Sreenivasa G, Kerner T, Felix R, Riess H. The cellular and molecular basis of hyperthermia. Crit Rev Oncol Hematol 2002; 43: 33–56
- Ito J, Hirabayashi M, Kato M, Takeuchi A, Ito M, Shimada M, Hochi S. Contribution of high p34Cdc2 kinase activity to premature chromosome condensation of injected somatic cell nuclei in rat oocytes. Reproduction 2005; 129: 171–180
- Gowdy PM, Anderson HJ, Roberge M. Entry into mitosis without Cdc2 kinase activation. J Cell Sci 1998; 111: 3401–3410
- Tosuji H, Fusetani N, Seki Y. Calyculin A causes the activation of histone H1 kinase and condensation of chromosomes in unfertilized sea urchin eggs independently of the maturation-promoting factor. Comp Biochem Physiol C Toxicol Pharmacol 2003; 135: 415–424
- Nowak SJ, Corces VG. Phosphorylation of histone H3: A balancing act between chromosome condensation and transcriptional activation. Trends Genet 2004; 20: 214–220
- Dyson MH, Thomson S, Mahadevan LC. Heat shock, histone H3 phosphorylation and the cell cycle. Cell Cycle 2005; 4: 13–17
- Gotoh E. Visualizing the dynamics of chromosome structure formation coupled with DNA replication. Chromosoma 2007; 116: 453–462