Abstract
The effects of hyperthermia on the oxygenation status in R3230 AC tumours of Fischer rats were measured using a polarographic oxygen electrode system. The median pO2 in about 10 mm diameter tumours grown s.c. in the leg of rats was 3.7 ± 0.3 mm Hg and it significantly increased upon heating at modest temperatures. For example, the tumour pO2 measured within 10–15 min after heating for 30 min at 42.5°C was about three-fold greater than that in the control tumours. About 62% of pO2 values measured in control tumours were <5 mm Hg. After heating at 42.5°C for 30 min, 37% of pO2 values were <5 mm Hg. Such an increase in tumour oxygenation or reoxygenation of hypoxic cells appeared to result from an increase in tumour blood flow caused by the mild temperature hyperthermia. The presence of hypoxic cells in tumours is believed to be a major factor in limiting the effectiveness of radiotherapy, certain chemotherapy drugs and phototherapy. Hyperthermia at mild temperatures easily achievable with the use of presently available clinical hyperthermia devices may be an effective means to overcome the hypoxic protection in the treatment of human tumours.
Introduction
Hypoxic cells are about three-fold radioresistant relative to oxygenated cells, and hypoxic cells are also resistant to certain chemotherapy drugs as well as phototherapy. Indications are that fractions of cells in human tumours are hypoxic and that the presence of such hypoxic cells may be one of the limiting factors for the complete control of human tumours by radiotherapy Citation1–6.
In recent years, the effectiveness of hyperthermia as an adjuvant to radiotherapy has been demonstrated Citation7–8. Experiments with laboratory animal tumours demonstrated that heating for 30–60 min at relatively high temperatures, i.e. >43–44°C, causes damage to tumour blood vessels and kills tumour cells Citation9–12. In addition, hyperthermia causes direct cellular radiosensitization Citation13–15. Unfortunately, with most of the currently used clinical hyperthermia devices, it is not an easy task to raise the human tumour temperature high enough to cause vascular damage, kill the tumour cells, including hypoxic cells, and also to radiosensitize the tumour cells. Oleson Citation16 recently pointed out that the thermal doses in past clinical trials in which hyperthermia was found to be effective were too small for inducing significant direct cell killing as well as thermal radiosensitization. Oleson thus hypothesized that indirect radiosensitization through an increase in blood flow and ensuing reoxygenation of hypoxic cells rather than thermal cytotoxicity and direct thermal radiosensitization could have been the reasons for the increase in tumour response to the combined treatment of hyperthermia and radiotherapy. As Koch et al. Citation17 suggested, improvement of tumour oxygenation by hyperthermia is a compelling hypothesis which warrants experimental investigation. We report here that hyperthermia at modest temperatures, e.g. 41–43°C, increases blood flow and increases oxygenation in a transplantable rat tumour suggesting that hyperthermia at modest temperatures may indeed be an effective means to overcome the hypoxic protection in radiotherapy.
Materials and methods
Tumours
The R3230 adenocarcinoma (R3230 AC tumour) (originally obtained from Dr M. Dewhirst, Duke University) of Fischer rat was used. Stock tumour cells stored frozen in liquid nitrogen are periodically thawed, cultured in vitro and injected subcutaneously (s.c.) into the right flank of male Fischer rats. Tumours are then maintained by serial transplantation of small viable tumour pieces to the right flank of other rats. For the present study, tumours grown in the flank of rats were minced to about 1.5 mm3 pieces and transplanted s.c. into the right hind legs of 250–300 g male Fischer rats. Tumours grown to about 10 mm in diameter, which took 20–30 days, were used for the experiments.
Heating
Tumours grown in the leg of rats were heated with a water bath as we described in detail elsewhere Citation18. Briefly, the rats were anaesthetized by an i.p. injection of a mixture of ketamine (87 mg/kg) and xylazine (13 mg/kg) in about 0.3 ml isotonic saline. Hairs on the tumour-bearing right legs were clipped with an electric clipper. The tumour-bearing legs were immersed into the preheated water through 2.5 cm diameter holes on a plexiglas board. The tumours were heated at 40.5–43.5°C for 30 or 60 min. During the heating, the temperature in the tumour periphery underneath the skin, as measured with 29-gauge needle-type thermocouples, was close to the water temperature while the temperature in the deeper regions was 0.2–0.3°C lower than the water temperature. The rectal temperature rose from the control temperature (36°C) by <1.0–1.5°C during the tumour heating.
Measurement of tumour oxygenation
The partial oxygen tension (pO2) in the tumours was measured with an Eppendorf polarographic oxygen electrode system (Eppendorf, Hamburg, Germany). The principle and technical details of this equipment have been described by Vaupel et al. Citation4. The electrodes were calibrated using isotonic saline solution equilibrated alternatively with air and 100% nitrogen gas immediately before and after measurement of pO2 in each tumour. Immediately after the tumours were heated, the rats were placed on a plexiglas board, the tumour-bearing right leg was gently stretched and affixed by taping the foot to the board. The body temperature (rectal temperature) was maintained at 36–37°C by placing a heating pad under the board. Commercially available self-adhering reference electrodes (SynCor™ National ECG Electrode) were attached to the surface of the shaved dorsal surface of the rats. A small incision was made in the skin on the distal side of tumours and a pO2 sensitive needle-type electrode (300μ diameter) was inserted and horizontally advanced about 1.5 mm deep so that the electrode tip was inside the tumour tissue. The computer-controlled electrode was automatically advanced by about 0.7 mm forward steps, immediately withdrawn by 0.3 mm to reduce the compression pressure, and the pO2 was read. The same procedures were repeated resulting in a 0.4 mm net forward advancement of the electrodes after each pO2 measurement. The length of each track was predetermined according to the geometry of the tumours. The pO2 was measured along 4–5 parallel tracks in the lower plane and 3–4 tracks in the upper plane of each tumour. Along each track, 10–15 pO2 readings were made resulting in 80–120 readings in each tumour. Measurement of pO2 in each tumour was completed within 10–15 min after tumour heating. The measured pO2 value was corrected for tumour temperature measured with a needle-type thermocouple immediately after the pO2 measurement. The pO2 in unheated control tumours was measured 30–60 min after the host rats were anaesthetized.
Data analysis
The information on pO2 values recorded in the Eppendorf histograph was transferred to an IBM personal computer and the data was analysed. The mean and median pO2 values in each tumour were obtained and their average for each group was calculated. The % of pO2 readings <5 mm Hg in each tumour was also obtained and averaged for each experimental group. The non-parametric Mann-Whitney U-test was used for the determination of statistical significance of the differences between the experimental groups. The Wilcoxon Rank Sum Test was used when the sample numbers exceeded 20 in either of the comparing groups.
Results
pO2 parameters in R3230 AC tumours determined immediately after heating at 40.5–43.5°C for 30 or 60 min are shown in . The mean and median pO2 in 25 control tumours were 9.8 ± 0.6 mm Hg and 3.7 ± 0.3 mm Hg respectively. A significant increase in tumour pO2 occurred upon heating at temperatures as low as 40.5°C for 30 min, as indicated by the increase in median pO2 to 5.4 mm Hg (p < 002). shows the histograms of pO2 distribution in tumours heated at 42.5–43.5°C for 30–60 min. A marked increase in pO2 occurred when the tumours were heated at 42.5°C for 30 min; the median pO2 increased to 12.2 ± 1.8 mm Hg, which was about 3-fold greater than the median pO2 in the control tumours (p < 0.001). However, the tumour pO2 returned to control level when the heating at 42.5°C was prolonged to 60 min. The mean and median pO2 in tumours heated at 43.5°C for 30 min also increased to the level similar to those caused by heating at 42.5°C for 30 min. On the other hand, prolonging the heating at 43.5°C to 60 min resulted in tumour pO2 to be significantly lower than the pO2 in control tumours (p < 0.001). shows the changes in the fraction of pO2 readings <5.0 mm Hg, which probably corresponds to about one-half the pO2 for a radiobiologically oxygenated condition. About 62% of all the pO2 readings in 25 control tumours were <5.0 mm Hg and about 37 and 30% of the pO2 readings were below 5 mm Hg when the tumours were heated at 42.5 and 43.5°C for 30 min respectively.
Figure 1. Frequency distribution of pO2 in R3230 AC tumours following heating at 42.5 and 43.5°C for 30 or 60 min. N = number of tumours studied; n = number of pO2 values measured. The mean and median pO2 values in each tumour were obtained and their average for each experimental group ±1 SE is shown.
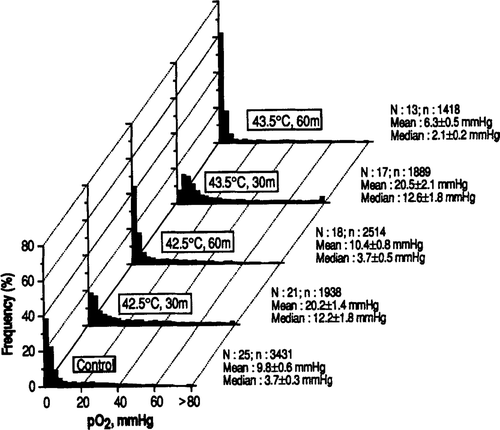
Discussion
The results obtained in the present study unequivocally demonstrated that hyperthermia at modest temperatures significantly improves tumour oxygenation. Although the effect of hyperthermia on tumour oxygenation has been sporadically reported in the past Citation19–20,Citation12,Citation21 this potentially important phenomenon has not been systematically investigated. Bicher et al. Citation19 reported that the pO2 in a C3H mouse mammary adenocarcinoma increased as a result of an increase in tumour blood flow when the tumours were heated at temperatures below 41°C, but it decreased as the heating temperature was raised >41°C. Vaupel et al. Citation22 observed that the oxygenation status in DS-carcinoma of rats significantly improved upon heating at 40°C while it declined when the heating temperature was raised to 43°C. More recently, Hetzel et al. Citation21 reported that the pO2 in a C3H mouse mammary adenocarcinoma grown in the flank increased 24 h after an exposure of the tumour to a TCD10 dose (dose which can cure 10% of tumours in 30 days) of heating at 43.5°C. When the heating dose was increased to TCD 60 (dose which can cure 60% of tumours in 30 days) of heating at 43.5°C, the tumour pO2 decreased at 4 h but increased again at 48 h. The investigators concluded that the improvement of tumour oxygenation after heating with the TCD10 dose resulted probably from an increase in tumour blood flow and that the improvement of tumour oxygenation following the heating at the TCD60 dose resulted mainly from a decrease in oxygen consumption as a result of heat damage to the tumour cells. These results are in agreement with our observations in the present study that the pO2 in R3230 AC tumours markedly increase upon heating at 41.5–43.5°C for 30 min. We have previously observed that hyperthermia at 40–42°C for 1 h markedly improved the oxygenation in FSa II tumours of C3H mice Citation23.
Figure 2. The % frequency of pO2 readings below 5 mm Hg in R3230 AC tumours heated at different temperatures for 30 min are shown. An average for each group ±1 SE is shown.
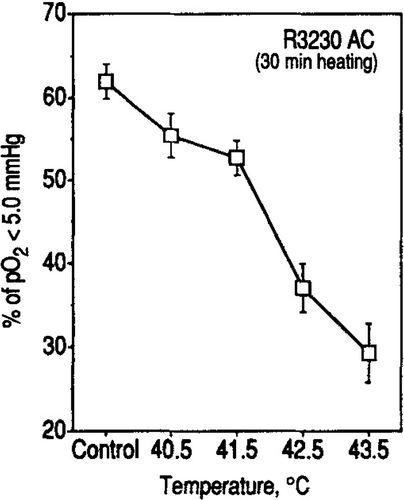
The increase in tumour pO2 by hyperthermia may result either from a decrease in oxygen consumption or an improvement of oxygen supply through an increase in tumour blood flow Citation24. However, oxygen consumption in tumour cells has been reported not to decrease but increase upon heating at 40–42°C Citation25–26,Citation12 Therefore, it would be reasonable to conclude that a decline in oxygen consumption was not the main factor for the improvement of oxygenation in R3230 AC tumours upon heating at 40.5–42.5°C although the possibility that heating at 43.5°C caused cellular injury and decreased oxygen consumption may not be ruled out. It has been known that the newly formed tumour vasculatures are vulnerable to heating Citation9–12. However, the tumour blood flow tends to increase upon heating at relatively mild temperatures. We have indeed observed that heating at 42.5°C for 30 min significantly increases the blood flow in R3230 AC tumours Citation27 indicating that the increase in pO2 in R3230 AC tumours heated at 42.5°C for 30 min in the present study resulted mainly from an increase in tumour blood flow. The decline in tumour pO2 after heating for 60 min at 42.5 or 43.5°C ( and ) may be caused either by a decrease in tumour blood perfusion or an increase in oxygen consumption. Experiments are in progress to elucidate the relationship between pO2, blood flow, and oxygen consumption in heated tumours. As shown in , the pO2 readings <5.0 mm Hg in the tumours significantly decreased upon heating at 40.5–43.5°C for 30 min, indicating significant fractions of the radiobiologically hypoxic cell populations are reoxygenated. The vascular response in human tumours to mild temperature hyperthermia may not be the same as that in rodent tumours. Nevertheless, Waterman et al. Citation28 reported that the blood flow in human tumours was increased by amounts varying from 15 to 250% during the first 20–50 min heating at 41–45°C suggesting that modest thermal dose hyperthermia may improve oxygenation also in human tumours.
Table I. pO2 values in R3230 AC tumours heated for 30 or 60 min at 40.5–43.5°C.
In conclusion, hyperthermia at mild temperatures, i.e. 41.5–42.5°C, which are achievable with presently available hyperthermia technology is an effective means to overcome the hypoxic protection in radiotherapy by reoxygenating the hypoxic cells. Recent results strongly suggest that prolonged heating at mild temperatures also increases the radiosensitivity of tumour cells Citation13–14 The potential usefulness of mild temperature hyperthermia in combination with irradiation in treating human tumours warrant careful examination.
Acknowledgements
The authors would like to thank Dr Dorothy Aeppli for her assistance with the statistical analysis, and Ms Peggy Evans for her assistance in the preparation of the manuscript. This research was supported by NCI grant numbers CA13353 and CA44114.
References
- Coleman CN. Hypoxia in tumors: A paradigm for the approach to biochemical and physiological heterogeneity. J National Can Inst 1988; 80: 310–317
- Adams GE. The clinical relevance of tumour hypoxia. Euro J Cancer 1990; 26: 420–421
- Brown JM. Tumour hypoxia, drug resistance and metastases. J National Can Inst 1990; 82: 338–339
- Vaupel P, Schlenger K, Knoop C, Höckel M. Oxygenation of human tumors: Evaluation of tissue oxygen distribution in breast cancers by computerized O2 tension measurements. Can Res 1991; 51: 3316–3322
- Hockel M, Knoop C, Schlenger K, Vorndran E, Baussmann E, Mitze M, Knapstein G, Vaupel P. Intratumor pO2 predicts survival in advanced cancer of the uterine cervix. Radioth Oncol 1993; 26: 45–50
- Brizel et al. 1994
- Valdagni R, Amichetti M. Report of long-term follow-up in a randomized trial comparing radiation therapy and radiation therapy plus hyperthermia to metastatic lymph nodes in stage IV head and neck patients. Int J Radiat Oncol Biol Phys 1994; 28: 163–169
- Overgaard J, Gonzalez DG, Hulshor MCCM, Archangeli G, Dahl O, Mella O, Bentzen SM. Randomized trial of hyperthermia as adjuvant to radiotherapy for recurrent or metastatic malignant melanoma. The Lancet 1995; 345: 540–543
- Jain RK, Ward-Hartley K. Tumor blood flow-characterization, modifications, and role in hyperthermia. IEEE Trans Sonics Ultrasonics 1984; SU 31: 504–526
- Song CW. Effect of local hyperthermia on blood Sow and micro-environment. Can Res (supplement) 1984; 44: 4721s–4730s
- Reinhold HS, Endrich B. Tumour microcirculation as a target for hyperthermia. Int J Hyperth 1986; 2: 111–137
- Vaupel P. Pathological mechanisms of hyperthermia in Cancer therapy. Biological Basis of Oncologic Thermotherapy, M Gautherie. Springer-Verlag, Heidelberg 1990; 73–134
- Armour EP, Wang Z, Corry PT, Martinez A. Sensitization of Rat 9L gliosarcoma cells to low dose rate irradiation by long duration 41°C hyperthermia. Can Res 1991; 51: 3088–3095
- Mackey MA, Anolik SL, Roti Roti JL. Changes in heat and radiation sensitivity during long duration, moderate hyperthermia in HeLa S3 cells. Int J Radiat Oncol Biol Phys 1992; 24: 543–550
- Dewey W C. Arrhenius relationships from the molecule and cell to clinic. Int J Hyperth 1994; 10: 457–483
- Oleson JR. Hyperthermia from the clinic to the laboratory: a hypothesis (Eugene Robinson Special Lecture). Int J Hyperth 1995; 11: 315–322
- Koch CJ, Evans SM, Lord EM. Comment on the hypothesis that hyperthermia facilitates reoxygenation. Int J Hyperth 1995; 11: 447–450
- Lokshina AM, Song CW, Rhee JG, Levitt SH. Effect of fractionated heating on the blood flow in normal tissues. Int J Hyperth 1985; 1: 117–129
- Bicher HI, Hetzel FW, Sandhu TS, Frinak S, Vaupel P, O’hara D, O'brien T. Effects of hyperthermia on normal and tumour microenvironment. Radiology 1980; 137: 523–530
- Vaupel PW, Otte J, Manz R. Oxygenation of malignant tumors after localized microwave hyperthermia. Radiat Environ Biophys 1982; 20: 289–300
- Hetzel FW, Chopp M, Dereski MO. Variations in pO2 and pH response to hyperthermia: Dependence on transplant site and duration of treatment. Radiat Res 1992; 113: 152–156
- Vaupel et al. 1992
- Iwata K, Shakil A, Hur W-J, Makepeace CM, Griffin RJ, Song CW. Tumor pO2 can be markedly increased by mild hyperthermia. Br J Can 1995, (in press)
- Secomb TW, Hsu R, Ong ET, Gross JF, Dewhirst MW. Analysis of the effect of oxygen supply and demand on hypoxic fraction in tumors. Acta Oncologica 1995; 34(3)313–316
- Durand RE. Potentiations of radiation lethality by hyperthermia in a tumour model: Effects of sequence, degree, and duration of heating. Int J Radiat Oncol Biol Phys 1978; 4: 401–405
- Lepock J, Cheng KH, Al-Qysi H, Sim I, Koch CJ, Kruuv J. Hyperthermia-induced inhibition of respiration and mitochondrial protein denaturation in CHL cells. Int J Hyperth 1987; 3: 123–132
- Nah BS, Choi I-B, Oh WY, Osborn JL, Song CW. Vascular thermotolerance in tumors and normal tissue in rats. Int J Radiat Oncol Biol Phys (in press) 1995
- Waterman FM, Nerlinger RE, Moylan DJ, Leeper DB. Response of human tumor blood flow to local hyperthermia. Int J Radiat Oncol Biol Phys 1987; 13: 75–82