Abstract
Introduction: Mild hyperthermia has been known to enhance the response of tumours to radiotherapy or chemotherapy by increasing tumour blood flow, thereby increasing tumour oxygenation or drug delivery. The purpose of this study was to assess the changes in temperature and blood flow in human cervical cancer in response to regional heating with modulated electro-hyperthermia (mEHT).
Methods: The pelvic area of 20 patients with cervical carcinoma was heated with mEHT. The peri-tumour temperature was measured using an internal organ temperature probe. The tumour blood flow was measured using 3D colour Doppler ultrasound by determining the peak systolic velocity/end-diastolic velocity ratio (S/D ratio) and the resistance index (RI) within blood vessels.
Results: The mean peri-tumour temperature was 36.7 ± 0.2 °C before heating and increased to 38.5 ± 0.8 °C at the end of heating for 60 min. The marked declines in RI and S/D values strongly demonstrated that heating significantly increased tumour blood perfusion.
Conclusions: Regional heating of the pelvic area with mEHT significantly increased the peri-tumour temperature and improved the blood flow in cervical cancer. This is the first demonstration that the blood flow in cervical cancer is increased by regional hyperthermia. Such increases in temperature and blood flow may account for the clinical observations that hyperthermia improves the response of cervical cancer to radiotherapy or chemotherapy.
Introduction
The biological rationale underlying the clinical application of hyperthermia has been well established [Citation1–7]. Indeed, numerous clinical trials have demonstrated that hyperthermia markedly improves the efficacy of radiotherapy or chemotherapy against various human cancers [Citation8–12]. Although the incidence and mortality of cervical carcinoma have decreased considerably in the last few decades, it still remains the fourth most common female malignancy worldwide. Importantly, hyperthermia has been reported to significantly improve the response of cervical cancer to chemotherapy [Citation12], radiotherapy [Citation13–17] and the combination of chemotherapy with radiotherapy [Citation18–20]. Further investigation of the various factors involved in the response of cervical cancer to hyperthermia may lead to better control of the disease.
The early biological investigations conducted in the 1970s–1990s showed that hyperthermia at >42–43 °C directly kills tumour cells and increases cellular radio-sensitivity and chemo-sensitivity [Citation1–3]. Furthermore, it was demonstrated that hyperthermia at >42–43 °C preferentially destroys tumour vasculature relative to normal tissue vasculature, thereby causing necrotic cell death in tumours [Citation4]. Unfortunately, it became increasingly evident in the 1980–1990s that heating deep-seated tumours with external hyperthermia devices increases the tumour temperature only to a range of 38–40 °C [Citation18–23]. Despite the limited heating capability, treatments of various tumours with hyperthermia in combination with radiotherapy or chemotherapy were found to be significantly more effective than radiotherapy or chemotherapy alone, as mentioned above.
Heating tumours to sub-cytotoxic levels or mild temperatures, i.e. <38–41 °C, increases tumour blood flow and leads to an increase in tumour oxygenation in rodent tumours [Citation24] and human tumours [Citation22,Citation25]. Since oxygen plays a critical role in the response of tumours to radiotherapy and certain chemotherapy drugs, it is believed that hyperthermia treatment with external radiofrequency (RF) or microwave heating devices enhances the efficacy of radiotherapy or chemotherapy by increasing tumour blood flow and oxygenation [Citation6,Citation22,Citation24,Citation25]. The purpose of the present study was to investigate whether hyperthermia with modulated electro-hyperthermia (mEHT) could increase the temperature and blood circulation in cervical carcinoma with the ultimate goal of revealing the potential application of mEHT for the control of cervical carcinoma.
Materials and methods
Patient selection
The following inclusion criteria were chosen to allow for the enrolment of a wide range of patients in this study:
Approved malignancy of the cervix
First-time diagnosis of cervical carcinoma
Naïve cases without pre-treatment with chemotherapy and/or radiotherapy
Eastern Cooperative Oncology Group (ECOG) score ≤3
Approval of informed consent in written form
All patients were “Naïve cases without pre-treatment with chemotherapy and/or radiotherapy”. All studies were performed just prior to standard therapy, and all patients were underwent the chemotherapy and radiotherapy.
This study was approved by the Institutional Review Board of Chonbuk National University Hospital (Jeonju, Republic of Korea) and was conducted according to the Declaration of Helsinki for biomedical research involving human subjects and the Guidelines for Good Clinical Practice. A detailed explanation of the study was provided to all subjects and written informed consent was obtained from each participant prior to screening.
Blood flow measurement
Transvaginal ultrasound and colour flow mapping were performed on all patients after receiving informed consent. The ultrasound equipment used was a colour Doppler ultrasound (Voluson P8, GE Ultrasound, Seoul, Korea) with a 5-MHz curvilinear transvaginal transducer. The cervix was scanned carefully and the tumour mass was visualised. The colour Doppler was used to detect tumour blood flow signals. All the measurements were recorded using manual or automatic methods. As absolute flow velocity is dependent on the incidence angle of the colour Doppler beam and an exact angle correction often cannot be made, we used standard angle-independent parameters that indicate flow resistance.
The following parameters were documented for the tumour area:
The ratio of peak systolic velocity to end-diastolic velocity (S/D ratio) of intra-tumoural vessels
The resistance index (RI) of intra-tumoural vessels
The RI in tumour-supplying vessels
The RI was calculated according to the following equation:
RI = (peak systolic velocity – end-diastolic velocity)/peak systolic velocity
Temperature measurement
The temperature was measured with a reusable internal organ temperature prove (Type M3, Philips Medical Systems, Eindhoven, The Netherlands). Before using for each patient, the circular probe (1 cm diameter, 2 mm thick) was calibrated against a NIST (National Institute of Standard and Technology) Traceable Certified mercury in glass thermometer. The accuracy of the probes was approximately ±0.1 °C. For the measurement of temperature, the probe was pre-warmed to 35–36 °C by keeping in sterile saline. The probe was inserted into the cervical os and positioned over the tumour area. The temperature reading stabilised usually in 20–30 s. The procedure for temperature measurement was executed by a gynaecologist. Peri-tumour temperature was measured at 30 min before hyperthermia (baseline), at 30 min and 60 min during the hyperthermia procedure, and at 30 min after hyperthermia. Attempts were made to make the four temperature measurements at the same region of the tumour. In order to avoid the RF-interference on temperature measurement, heating was paused temporarily when the temperature at 30 min time point was measured. The temperature at 60 min heating was determined immediately after the 60 min heating.
Hyperthermia treatment
Regional hyperthermia was performed using a mEHT device (EHY2000, Oncotherm GmbH, Troisdorf, Germany) [Citation26]. We have previously used this device to elucidate the effect of regional heating on the pharmacokinetics of an orally administered drug [Citation27]. Patients were placed in a supine position on a couch, and a 30-cm diameter circular electrode was lightly coupled to the pelvic area. All patients underwent two-dimensional simulation to measure the size of the tumour. The pelvic area was heated at 80 W for the first 10 min, 120 W over the next 10 min and 150 W for the remaining treatment time (40 min).
FDG PET/CT protocol and image analysis (SUVmax and size measurement)
All patients fasted for at least 6 h prior to the intravenous injection of [18F]2-fluoro-2-deoxyglucose (FDG). The blood glucose levels in all patients were below 126 mg/dl. Approximately, 5.5 MBq of FDG per kilogram of body weight was administered intravenously. Scanning was performed at 60 min after FDG administration. Images were obtained from the base of the skull to the proximal thigh using either a Biograph TruePoint 40 positron emission tomography/computed tomography (PET/CT) scanner (Siemens Medical Solutions, Knoxville, TN) or a Biograph 16 PET/CT scanner (Siemens Medical Solutions, Knoxville, TN). A CT scan was obtained first using a continuous spiral technique (120 kVp, 160 mA, 0.5 s rotation time). A PET scan was then acquired in a three-dimensional mode for 2.5 min per bed position. The obtained PET data were reconstructed iteratively using an ordered-subset expectation maximisation algorithm (128 × 128 matrix, 3.27 mm slice thickness, sub-set: 21, iterations: 2).
FDG PET/CT images were analysed at a workstation (Syngo MI Applications, Flexible Display 7.0.7.7; Siemens Medical Solutions, Erlangen, Germany) and the maximum standardised uptake value (SUVmax) was chosen to assess the glucose uptake of the tumours. A volume of interest (VOI) was carefully drawn slightly large enough to include tumours in the axial, coronal and sagittal planes. SUVmax, defined as the SUVmax within the VOI, was calculated as follows: SUVmax = concentration of highest tumour activity in the VOI (MBq/ml)×total body weight (kg)/injected radioactivity (g/MBq). The size of each tumour was measured on a two-dimensional transverse image from the FDG PET/CT scans.
Statistical analysis
SAS software (Version 9.3, SAS Institute Inc., Cary, NC) was used for statistical analysis of the complete population. For the sub-population calculations, non-parametric independent (Mann–Whitney U test) and dependent (Wilcoxon’s signed-rank test) tests were used (WinStat, Fitch Software, Cambridge, MA). The accepted significance level was below p = 0.05.
Results
Baseline characteristics of the patients
The baseline characteristics of the 20 patients treated are shown in . Their median age was 50.5 years old (range, 30–81 years). Patients were classified as stage IIb (10 patients), IIIa (two patients), IIIb (six patients), IVa (one patient) or IVb (one patient). The squamous cell carcinoma (sqcc) to non-squamous cell carcinoma (non-sqcc) ratio was 13:7. The mass nature was exophytic in 14 patients and endophytic in six patients.
Table 1. Baseline characteristics of patients (N = 20) assigned to treatment.
The initial characteristics did not vary significantly with subgrouping by mass size, SUVmax, presentation or pathology (). The RI increased with an increase in the tumour volume and an increase in the intensity of SUVmax, but RI was independent of the stage of cancer. Haemoglobin and haematocrit levels did not show significant differences among subgroups except for the mass size of the tumour. Furthermore, the anaemic levels exerted little effect on the tumour temperature, S/D ratio and RI values.
Table 2. The main values of starting characteristics divided into subgroups to show the cohort properties of the treated population.
Peri-tumoural temperatures and Doppler parameters
The peri-tumour temperatures, the S/D ratios and the RI values are shown in . The changes in these parameters were mostly significant at the end of the 60-min heating procedure. The mean peri-tumour temperature of the 20 patients before heating was 36.7 ± 0.2 °C, and it increased to 37.5 ± 0.5 °C and 38.5 ± 0.8 °C upon heating for 30 and 60 min, respectively, and was 37.1 ± 0.3 °C at 30 min after heating. The S/D ratio was 1.65 ± 0.20 at baseline, 1.40 ± 0.13 and 1.22 ± 0.09 upon heating for 30 and 60 min, respectively, and 1.40 ± 0.16 at 30 min after heating. The RI was 0.40 ± 0.12 before heating, 0.29 ± 0.11 and 0.19 ± 0.06 upon heating for 30 and 60 min, respectively, and 0.30 ± 0.10 at 30 min after heating. shows all patients temperature results.
Figure 1. Peri-tumour temperatures were measured at 30 min before hyperthermia (baseline), at 30 min and 60 min during the hyperthermia procedure, and at 30 min after hyperthermia. The peri-tumour temperatures of all patients are shown.
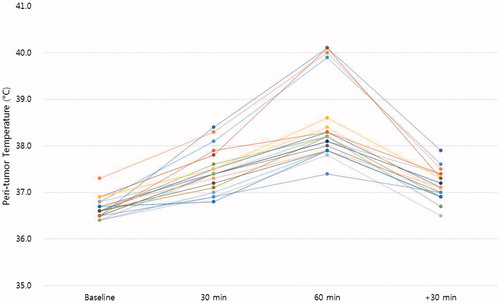
Table 3. Changes in the peri-tumour temperature, S/D ratio and RI index in cervical tumours.
The marked declines in the S/D ratio and RI clearly demonstrated that the blood flow into the cervical tumours markedly increased when the tumours were heated with the mEHT unit. shows examples of changes in the RI and S/D ratio in cervical cancer during heating in a patient.
Measuring data grouped by pathology, tumour presentation and SUVmax
Regrouping the measurement data by the pathology of tumours (sqcc vs. non-sqcc) is shown in . There were no significant differences in the S/D ratio or RI between the subgroups.
Table 4. The peri-tumour temperatures, S/D ratios and RI indexes in squamous cell carcinoma (sqcc) and non-squamous cell carcinoma (non-sqcc).
Discussion
In the present study, treating human cervical cancer with mEHT significantly increased the peri-tumour temperature and tumour blood flow (Doppler flow). These increases were independent of various cervical tumour properties suggesting that the changes in temperature and blood flow we observed in the present 20-patient trial are general phenomena that occur in human cervical cancer treated with mEHT.
The mean peri-tumour temperature increased from 36.7 ± 0.5 °C before heating to 38.5 ± 0.8 °C after heating with mEHT for 1 h (). Given the possibility that there is less blood perfusion and thus less perfusion-mediated heat dissipation in the inner region of tumours than in the periphery of tumours, it would be reasonable to expect that during heating with mEHT, the temperature inside the cervical tumour mass was higher than the measured peri-tumour temperature, i.e. 38.5 °C.
The S/D ratio decreased from the average baseline value of 1.65 ± 0.20 to 1.22 ± 0.09 upon heating for 60 min, and the RI index deceased from the average baseline value of 0.40 ± 0.12 to 0.19 ± 0.06 upon heating for 60 min (). These declines in the S/D ratio and RI index demonstrated that the flow resistance in blood vessels significantly declined, implying that blood flow into the tumours significantly increased by the heating. Unfortunately, however, these changes alone precluded us from estimating the extent of increased blood perfusion in the whole tumour mass.
Another interesting observation in the present study was that the increase in peri-tumour temperature, excluding the cooling period, was negatively related to the RI and S/D by R2 ≈ 0.999 and R2 ≈ 0.972, respectively (), and positively related to blood flow. Such a significant correlation between the means of RI and S/D suggested that only one of them could be used to estimate the changes in tumour temperature or blood flow. The feasibility of non-invasively estimating tumour temperature from the Doppler-based parameters remains to be investigated. As shown in , the peri-tumour temperatures of four patients were noticeably higher compared to those of other patients. The RI and S/D of those four cases were found to be slightly lower than those of other patients although, the differences were not statistically significant.
Figure 3. The increase in peri-tumour temperature, excluding the cooling period, was negatively related to the RI and S/D by R2 ≈ 0.999 (A) and R2 ≈ 0.972 (B), respectively, and positively related to blood flow.
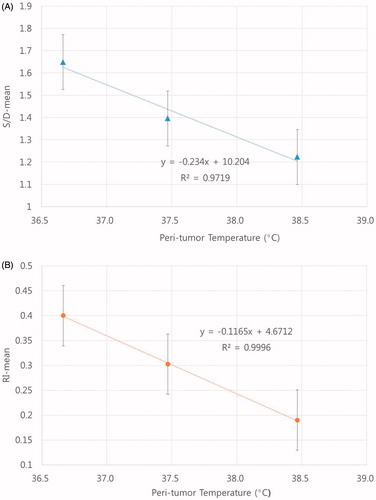
In previous clinical trials on the hyperthermia treatment of human cervical cancer, heating with external RF or microwave heating devices increased tumour temperature only to a sub-cytotoxic range of 38–40 °C [Citation18,Citation23]. Despite such moderate increases in temperature, hyperthermia has been shown to significantly improve the efficacy of radiotherapy or chemotherapy in controlling human cervical cancer. Several pre-clinical and clinical studies indicated that hyperthermia at a mild, sub-cytotoxic temperature enhanced the efficacy of radiotherapy by increasing tumour oxygenation via increasing tumour blood flow. Song et al. [Citation24] reported that mild temperature hyperthermia at 38–41 °C increased blood flow, thereby increasing oxygenation in experimental rodent tumours. Thrall et al. [Citation28] and other investigators [Citation29,Citation30] suggested that mild temperature hyperthermia has the potential to improve tumour oxygenation and increase tumour blood flow in spontaneous canine soft tissue sarcoma models. A similar increase in oxygenation by mild temperature hyperthermia has subsequently been observed in various human tumours [Citation22,Citation25]. Brizel et al. [Citation22] reported that heating human soft tissue sarcoma to T90 of 39.9 °C led to an increase in tumour oxygenation and that the magnitude of improvement in tumour oxygenation was positively correlated with the amount of necrosis in the tumours caused by subsequent radiation therapy. The authors further concluded that such an increase in tumour oxygenation by the mild heating was due to reduced oxygen demand resulting from the cytotoxic effect of hyperthermia and/or an increase in tumour blood flow. It is of note that reduction of oxygen demand by heating would occur only when the heat-induced cell death and/or damage are significant, and that heating at sub-cytotoxic temperatures, i.e. <41 °C, would increase rather than decrease the oxygen demand by increasing cellular metabolisms. It is therefore highly likely that the increase in tumour oxygenation by mild-temperature is due mainly to an increase in tumour blood flow.
It is relevant that Vaupel and Kelleher [Citation31] reported that hyperthermia of uterine cervix cancers may change the tumour blood flow in non-predictable direction and concluded that clinical studies are urgently needed to shed light on the possible impact of blood flow in the treatment of cervical cancer with hyperthermia.
In conclusion, mEHT increases the peri-tumour temperature and blood flow in human cervical tumours to the levels reported to improve the response of various human tumours to radiotherapy and chemotherapy alone or combined. Clinical trials are warranted to further elucidate the potential usefulness of mEHT in improving the efficacy of radiotherapy against human cervical cancer.
Disclosure statement
The authors have no conflicts of interest to declare.
Additional information
Funding
References
- Dewey WC. (1994). Arrhenius relationships from the molecule and cell to the clinic. Int J Hyperthermia 10:457–83.
- Hall EJ, Roizin-Towle L. (1984). Biological effects of heat. Cancer Res 44:4708s–13s.
- Hahn GM. (1982). Hyperthermia and cancer. New York, NY: Plenum Press.
- Song CW. (1984). Effect of local hyperthermia on blood flow and microenvironment: a review. Cancer Res 44:4721s–30s.
- Dahal O. (1994). Mechanisms of thermal enhancement of chemotherapeutic cytotoxicity. In: Urano M, Douple E, eds. Hyperthermia and oncology. Utrecht: VSP, 9–28.
- Dewhirst MW, Vujaskovic Z, Jones E, et al. (2005). Re-setting the biologic rationale for thermal therapy. Int J Hyperthermia 21:779–90.
- van den Tempel N, Horsman MR, Kanaar R. (2016). Improving efficacy of hyperthermia in oncology by exploiting biological mechanisms. Int J Hyperthermia 32:446–54.
- Valdagni R, Amichetti M. (1993). Report of long-term follow-up in a randomized trial comparing radiation therapy and radiation therapy plus hyperthermia to metastatic lymph nodes in stage IV head and neck patients. Int J Radiat Oncol Biol Phys 28:163–9.
- Overgaard J, Gonzalez Gonzalez D, Hulshof MC, et al. (1995). Randomised trial of hyperthermia as adjuvant to radiotherapy for recurrent or metastatic malignant melanoma. European Society for Hyperthermic Oncology. Lancet 345:540–3.
- Wust P, Hildebrandt B, Sreenivasa G, et al. (2002). Hyperthermia in combined treatment of cancer. Lancet Oncol 3:487–97.
- Datta NR, Ordonez SG, Gaipl US, et al. (2015). Local hyperthermia combined with radiotherapy and-/or chemotherapy: recent advances and promises for the future. Cancer Treat Rev 41:742–53.
- Issels R. (1999). Hyperthermia combined with chemotherapy – biological rationale, clinical application, and treatment results. Oncol Res Treat 22:374–81.
- Datta NR, Bose AK, Kapoor HK. (1987). Thermoradiotherapy in the management of carcinoma cervix (stage IIIB): a controlled clinical study. Indian Med Gaz 121:68–71.
- van der Zee J, Gonzalez DG, van Rhoon GC, et al. (2000). Comparison of radiotherapy alone with radiotherapy plus hyperthermia in locally advanced pelvic tumours: a prospective, randomised, multicentre trial. Dutch Deep Hyperthermia Group. Lancet 355:1119–25.
- Harima Y, Nagata K, Harima K, et al. (2001). A randomized clinical trial of radiation therapy versus thermoradiotherapy in stage IIIB cervical carcinoma. Int J Hyperthermia 17:97–105.
- van der Zee J, Gonzalez GD. (2002). The Dutch deep hyperthermia trial: results in cervical cancer. Int J Hyperthermia 18:1–12.
- Lutgens LC, Koper PC, Jobsen JJ, et al. (2016). Radiation therapy combined with hyperthermia versus cisplatin for locally advanced cervical cancer: results of the randomized RADCHOC trial. Radiother Oncol 120:378–82.
- Jones EL, Samulski TV, Dewhirst MW, et al. (2003). A pilot phase II trial of concurrent radiotherapy, chemotherapy, and hyperthermia for locally advanced cervical carcinoma. Cancer 98:277–82.
- Westermann AM, Jones EL, Schem BC, et al. (2005). First results of triple-modality treatment combining radiotherapy, chemotherapy, and hyperthermia for the treatment of patients with stage IIB, III, and IVA cervical carcinoma. Cancer 104:763–70.
- Sreenivasa G, Hildebrandt B, Kummel S, et al. (2006). Radiochemotherapy combined with regional pelvic hyperthermia induces high response and resectability rates in patients with nonresectable cervical cancer > or = FIGO IIB “bulky”. Int J Radiat Oncol Biol Phys 66:1159–67.
- Dewhirst MW, Griffin TW, Smith AR, et al. (1993). Intersociety council on radiation oncology essay on the introduction of new medical treatments into practice. J Natl Cancer Inst 85:951–7.
- Brizel DM, Scully SP, Harrelson JM, et al. (1996). Radiation therapy and hyperthermia improve the oxygenation of human soft tissue sarcomas. Cancer Res 56:5347–50.
- Wust P, Gellermann J, Harder C, et al. (1998). Rationale for using invasive thermometry for regional hyperthermia of pelvic tumors. Int J Radiat Oncol Biol Phys 41:1129–37.
- Song CW, Shakil A, Osborn JL, et al. (1996). Tumour oxygenation is increased by hyperthermia at mild temperatures. Int J Hyperthermia 12:367–73.
- Vujaskovic Z, Song CW. (2004). Physiological mechanisms underlying heat-induced radiosensitization. Int J Hyperthermia 20:163–74.
- Andocs G, Szasz O, Szasz A. (2009). Oncothermia treatment of cancer: from the laboratory to clinic. Electromagn Biol Med 28:148–65.
- Lee SY, Kim MG. (2015). The effect of modulated electro-hyperthermia on the pharmacolinetic properties of nefopam in healthy volunteers: a randomised, single-dose, crossover open-label study. Int J Hyperthermia 31:869–74.
- Thrall DE, Larue SM, Pruitt AF, Dewhirst MW. (2006). Changes in tumour oxygenation during fractionated hyperthermia and radiation therapy in spontaneous canine sarcomas. Int J Hyperthermia 22:365–73.
- Vujaskovic Z, Poulson JM, Dewhrist MW, et al. (2000). Temperature-dependent changes in physiologic parameters of spontaneous canine soft tissue sarcomas after combined radiotherapy and hyperthermia treatment. Int J Radiat Oncol Biol Phys 46:179–85.
- Gillette SM, Dewhirst MW, et al. (1992). Response of canine soft tissue sarcomas to radiation or radiation plus hyperthermia: a randomized phase II study. Int J Hyperthermia 8:309–20.
- Vaupel PW, Kelleher DK. (2012). Blood flow and associated pathophysiology of uterine cervix cancers; characterisation and relevance for localized hyperthermia. Int J Hyperthermia 28:518–27.