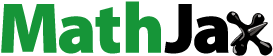
Abstract
The poor penetration of anti-fungal agents into the cornea through the intact epithelium layer makes it difficult to treat acute fungal corneal infections. Herein, we developed Amphotret (amphotericin B) antifungal drug contained polycaprolactone-Fe3O4 (PCL-FO) magnetic nanofibers (MNFs) using the electrospinning technique. These MNFs generate heat in the presence of AC magnetic field (AMF) and release drug upon heating. MNFs were compatible with human mesenchymal stem cells (hMSCs) and HeLa cells, which exhibited unaltered proliferation, ruling out any toxicity from the systems. Hyperthermia induced via MNFs from 42 °C to 50 °C compromised the viability of Candida albicans cells. Further, the efficacy of the systems was increased in the presence of both heat and drug simultaneously in vitro, leading to near 100% loss in viability of C. albicans cells at 50 °C with simultaneous drug release from MNFs. Thus, we propose magnetic hyperthermia as adjunctive therapy for fungal keratitis.
1. Introduction
Keratitis is an inflammation in the cornea of the eye due to the invasion of microorganisms, i.e. bacterial, viral or fungal cells [Citation1–3]. The uncontrolled proliferation of microorganisms penetrates and extends to all the ocular tissues which can eventually lead to complete vision loss [Citation4,Citation5]. Among all infections from different microorganisms, fungal keratitis is still a major challenge to diagnose and treat. Most cases of the fungal keratitis reported in the developing countries, for example, India, Ghana, China. Gopinathan et al., reported that the patients with agriculture-based activities were at 1.33 times (95% CI 1.16–1.51) greater risk of developing microbial keratitis and patients with ocular trauma were 5.33 times (95% CI 6.41–6.44) more likely to develop microbial keratitis [Citation6]. It is reported that the fungal keratitis treated with Natamycin 5% monotherapy for large ulcer size and infection with Aspergillus is not satisfactory [Citation4]. Additionally, Srinivasan et al. [Citation7] reported that the treatment of infective keratitis is very expensive within the existing healthcare system. Antifungal agents are known to be an effective treatment for fungal keratitis. However, the resistance against antifungal drugs, corneal anatomy, and low vascularization make the effect of therapies largely variable and often unsuccessful, exhibiting the infectious keratitis as a real ocular urgency [Citation1,Citation8]. Further, the chances of failure of the treatment are more after the formation of biofilm cell communities as they are impermeable to antifungal drugs unlike planktonic cells [Citation9]. Thus, for acute fungal infection therapeutic keratoplasty is required for the treatment despite timely medical intervention [Citation10–13].
Intrastromal injection of anti-fungal like Voriconazole and 5% Natamycin have been tried as an adjunct to topical Natamycin therapy. Sharma et al. showed intrastromal injections are not offering any beneficial effect over topical therapy in recalcitrant fungal keratitis [Citation14]. Collagen cross-linking is advocated in the management of fungal keratitis as an adjunctive therapy since corneal strengthening may inhibit corneal melting and prevent perforation but results of several trials have been contradictory [Citation15]. In vitro studies found that collagen cross-linking with riboflavin to be effective in reducing the viability of fungal cells. However, clinical experience is not supportive, and cases of corneal perforation are reported in deep-seated infections following collagen cross-linking.
The use of external heat therapy for the treatment of nominal ailments is well established decades ago. With the advancement in technology, the delivery of heat energy locally with minimal invasion is possible. Local hyperthermia can be achieved by radio frequency, laser ablation, microwaves, ultrasound waves, and magnetic field. The thermal threshold for tissue damage is dependent on the type of tissue, temperature, duration of exposure and the technique of heat supply [Citation3]. It is reported that cumulative equivalent minutes (CEM43), the metric of thermal tissue damage at 43 °C, CEM43 = 2.7 min can damage cornea when hyperthermia achieved by ultrasound, whereas diode laser heating did not show any damage in the cornea at CEM43 up to 1.26*103 min [Citation3]. Kaluorachi et al. have demonstrated Aspergillus fumigatus hyphal damage caused by noninvasive radiofrequency field induced hyperthermia (RFHT) [Citation16]. RFHT exposure resulted in damage of 70% of the hyphae over 5 min and a 10 min exposure to RFHT at 48.09° caused extreme hyphal damage (>90%). Photo dynamic therapy (PDT) involves activation of a photosensitizing agent by light ranging from Ultra-violet-A (UVA) to near-infrared wavelength [Citation17]. The photosensitizer reaches an excited state that undergoes a reaction with ambient oxygen to create a reactive oxygen species (ROS). These ROS then react with intracellular components and induce cell inactivation and death. Arboleda et al. [Citation15] reported Rose Bengal mediated PDT on fungal isolates which are common causes of fungal keratitis. 0.1% Rose Bengal mediated PDT successfully inhibited the growth of all fungal isolates (Fusarium solani, Aspergillus fumigatus, Candida albicans) in the irradiated area. Anecdotal case reports of successful treatment by this modality are reported in the literature.
Magnetic hyperthermia (MH) is a cutting-edge technology, still in its infancy, based on the principle of delivering heat energy (42–50 °C) locally to kill infected cells using a magnetic material with the application of external alternating magnetic field [Citation18–22]. In order to achieve localized heating, various magnetic nanoparticles (MNPs) have been developed so far [Citation23,Citation24]. However, there are several challenges associated if NPs are to be considered for corneal infection treatment. The first challenge is the localization of NPs at the damaged site: for the cancer treatment, the tumor can be targeted by binding of specific biomolecules to NPs, however, if unspecified NPs are used for corneal treatment then normal cells will be damaged by unwanted cellular uptake [Citation25]. Second, monodispersity and stability: this is a major hurdle of NPs due to the high surface energy they possess, NPs have the tendency to agglomerate and lead to non-uniform heating. Third, removal of accumulated NPs after treatment: the accumulated NPs in the body over a long term shows toxic effects which leads to other physiological problems. Finally, higher concentrations of NPs are required to increase temperatures. To overcome challenges associated with NPs, herein, the magnetic nanofibers (MNFs) were fabricated to deliver heat energy as well as antifungal drug upon heating to treat corneal fungal infection efficiently. The candidacy of the MNFs was checked in vitro against Candida albicans cells which are virulent fungal pathogens and involved in infections, for instance, of the skin, oral cavity and esophagus, and vascular system of human [Citation26]. The magnetic fibrous mat is flexible and soft. It can easily be cut in any shape and size according to requirement and can be placed close to the infected cornea. Unlike NPs, MNFs will generate heat outside the cells and can be easily removed after the treatment. We believe such effective properties of the system make it a suitable candidate to be considered for the treatment of corneal infection.
2. Materials and methods
2.1. Materials
Polycaprolactone (PCL, Mw = 80,000), Fe3O4 nanoparticles (FO NPs spherical 50–100 nm diameter), fetal bovine serum (FBS), 0.25% trypsin-EDTA solution, 3(4,5-dimethylthiazol-2-yl)-2,5-diphenyl tetrazolium bromide (MTT) and antibiotic-antimycotic solution were obtained from Sigma Aldrich, USA. 2,2,2-Trifluoroethanol (TFE) was obtained from Spectrochem chemicals, Mumbai, India. Formaldehyde and dimethyl sulfoxide (DMSO) were bought from Fischer Scientific, USA and Dulbecco’s Modified Eagle’s Medium (DMEM) from HiMedia, France. ActinGreen 488 ready probes reagent and Hoechst 33342, KnockOut™ DMEM, Recombinant Human FGFb, Mesenchymal stem cell FBS were obtained from ThermoFisher Scientific, USA. Human mesenchymal stem cells (hMSCs) were obtained from Lonza, Swiss. Amphotericin B (Amphotret) was bought from Bharat Serum and Vaccines LMT, India.
2.2. Methods
2.2.1. Fabrication of magnetic nanofibers
The MNFs were fabricated using electrospinning technique (E spin: Physics Equipment, Chennai, India) [Citation27]. For electrospinning, FO NPs were mixed using ultrasonicator in the PCL, 90% TFE and 10% DMSO solution prior to spinning. The distance between the syringe tip and the collector was 7 cm and the applied voltage was kept at 20 kV. In the case of drug contained fibers, 0.02% of Amphotret (amphotericin) was added in the PCL-FO solution before electrospinning.
2.2.2. Characterization of magnetic nanofibers
Field emission scanning electron microscopy (FE SEM, Carl Zeiss Ultra55 FESEM, Germany) was used to characterize the fibrous morphology before and after AMF exposure. MNFs were coated with a few nm of gold by sputter coating for 38 s before SEM characterization. The induction heating and drug release from the fibers in 1× PBS were confirmed using Ambrell Easy Heat solution at an alternating current of 3.6 kA/m and frequency 236 kHz and the change in temperature was measured using alcohol thermometer. For drug release study, 10 mg MNFs were suspended in 1× PBS and optical density (Tecan Infinite® M 1000 PRO) of the supernatant solution was measured at 407 nm before and after AMF exposure.
2.2.3. Cumulative equivalent minutes calculation
The standard metric to measure the thermal damage to tissue is calculated as cumulative equivalent minutes at 43 °C (CEM43).
where t is the exposure time (in minutes), T is the final temperature (in degrees Celsius), R = 0.5 for temperatures >43 °C, and R = 0.25 for temperatures <43 °C [Citation3,Citation16].
2.2.4. Cell culture
The MNFs were sterilized using 70% ethanol followed by UV exposure for 10 min. HeLa cells (ATCC) were grown in 25 cm2 culture flasks with DMEM, supplemented with 10% FBS and 1% antibiotic solution. Similarly, hMSCs (Lonza, Swiss) were maintained in the KnockOut™ DMEM, 5 ng/ml FGFb, 15% Mesenchymal stem cell FBS and 1% antibiotic solution. For trypsinization of the cells, trypsin (0.25% trypsin–EDTA solution) was used. The number of cells was counted using a hemocytometer (Improved Neubauer cell counter). The seeded cells were incubated at 37 °C in CO2 incubator (New Brunswick™ Galaxy® 1705) with 5% CO2 and 90% humidity. Tissue culture polystyrene (TCPS) well plates were used as a control in all the cell culture experiments.
2.2.4.1. Cell morphology analysis
The cellular morphology and cell-to-cell contacts after incubation of cells on the MNFs were analyzed by fluorescence microscopy (InCell Analyzer 6000, GE health care life science, USA) and SEM. 2 × 104 cells were seeded on the fibers for 24 h in the case of HeLa cells and 48 h in the case of hMSCs, Post incubation, cells were fixed with 3.7% formaldehyde in 1× PBS for 20 min. For fluorescence microscopy, cells were permeabilized with 0.1% Triton-X 100 in 1× PBS. Subsequently, cells were blocked with 5% FBS in 1 × PBS. ActinGreen 488 ready probes reagent and Hoechst 33258 dyes were added to stain actin filament and nucleus of the cell, respectively. For SEM, after fixing, cells were dehydrated with serial dilutions of ethanol for 10 min each and followed by gold coating.
2.2.4.2. Cell viability assessment
The metabolic activity (viability) of the hMSCs and HeLa cells after incubation with MNFs was quantified by MTT assay. Metabolically active cells form formazan crystals after reacting with MTT salt. For MTT assay, 2 × 104 cells were seeded on the sterilized MNFs and incubated for 24/48 h. After incubation, 0.5 mg/ml of MTT solution was added in each well and incubated for a period of 2 h. The formed formazan crystals were solubilized in DMSO and optical density was recorded at 570 nm using a plate reader (Tecan Infinite® M 1000 PRO). The MTT assay was repeated five times. The viability of HeLa cells immediately after 10 min of AC magnetic field (AMF) exposure with MNFs was also quantified by MTT assay.
2.2.5. Colony forming unit assay and live-dead staining of Candida albicans
Candida albicans, wild-type strain SC5314, cells were grown overnight in YPD (yeast extract 1%, peptone 2%, dextrose 2%) and subsequently diluted to 0.1 OD/ml (∼106 cells/ml) in phosphate buffer saline (PBS). To 1 ml of this cell suspension 10 mg of MNFs was added. This was followed by exposure of the vial containing cells and MNFs to an external AMF (). Post-treatment, the cell suspension was diluted and plated on to Sabouraud dextrose agar plates and incubated for 48 h before counting. The limit of detection for this assay is a single viable C. albicans cell.
For live-dead staining, C. albicans cells post-treatment to an external AMF were pelleted and resuspended in a solution containing 5 μg/ml of Propidium Iodide (PI, HiMedia). After incubation of 20 min cells were imaged using a fluorescence microscope. The images were captured using a Zeiss Axio 7 microscope equipped with a pco edge 4.2 camera.
2.2.6. Determining minimum inhibitory concentration (MIC50) of amphotericin B
The MIC50 of Amphotericin B was determined according to the CLSI standards [Citation28]. In brief, C. albicans cells were grown overnight in YPD. Cells were counted and diluted to ∼104 cells/ml in RPMI 1640 containing 2% glucose and buffered with 3-(N-morpholino) propanesulfonic acid (MOPS), pH 7.0. 100 μl of this cell suspension was added to microdilution plates containing dilution of Amphotericin B (0.03125μg/ml to 2 μg/ml) in the prepared RPMI medium. The microdilution plates were incubated at 37 °C for 48 h, mixed and the optical density was determined at 600 nm.
2.3. Statistical analysis
All the experimental data were expressed as mean ± standard error (SE) and were analyzed by ANOVA (SPSS 16.0) for the calculation of significance level of the experimental data. The differences were considered statistically significant at p ≤ .05. The significance of cfu differences was assessed by two-tailed unpaired t-tests.
3. Results
3.1. Characterization of MNFs
The polycaprolactone-Fe3O4 (PCL-FO) based Amphotret (amphotericin) antifungal drug contained MNFs were fabricated using the electrospinning technique. shows the representative higher magnification SEM image of MNFs. It is evident from SEM that the diameter of fibers is in the range of 200–1000 nm and the FO MNPs (50–100 nm) are decorated on the PCL fibrous matrix. The weight of the fibrous mat did not change over time when incubated in PBS for 48 h, which confirmed the FO NPs are tightly bound with the fibrous matrix. To further confirm the binding of NPs to PCL matrix, SEM of the fibrous mat was done after AMF exposure for 15 min. It is evident from the , MNFs lost its morphology after generating heat, however, FO NPs still embedded in the PCL matrix (). Further, when the 10 mg of MNFs subjected to AMF (3.6 kA/m, 236 kHz) for 15 min, the surrounding media temperature was increased by 30 °C (). The standard 0.15% (W/V) Amphotericin B is used clinically for the treatment of fungal keratitis. In order to achieve similar released drug concentration, 2 µg/ml drug was incorporated into the fibers and interestingly, fibers released around 80% of the drug upon heating. In the absence of AMF, at room temperature there was only 0.6 µg/ml drug released from the fibers, however, in the presence of AMF fibers released significantly higher 80% of the incorporated drug 1.5 µg/ml at 47 °C. The amount of drug released in the presence of heat is around 2-fold to the drug released in the absence of heat in 15 min at 47 °C ().
3.2. Cytocompatibility of MNFs
The in vitro cytocompatibility of the MNFs were tested with hMSCs and HeLa cells. hMSCs can be differentiated in the corneal epithelial cells and they support corneal repair [Citation29–31]. Similarly, HeLa cells are malleable and can adapt to almost any condition, proving to be robust cellular models for in vitro testing. The cytocompatibility of MNFs was tested by assessment of viability and morphology of HeLa cells and hMSCs incubated with MNFs for durations of their doubling time of 24 and 48 h, respectively. There was no difference in the morphology of HeLa cells () and hMSCs () observed when compared to cells grown on TCPS. Interestingly, cells spread on the MNFs () and made multiple contacts with fibers. The viability of the cells was comparable to control (TCPS) after 24 h of growth in the case of HeLa cells and after 48 h in the case of hMSCs ().
3.3. Toxicity of drug and heat to cells
The effect of the application of AMF with MNFs on C. albicans cells was scored using the colony forming unit (CFU) assay. The limit of detection for the CFU assay is a single cell. This test determines the ability of C. albicans cells to divide and form individual colonies post treatment and are scored relative to the untreated controls. To determine the minimum inhibitory concentration (MIC), cells were incubated with varying concentrations of the drug for 72 h and MIC50 was determined to be 0.0625 µg/ml (). MNFs and drug contained MNFs when incubated for 15 min with cells in the absence of AMF, did not lead to a significant change in the viability. Increasing the temperature between 42 °C and 50 °C through the application of AMF resulted in reduced viability of C. albicans cells, from ∼80% to 35%. The cfu assay confirms the viability of C. albicans cells after exposure of AMF with MNFs (without drug) is significantly lower (p = .0049 at 47 °C and p = .0006 at 50 °C) when compared to cfu of cells without AMF and without the drug. The cfu count of C. albicans was further significantly reduced ∼50% to 0% (p < .0001 at 47 and 50 °C) in the simultaneous presence of both drug and increasing heat (). The drug released from the fibers in the absence of heat was not enough (0.6 µg/ml) to kill cells in the 15 min duration, however, when temperature increased, the amount of drug released from the fibers (1.5 µg/ml, ) is also increased and resulted in increased cell death. A similar effect can be seen on the HeLa cells when subjected to heat, generated by MNFs in the presence of AMF in the similar condition (). However, the reduction in the cell viability below 43 °C is not significant when compared to control. Also, previously, we have reported that above 47 °C 90% of HeLa cells can be killed in the 10 min duration in vitro [Citation27].
Figure 4. Effect of AC magnetic field (AMF) and drug release on C. albicans cells. (a) Minimum inhibitory concentration (MIC) curve (b) viability of C. albicans cells. Samples were subjected to AMF for 15 min. Without AMF/without drug, the cfu counts are statistically significant when compared to with AMF/without drug (p = .0049 at 47 °C and p = .0006 at 50 °C). Also, without AMF/with drug cfu counts are statistically significant when compared to with AMF/with drug (p = .0001 at 47 °C and 50 °C), (c) HeLa cell viability of AMF exposure. Error bar represents mean ± SE. *Shows the significant difference at p ≤ .05 w.r.t. control and (d) vital staining of C. albicans cells by propidium iodide (PI). Control and test samples were stained with PI to score for live–dead C. albicans cells. Scale bar 6 μm.
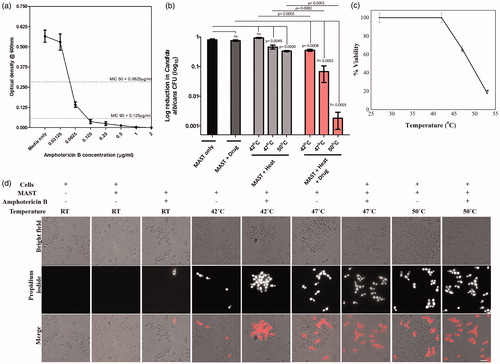
The C. albicans cells were stained with PI in control and test samples to observe the loss in vitality of cells after treatment. Vital cells do not take up the PI stain, since PI is excluded from entering a living cell, but can stain dead cells. The proportion of cells that had taken up PI staining increased with the temperature of hyperthermia treatment. While, in cells incubated with MNFs only without hyperthermia, fewer PI-stained cells were observed. Indicating hyperthermia treatment even in the absence of drug rendered cells inviable (). Cells incubated with MNFs without AMF induced hyperthermia were largely vital. Simultaneous treatment of cells with MNFs contained drug and exposure to AMF significantly reduced the viability. This loss in viability was close to 100% at 50 °C.
4. Discussion
The failure of fungal keratitis treatment despite proper antifungal therapy is due to the poor ocular penetration of medications, poor drug bio-availability, narrow spectrum of anti-fungal agents or inability to break the fungal biofilm [Citation32]. Novel treatment modalities, for example, intrastromal injection of anti-fungal, corneal collagen cross-linking and Rose Bengal/Riboflavin photodynamic therapy for inhibition of fungal keratitis isolates have been tried, but none of these therapies are routinely followed in clinics. In the present work, the MNFs were fabricated to kill fungal cells in a short period of time with heat and delivery of antifungal drug locally upon the application of the AMF.
It is reported that the heat treatment up to 60 °C has been used to reshape the cornea to remove myopia or hyperopia permanently [Citation33]. The heating of the cornea (thermokeratoplasty) is achieved with conducted heated probes (130 °C), however, this technique has many issues, for example, maintain the temperature of the probe, uniform delivery of heat energy and chances to damage healthy cells were high [Citation33,Citation34]. Herein, the MNFs were fabricated to deliver localized heat energy without harming healthy cells. These MNFs were fabricated using electrospinning technique from FO (Fe3O4) NPs suspended PCL solution. All the solvents from PCL-FO solution evaporate during the electrospinning process in the presence of high external electric field which allows NPs to distribute and tightly bind to the PCL matrix uniformly. These MNFs are continuous fibers with the diameter in the range of 200–1000 nm. The generation of heat in the embedded FO (50–100nm) NPs in MNFs under AMF is governed by a hysteresis loss mechanism [Citation23].
In order to enhance the efficacy of the system, the antifungal drug Amphotericin B was incorporated in the MNFs during fabrication. The loaded drug from the fibers released through diffusion when submerged in the media. Interestingly, the increased temperature in the presence of AMF enhanced the rate of diffusion and resulted in increased drug release from the MNFs [Citation35]. The local delivery of heat and drug, without compromising drug activity at a higher temperature (), enhance the efficacy of the systems as well as reduce the side effect of the treatment. However, for the localized delivery of the heat and drug, it is essential that the system should not affect or create any toxic environment to its surroundings. To rule out the toxicity of the system, the cytocompatibility of MNFs was confirmed with the robust model HeLa cells and multilineage hMSCs which can be differentiated in the corneal epithelial cells [Citation31]. It was observed that the MNFs were compatible with HeLa cells as well as hMSCs and no significant difference in the cell viability was observed when compared to the control (). Although magnetic fibrous mats are cytocompatible to HeLa and hMSCs cells, there is still a need to study further the compatibility of the fibrous mat with corneal epithelial cells in the presence/absence of AMF for suitability of the system for the treatment of fungal keratitis.
Most fungal corneal infections are due to the invasion of C. albicans, Aspergillus and Fusarium cells. C. albicans is a pathogenic fungus and involved in infecting regions such as, skin, oral cavity esophagus, and causing systemic infections in humans [Citation26]. Herein, the survival of C. albicans after exposure of heat and drug for 15 min at in vitro was investigated using cytocompatible MNFs. In the current study, the combination of heat (42 °C) and the antifungal drug Amphotericin B, killed 70% of C. albicans. At 47 °C along with the drug, 90% of C. albicans were killed and at 50 °C almost all C. albicans cells were rendered inviable in the presence of the drug. Since the percentage of viable cells decreases with increasing temperature () in the presence of drug compared to without drug, which further confirmed the fungicidal activity of the drug Amphotericin B did not alter at higher temperatures [Citation36]. Although drug release from the fibers was greater than the MIC, we suspect the short duration of exposure, 15 min vs. 72 h, to account for the lower rate of cell death in non-heated condition. It has been shown to require durations greater than 10 h with amphotericin B to reduce viability in C. albicans [Citation37]. With this result, we are contemplating the use of MH at a temperature between 40 °C and 47 °C for fungal corneal infection as adjunctive therapy to reduce the extent of tissue damage observed at higher temperatures. Laser thermokeratoplasty experience has suggested that beyond a temperature of 55 °C, permanent changes in corneal collagen can occur [Citation36]. Protein denaturation is one of the main mechanisms for cell death; which is observed at a temperature greater than 40 °C. Some of the effects of protein denaturation are damage to the DNA repair system and alterations to the cell membrane [Citation20,Citation38–40].
The effects of heat on fungi depend on many factors, including the genus, species, and strain of the fungus, the amount of available water, kinds of nutrients, and many other environmental factors. Temperature is also a crucial factor. Most of the research on temperature relationships for the fungi has been done in the food industry where heat is commonly used to prevent fungal growth. As temperatures progress above the optimum temperature, the chemical reactions occur less efficiently, and growth slows. Eventually, the temperature can reach a point wherein growth stops, and cell components are damaged by the heat. Enzymes are proteins that change structurally when heated to their limit of tolerance. Likewise, membranes, which contain lipids, change in structure, and their function of protecting and regulating the internal environment of the cell becomes compromised. Thus, hyperthermia compromises cellular functions while the simultaneous release of drug from the same medium, here MNFs, may be responsible for the observed synergistic effect on the viability of C. albicans.
The severity of thermal damage is represented by CEM43 at 43 °C [Citation3]. It is reported that thermal damage in rabbit cornea with ultrasound heating at CEM43 = 21.3 min resulted in mild and acute effects in the cornea which results in epithelial cell edema, collagen disorganization, severe stromal edema, intrastromal vacuole formation, plump keratocyte nuclei, and endothelial cell detachment). When CEM43 increased to 2.2 × 104 min, the severe corneal damage was observed immediately and 7 days after treatment [Citation41]. In the present study, it is observed that at lower CEM43 (5.6 min) the cell death was very less, however, with same CEM43 in the presence of drug the cell damage was higher (. At CEM43 = 39.8 min, in the presence of the drug, around 90% of cell death was achieved.
While utilizing Amphotericin B containing MNFs with AMF exhibits promising results as described in this study, this technique is yet in early stage and there is a need of further investigation to fully standardize and establish the concept. With the current preliminary study, we have established the proof of concept and believe the localized delivery of heat, as well as drug to treat corneal infection, can be possible using MNFs as shown in the schematic (). The MNFs mat can be placed on the damaged cornea in the patient’s eye and the required frequency of the magnetic field can be applied using AMF generator. The increased temperature will be measured, and the fibrous mat will be removed from the eye after exposure. It is believed that this technique will not affect healthy cells because of the rapid dissipation of heat through cornea [Citation33,Citation34]. The MNFs mat overcomes all the issues associated with NPs as discussed before, i.e. localization, monodispersity, concentration and removal of the NPs after treatment. While treating corneal keratitis using MNFs is a promising future therapy, we also foresee this system for more general use to treat external fungal infections.
5. Conclusions
To conclude, the magnetic field induced hyperthermia can be an effective adjunctive therapy for the treatment of fungal keratitis using PCL-Fe3O4 MNFs. The efficacy of the system can be enhanced further with the incorporation of the antifungal drug in the MNFs. Our study shows a dramatic loss in the viability of a planktonic pathogen (Candida albicans) in the presence of AMF using drug contained cytocompatible magnetic nanofibrous mat. Yet, there is still a room to investigate this system with better ex vivo keratitis model as well as with corneal epithelial cells to advance the understanding about the system in the future before any preclinical study. Additionally, MH can be a simple option to combine with anti-fungal therapy in superficial fungal infections like skin and nails as well.
Acknowledgments
The authors sincerely thank Center for Nano Science and Engineering (CeNSE), Center for Biosystems Science and Engineering (BSSE), and Materials Engineering at Indian Institute of Science, Bangalore for providing various research facilities and specially thank Prof. Atul H. Chokshi for the Ambrell easy heat induction heater facility.
Disclosure statement
The authors report no conflict of interest.
Additional information
Funding
References
- Ansari Z, Miller D, Galor A. Current thoughts in fungal keratitis: diagnosis and treatment. Curr Fungal Infect Rep. 2013;7:209–218.
- Sridhar MS. Anatomy of cornea and ocular surface. Indian J Ophthalmol. 2018;66:190.
- Yarmolenko PS, Moon EJ, Landon C, et al. Thresholds for thermal damage to normal tissues: an update. Int J Hypertherm. 2011;27:320–343.
- Prajna VN, Prajna L, Muthiah S. Fungal keratitis: the Aravind experience. Indian J Ophthalmol. 2017;65:912.
- Qiu S, Zhao G-Q, Lin J, et al. Natamycin in the treatment of fungal keratitis: a systematic review and meta-analysis. Int J Ophthalmol. 2015;8:597.
- Gopinathan U, Sharma S, Garg P, et al. Review of epidemiological features, microbiological diagnosis and treatment outcome of microbial keratitis: experience of over a decade. Indian J Ophthalmol. 2009;57:273.
- Srinivasan M, Upadhyay MP, Priyadarsini B, et al. Corneal ulceration in south-east Asia III: prevention of fungal keratitis at the village level in south India using topical antibiotics. Br J Ophthalmol. 2006;90:1472–1475.
- Netea MG, Brown GD. Fungal infections: the next challenge. Curr Opin Microbiol. 2012;15:403–405.
- Fanning S, Mitchell AP. Fungal biofilms. PLOS Pathog. 2012;8:e1002585.
- Stone D, Tan JF. Fungal keratitis: update for 2014. Curr Ophthalmol Rep. 2014;2:129–136.
- Ti S-E, Scott JA, Janardhanan P, et al. Therapeutic keratoplasty for advanced suppurative keratitis. Am J Ophthalmol. 2007;143:755–762.
- Barbany M, Gris O, Güell JL. Therapeutic sectorial full-thickness sclero-keratoplasty for recurrent fungal keratitis. Arch Soc Esp Oftalmol. (English Edition). 2015;90:385–388.
- Sun RL, Jones DB, Wilhelmus KR. Clinical characteristics and outcome of Candida keratitis. Am J Ophthalmol. 2007;143:1043–1045.
- Sharma N, Chacko J, Velpandian T, et al. Comparative evaluation of topical versus intrastromal voriconazole as an adjunct to natamycin in recalcitrant fungal keratitis. Ophthalmology. 2013;120:677–681.
- Arboleda A, Miller D, Cabot F, et al. Assessment of rose bengal versus riboflavin photodynamic therapy for inhibition of fungal keratitis isolates. Am J Ophthalmol. 2014;158:64–70.
- Kaluarachchi WD, Cisneros BT, Corr SJ, et al. Aspergillus fumigatus hyphal damage caused by noninvasive radiofrequency field-induced hyperthermia. Antimicrob Agents Chemother. 2013;57:4444–4448.
- Li X, Zhang C, Hong Y, et al. Local hyperthermia treatment of extensive viral warts in Darier disease: a case report and literature review. Int J Hypertherm. 2012;28:451–455.
- Kolosnjaj-Tabi J, Wilhelm C. Magnetic nanoparticles in cancer therapy: how can thermal approaches help? Nanomedicine 2017;12:573–575.
- Singh D, McMillan JM, Kabanov AV, et al. Bench-to-bedside translation of magnetic nanoparticles. Nanomedicine. 2014;9:501–516.
- Johannsen M, Gneveckow U, Eckelt L, et al. Clinical hyperthermia of prostate cancer using magnetic nanoparticles: presentation of a new interstitial technique. Int J Hypertherm. 2005;21:637–647.
- Maier-Hauff K, Ulrich F, Nestler D, et al. Efficacy and safety of intratumoral thermotherapy using magnetic iron-oxide nanoparticles combined with external beam radiotherapy on patients with recurrent glioblastoma multiforme. J Neurooncol. 2011;103:317–324.
- Lübbe AS, Bergemann C, Riess H, et al. Clinical experiences with magnetic drug targeting: a phase I study with 4'-epidoxorubicin in 14 patients with advanced solid tumors . Cancer Res. 1996;56:4686–4693.
- Fantechi E, Innocenti C, Zanardelli M, et al. A smart platform for hyperthermia application in cancer treatment: cobalt-doped ferrite nanoparticles mineralized in human ferritin cages. ACS Nano. 2014;8(5):4705–4719.
- Pankhurst QA, Connolly J, Jones S, et al. Applications of magnetic nanoparticles in biomedicine. J Phys D 2003;36(13):R167.
- Kaur P, Aliru ML, Chadha AS, et al. Hyperthermia using nanoparticles – promises and pitfalls. Int J Hypertherm. 2016;32:76–88.
- Calderone RA, Fonzi W. Virulence factors of Candida albicans. Trends Microbiol. 2001;9(7):327–335.
- Tiwari P, Agarwal S, Srivastava S, et al. The combined effect of thermal and chemotherapy on HeLa cells using magnetically actuated smart textured fibrous system. J Biomed Mater Res B Appl Biomater. 2018;106:40–51.
- Wayne PA. Reference method for broth dilution antifungal susceptibility testing of yeasts, approved standard. CLSI document M27-A2 (2002).
- Pittenger MF, Mackay AM, Beck SC, et al. Multilineage potential of adult human mesenchymal stem cells. Science. 1999;284(5411):143–147.
- Yao L, Bai H. Mesenchymal stem cells and corneal reconstruction. Mol Vis. 2013;19:2237.
- Gu S, Xing C, Han J, et al. Differentiation of rabbit bone marrow mesenchymal stem cells into corneal epithelial cells in vivo and ex vivo. Mol Vis. 2009;15:99–107.
- Saraswathi P, Beuerman RW. Corneal biofilms: from planktonic to microcolony formation in an experimental keratitis infection with Pseudomonas aeruginosa. Ocular Surf. 2015;13:331–345.
- Trembly BS. Thermal techniques for reshaping the cornea. Matching the energy source to the clinical need: a critical review. Int Soc Optics Photonics. 2000;10297(04):51–79.
- Shaw EL, Gasset AR. Thermokeratoplasty (Tkp) temperature profile. Invest Ophthal Vis Sci. 1974;13:181–186.
- Kim Y-J, Ebara M, Aoyagi T. A smart hyperthermia nanofiber with switchable drug release for inducing cancer apoptosis. Adv Funct Mater. 2013;23(46):5753–5761.
- Hamilton‐Miller JMT. The effect of pH and of temperature on the stability and bioactivity of nystatin and amphotericin B. J Pharm Pharmacol. 1973;25:401–407.
- Liao RS, Rennie RP, Talbot J, et al. Assessment of the effect of amphotericin B on the vitality of Candida albicans. Antimicrob Agents Chemother. 1999;43(5):1034–1041.
- Brinkmann R, Radt B, Flamm C, et al. Influence of temperature and time on thermally induced forces in corneal collagen and the effect on laser thermokeratoplasty. J Cataract Refrac Surg. 2000;26:744–754.
- Johannsen M, Thiesen B, Wust P, et al. Magnetic nanoparticle hyperthermia for prostate cancer. Int J Hypertherm. 2010;26:790–795.
- Zhang H-G, Mehta K, Cohen P, et al. Hyperthermia on immune regulation: a temperature's story. Cancer Lett. 2008;271:191–204.
- Mencucci R, Ambrosini S, Ponchietti C, et al. Ultrasound thermal damage to rabbit corneas after simulated phacoemulsification. J Cataract Refrac Surg. 2005;31(11):2180–2186.