Abstract
Objective: Hot environments are associated with impaired glucose metabolism at rest in healthy humans. The purpose of this study was to explore the contribution of key glucoregulatory hormones and biomarkers to this altered glucose tolerance.
Methods: The effects of ambient temperature on glucose tolerance and its determinants were assessed with a 3-hr oral glucose tolerance test (OGTT) administered to 19 healthy young men and women at 22 °C and 31 °C.
Results: The glucose response amplitude was greater in warm environment (AUC 904 ± 151 vs. 721 ± 89 mmol/l·180 min at 31 °C and 22 °C, respectively, p < .001). There was no significant effect of environmental temperature on insulin, growth hormone or pancreatic polypeptide concentrations (all p > .17). The cortisol response to the glucose load was reduced 30, 60, 90 and 120 minutes postload at 31 °C compared with 22 °C (p = .001). The interleukin-6 concentration was also lower in the session at 31 °C (p = .043).
Conclusion: We conclude that the effects of environmental temperature on the glucoregulatory hormones and biomarkers reported in this study do not explain the exaggerated increase in blood glucose after a glucose load taken in a warm environmental temperature.
Precis statement: This work demonstrates in healthy men and women that the ingestion of glucose elicits an exaggerated increase in blood glucose when the environmental temperature is warm.
Introduction
Hot environments are associated in humans with substrate shift during exercise [Citation1,Citation2] and impaired glucose metabolism at rest [Citation3–8], at least in the short term. The prevalence of abnormal glucose tolerance and gestational diabetes mellitus is markedly increased in pregnant women in the warmest season of the year compared with the coldest [Citation9,Citation10]. It remains an open question as to whether this phenomenon is only apparent, which was suggested based on the putative impact of the redistribution of blood flow between cutaneous and visceral beds [Citation5].
Glucose homeostasis is preserved in various conditions that are likely to modify the rate of glucose entering the circulation or the rate of glucose removal, with examples being, respectively, large food intake and intense exercise. When glucose is ingested, glucoregulatory hormones are released by the pancreas (insulin, amylin) to avoid hyperglycemia [Citation11]. More specifically, insulin increases cellular permeability to glucose and activates muscle and hepatic glycogenogenesis.
Other hormones and cytokines share hyperglycemic effects among their main functions. For example, glucagon, epinephrine, cortisol, growth hormone (GH), glucagon-like peptide-1, glucose-dependent insulinotropic peptide, thyroxine, and interleukin-6 (IL-6) increase the glucose influx more or less directly from the following sources: intestinal absorption during the fed state, endogenous glucose production from glycogenolysis, and gluconeogenesis [Citation12]. GH and cortisol particularly stimulate these metabolic mechanisms at the hepatic level. They also facilitate lipid oxidation in cases of low carbohydrate content and share anabolic effects through the stimulation of transcription and translation of gene expression to convert amino acids to protein.
Glucose homeostasis is also preserved by factors able to mediate hepatic sensitivity to insulin. This is the case of pancreatic polypeptide (PP), which regulates hepatic insulin receptor gene expression and hepatocyte insulin receptor availability [Citation12].
The purpose of this study was to identify the effects of environmental heat on key glucoregulatory hormones and biomarkers in order to improve understanding of the heat-related altered glucose tolerance in healthy humans. The effects of ambient temperature on glucose tolerance and its determinants were thus assessed by a 3-h oral glucose tolerance test (OGTT) performed in two different environments. The main biological outcomes were glucose, hormones with expected major hypo- and hyperglycemic effects (i.e., insulin, cortisol, GH) in this context, PP as a reflection of the gastrointestinal contribution to glucose homeostasis, and IL-6 as a major marker of inflammatory processes involved in glucoregulation and insulin sensitivity.
Material and methods
Participants and ethics
The data were from active, healthy young participants: 7 women and 12 men from a student population. Thirteen men had completed both trials but the results of one had to be discarded because his metabolic profile was potentially abnormal based on the index calculated from the homeostasis model assessment of insulin resistance and using the Matsuda index [Citation13].
During the initial contact by interview, phone or e-mail, potential participants were screened with regard to the eligibility criteria to discard smokers, dieters, individuals with disease or low or high weight at birth, and those who had been living in the Caribbean area for less than 2 years. During the second screening, health status and history of living in the Caribbean were assessed by interview, and eating behavior was characterized using the Three Factor Eating Questionnaire designed to assess uncontrolled eating, cognitive restraint and emotional eating [Citation14]. Students with elevated scores would have been discarded because part of the study included appetite evaluation, but all scores remained within normal values. The eligible volunteers were then invited to participate in the study and read the information note. They all signed a written informed consent form before the first session. They were financially compensated for completing the study.
All procedures used in this study were in accordance with institutional guidelines and the Helsinki Declaration of 2013. The study procedures were approved by the Human Subject Review Committee (CPP13-018a/2013-A01037-38). The protocol (APPA) was registered in EudraCT (2013–003206-25) and ClinicalTrials (NCT02157233).
Experimental procedures and blood sampling
After the screening visit, the participants were studied on two separate occasions separated by 3–10 days during a standard OGTT under two ambient temperatures: control (22 °C) and warm (31 °C), presented in random order. Both sessions were performed after an overnight fast for 12 h prior to the OGTT, starting from 6:30 AM. Participants were wearing light clothing. They were instructed to avoid alcohol and heavy exercise on the eve of the sessions and to have sufficient carbohydrate intake the day before their first session and comparable food intake from one session to another.
An intravenous cannula was inserted into an antecubital vein within 10-20 min of arrival at the laboratory and kept in place until the end of the session. Immediately after the first blood sampling (T0), the participants drank a solution containing 75 g of glucose dissolved in 250 ml of water (Gluco75; Odil SAS, Dijon, France) within 5 min. Postload blood samples were drawn at 30, 60, 90, 120 and 180 min (T30, T60, T90, T120, T180). Blood samples were immediately stored in a refrigerator at 4 °C before processing for further analyses. The participants remained in a seated position throughout the entire OGTT and engaged in quiet activities such as reading and talking. They were allowed to drink water ad libitum all through the sessions.
After the 22 °C session, the participants’ height and weight were measured and used to calculate the body mass index. Body composition was measured using an Inbody S10 4-electrode body composition analyzer (Biospace, Seoul, South Korea) based on bioelectric impedance analysis.
Analytical procedures
Plasma glucose, insulin and cortisol were analyzed within 2 h on a Cobas 6000 automatic platform (Roche, Mannheim, Germany) (<c501> module for glucose and < e601> module for insulin and cortisol) after centrifugation. Other plasma samples were aliquoted after centrifugation at 1600 × g for 15 min at 4 °C and frozen (−80 °C) for further analyses. The total area under the curve (AUC) for glucose, insulin and cortisol was calculated according to the trapezoidal rule.
The Matsuda index was calculated from the fasting glucose and insulin and the glucose and insulin from each of the OGTT timepoints, according to the published formula (14). The index obtained from the control session (22 °C) was used to characterize the metabolic profile. Values equal to or lower than 2.5 characterize whole body insulin resistance, and the higher the value is, the better the insulin sensitivity is.
IL-6, PP and GH concentrations were measured from frozen aliquots using Enzyme Immunoassay kits according to the manufacturer's protocols (ThermoFisher Scientific, Waltham, USA).
Statistical analyses
Repeated measure analyses of variance with two group factors (environmental temperature with 2 levels, and time with 6 or 4 measurement times depending on the variables) were performed for the relevant variables. Data were tested for sphericity using Mauchly’s test and if the assumption of sphericity was violated, the Greenhouse–Geisser correction was undertaken to adjust the degrees of freedom. Post-hoc Tukey’s tests were performed in cases of significant interaction effect. As the Matsuda index was non-normally distributed, Wilcoxon’s rank-sum tests were used to assess the effect of environmental temperatures. The AUC were analyzed with paired t-tests.
All results were analyzed with the SPSS v(0).20 software package (SPSS Inc, Chicago, IL, USA). Data are presented as mean ± SD or median [lower − upper quartile] according to the distribution, except in figures where SEMs are used. Statistical significance was set at p < .05.
Results
presents the participants’ anthropometric and metabolic characteristics.
Table 1. Characteristics of the participants.
No main gender effect and no interaction effect involving gender was noted on the glucose and insulin responses to the OGTTs. Therefore, the men’s and women’s data were pooled.
Blood glucose concentration () was affected by the environmental temperature (p = .03 for the higher values in warm compared with control environment), as well as by time (p < .001), but the temperature × time interaction was not significant (p = .12). The significantly greater glucose response in warm environment was also evidenced by the glucose AUC (904 ± 151 vs. 721 ± 89 mmol/l·180 min at 31 °C and 22 °C, respectively, p < .001).
Figure 1. Glucose (mmol/l) (A) and insulin (pmol/l) (B) responses during the session at 22 °C ambient temperature (white triangles, dashed line), and during the session at 31 °C (black triangles, solid line). A. Time p < .001; temperature p = .028 (as indicated by *); temperature × time p = .12. (B) Time p < .0001; temperature p = .17; metabolic level × temperature p = .21.
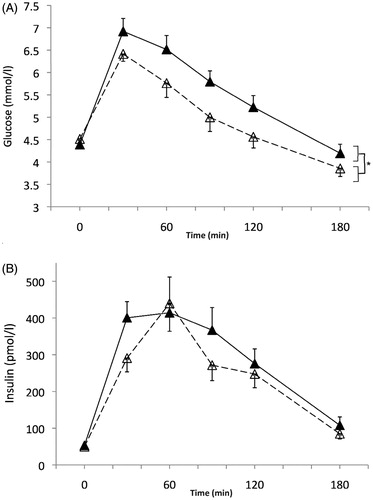
Blood insulin concentrations increased with time within the OGTT (p < .001), but the main effect of ambient temperature was not significant (p = .17), nor was the interaction between this environmental factor and time (p = .21). Insulin AUC did not vary significantly with environmental temperature (44.5 ± 21.6 vs. 51.9 ± 30.5 nmol/l at 22 °C and 31 °C, respectively, p = .17).
The Matsuda index characterizing insulin sensitivity calculated from glucose and insulin measured during the OGTT was not significantly different (p = .08) at 22 °C (6.7 [5.5–9.9]) and at 31 °C (6.5 [5.3–6.9]).
Cortisol concentrations () were lower when the OGTT was performed in the warm environmental temperature as compared with the control (main effect of environmental temperature: p = .001). There was also a main effect of time (p < .001) with values globally dropping from baseline. The cortisol response to the glucose load was also affected by the environmental temperature, with a significant temperature x time interaction (p = .007). The post-hoc analyses revealed that cortisol concentrations were not significantly different at T0 and T180 between the two conditions of ambient temperature (p = .12 and p = .97, respectively). However, differences between the warm and control conditions were evidenced at 30, 60, and 90 and 120 min after the glucose load (all p < .001). In the warm condition, each value was significantly lower than the one measured before it (T30 vs. T0, T60 vs. T30, etc.) up to 120 min. In the control condition, this was observed only from T60 (vs. T30) and up to 180 min. The significantly reduced cortisol response to the glucose load in warm environment was also evidenced by the cortisol AUC (46.4 ± 15.7 vs. 73.5 ± 19.8 μmol/l·180 min at 31 °C and 22 °C, respectively, p < .001).
Figure 2. Cortisol (nmol/l) (A) and GH (µg/l) (B) responses during the session at 22 °C ambient temperature (white triangles, dashed line), and during the session at 31 °C (black triangles, solid line). (A) Time p < .001; temperature p = .001; temperature × time p = .007. *Significant temperature effect at different sampling times, from the post-hoc tests. (B) Time p = .005; temperature p = .60; temperature × time p = .46.
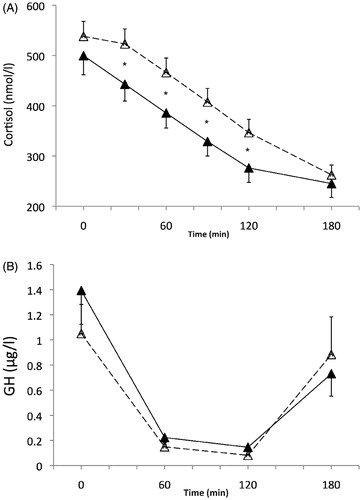
GH concentrations significantly decreased throughout time after the glucose load (main time effect, p = .005). This general decrease was independent of the ambient temperature (main effect of temperature, p = .60, time x temperature interaction effect, p = .46).
There was no significant variation in PP concentrations in response to the glucose load (main effect of time, p = .38), nor in relation to the ambient temperature (main effect: p = .53; interaction effect with time, p = .23, ).
Figure 3. Pancreatic peptide (µg/l) (A) and IL-6 (ng/l) (B) responses during the session at 22 °C ambient temperature (white triangles, dashed line), and during the session at 31 °C (black triangles, solid line). (A) Time p = .38; temperature p = .53; temperature × time p = .23. (B) Time p < .001; temperature p = .043 (as indicated by *); temperature × time p = .68.
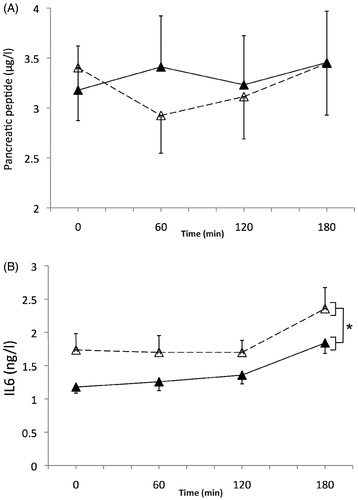
Environmental temperature had a significant main effect on IL-6 concentrations (p = .043), with an overall higher level in the control condition (22 °C). Time also had a significant effect (p < .001), but the temperature x time interaction was not significant (p = .68, ).
Discussion
We hypothesized that glucoregulatory and inflammatory biomarkers would display specific patterns in relation to warm environmental temperature, which might help explain the glucose metabolism impairment reported in this environment. We confirmed that a glucose load elicits an exaggerated increase in blood glucose in a warm environment. None of the biomarkers explored in this study varied significantly under the influence of ambient temperature in a way likely to explain the greater increase in blood glucose in a warm environment.
Our aim with this study was to elucidate the mechanisms likely to be involved in heat-related glucose metabolic impairment. The results from animal studies are of little help in understanding this phenomenon because glucose tolerance is paradoxically improved by heat treatment in animal models [Citation15–17]. It should nevertheless be kept in mind that the species used for these studies were furred and may have a specific cutaneous blood flow, an unusual ability to activate brown adipose tissue, and contributions from sudation and thermal polypnea in thermoregulation. Therefore, it is unlikely that observations in mice or rats exposed to heat, most often through warm baths, are relevant to building explanatory hypotheses about our current results in humans.
The potential causes for disrupted glucoregulation, whether in a short or longer term, depend on hyperglycemic and hypoglycemic hormones and the signaling pathways of their receptors. The prevention of hyperglycemia in humans is mainly achieved by the activation of insulin and, secondarily, the dissipation of glucose counterregulatory (glucose-raising) systems impacting the liver, kidney and skeletal muscle [Citation18]. We thus investigated the impact of environmental temperature on: (1) the activation of hormones with a hyperglycemic effect or the restricted lowering of their concentration in response to a glucose load that indicates the failed suppression of endogenous glucose production, and/or (2) the inhibition of hypoglycemic factors.
Regarding the hyperglycemic factors, which are expected to have a limited impact on glucoregulation after a glucose load, data from the four main hormones would be needed to have a complete picture. These hormones are glucagon and epinephrine, known for their rapid effects in hypoglycemia risk, and cortisol and GH, which have delayed actions. We focused on the last two because the mechanisms by which glucagon secretion is inhibited depend on insulin [Citation19], which suggests its secondary contribution, and because the range for adrenalin to drop is so small that the normal response to a glucose load was reported to be minor or no inhibition [Citation20].
An important metabolic function of cortisol in the fasting state and during exercise is the preservation of the glucose supply through the stimulation of gluconeogenesis [Citation21,Citation22] and, incidentally, hepatic and muscle glycogenolysis. Cortisol secretion has a marked circadian rhythm [Citation23], and the acrophase is observed within 1 h of awakening. This is followed by a gradual decrease over the course of the morning and the rest of the day. In our study, the ‘time effect’ reported for cortisol thus covered the effect of the glucose load and the concomitant effect of duration since awakening. It was characterized by an overall decrease, which is absolutely consistent with its physiological functions. The other statistical results (the main effect of environmental temperature and the interaction effect between time and environment) show that the decrease was more marked in the warmer condition, in addition to the overall lower values in warmth. In the literature, cortisol concentrations have usually been reported to be elevated during exercise in the heat [Citation24] and with passive heat exposure [Citation25], possibly related to an increase in core temperature (and not skin temperature) and/or an increase in physiological stress [Citation24]. The lower cortisol levels reported in this study are not surprising given that the participants were heat-acclimatized and the heat stress was modest. Our observations exclude the contribution of cortisol to the exaggerated glucose response in this condition. Indeed, because of its hyperglycemic effects, it should have displayed overall higher values or a smaller decrease in the warmer condition than in the control condition to be putatively involved in the glucose response.
The notion of a GH contribution can be discarded for similar reasons. Apart from its main anabolic function, GH acts as a stress hormone that stimulates the release of glucose into circulation. Our results agree with the more or less complete suppression of GH secretion and the clearance of circulating GH in response to a glucose load in normal participants described in an earlier study [Citation26]. The decrease in GH concentration was similar in the two environmental temperatures, instead of being canceled or weakened. Therefore, mechanisms directly related to GH can be eliminated as potential explanations, although with less confidence than for cortisol.
Other groups and ours have quite consistently reported an exaggerated increase in blood glucose after a meal or a glucose load taken in a warm environmental temperature [Citation3–8,Citation27] in the human model. The discrepancies in the literature are related to the concomitant insulin response, which is the strongest hypoglycemic factor. Different profiles have been reported, with some studies describing a heat-related increase in the postprandial (or post-glucose load) rise in insulin concentration [Citation3,Citation4,Citation7], and others describing no significant effect [Citation5] in relation to environmental temperature. This was also the case in our study. The reasons for these discrepancies are not clear, although they may theoretically be related to the glucose load or the participants’ heat acclimatization, physical fitness, age, initial glucose tolerance, etc. The fact is that the insulin response was never reduced. It can thus be concluded that the exaggerated blood glucose excursion was not consecutive to a limited response of the pancreatic beta cells. The insulin and glucose profiles were instead indicative of the failure of insulin, despite a normal release in the warm condition, to prevent hyperglycemia as efficiently as in the control environmental temperature.
Another factor that may help explain our results is the gastrointestinal contribution to glucose homeostasis. An increased absorption rate with a lower proportion of the glucose load retained by the splanchnic bed could lead to higher peak values of blood glucose. We assumed that the measurement of the pancreatic polypeptide hormone would affirm or reject this hypothesis. PP is known to play a role in the regulation of pancreatic endocrine secretions and other metabolic processes. For example, PP infusion reverses hepatic insulin resistance in patients who are PP-deficient due to pancreatic resection or chronic pancreatitis [Citation28]. It is expected to increase rapidly in healthy humans after food ingestion, particularly when the meal is rich in proteins, although its actions are quite complex since intravenous boluses and oral intake loads have opposite effects [Citation29]. Its interaction with glucose metabolism and insulin sensitivity, in association with its effects on gut function, suggest it may be a serious candidate. However, we were unable to identify the effects of the environmental temperature, the glucose load, or the interaction between them. Our understanding was thus not improved by the PP results in this study.
Interleukin-6 is a pro-inflammatory myokine that contributes to glucose homeostasis and insulin sensitivity. It is involved in the control of local and systemic acute inflammatory responses that are associated with impaired insulin sensitivity. Whether it promotes insulin resistance or is subsequent to it has been debated [Citation30]. The change in its concentration during the OGTT was expected and showed a rather late and moderate increase, similar to previous observations [Citation31]. It is interesting to note that the IL-6 levels, like the cortisol levels, were lower with warmer temperature. This profile converges with earlier studies that have shown that interleukins can activate the hypothalamic-pituitary-adrenal axis [Citation32]. Increased acute exposure to IL-6 was shown to increase glucose transport and utilization in intact human skeletal muscle [Citation12]. We thus suggest that the lower IL-6 concentration in warmer temperature might have contributed to lower cortisol, reduced glucose utilization by tissues, and limited glucose release from gluconeogenesis, consecutively to the accumulation of glucose in the post-glucose load phase.
One hypothesis for this observation involves brown adipose tissue, known to function in the dissipation of chemical energy in response to cold or excess feeding [Citation33]. An increased recruitment of brown adipose tissue is associated with improved regulation of glucose homeostasis and enhanced postprandial insulin sensitization and energy metabolism. This occurs in relation to skin temperature changes and does not require central temperature modification [Citation34]. In addition, based on rodent studies, brown adipose tissue-derived IL-6 was reported to be necessary to improve glucose homeostasis [Citation33]. Accordingly, with warmer environmental temperature, it is hypothesized that there is less recruitment of brown adipose tissues, accompanied by a lower release of IL-6. The consequence is less sensitivity to insulin and reduced regulation of glucose homeostasis, as observed in the current study. These findings support the emerging evidence on the role of ambient temperature-induced hormones.
Our results highlight the need for further investigation on this issue, which is important from theoretical and clinical points of view. In particular, a demonstration of the likelihood of reduced insulin sensitivity in the heat is needed and the alterations in the corresponding signaling pathways should be identified. The data from this study bring further evidence that standardization or correction of the temperature during glucose tolerance testing may be required. They also raise questions about a putative overdiagnosis of diabetes when the test is performed in a warm environmental temperature. Striking epidemiological studies have underlined the clinical reality of the feature apprehended in our study from an experimental physiological viewpoint. For example, doubled glucose intolerance rates on warmer days in a temperate zone have been reported in pregnant women [Citation9,Citation10]. More recently, the age-adjusted incidence of diabetes was shown to be related to outdoor temperature [Citation35]. This study thus supports the hypothesis of a real – and not only apparent − increase in glucose impairment risk with heat exposure.
In conclusion, we report an exaggerated increase in blood glucose after a glucose load taken in a warm environmental temperature in normal healthy men and women. Mechanisms in relation to cortisol and GH were discarded from those likely to be involved in this metabolic impairment. We suggest that further investigations focused on inflammatory mechanisms and their control of brown adipose tissue activity may well provide significant insights on these questions.
Acknowledgements
We warmly thank the participants, and the nurse and physician (Véronique Conord, Stéphane Henri) for their great involvement. The authors also thank Cathy Carmeni for her excellent revision and suggestions.
Disclosure statement
The authors report no conflict of interest.
Additional information
Funding
References
- Febbraio MA, Snow RJ, Hargreaves M, et al. Muscle metabolism during exercise and heat stress in trained men: effect of acclimation. J Appl Physiol. 1994;76:589–597.
- Jentjens R, Wagenmakers AJM, Jeukendrup AE. Heat stress increases muscle glycogen use but reduces the oxidation of ingested carbohydrates during exercise. J Appl Physiol. 2002;92:1562–1572.
- Akanji AO, Bruce M, Frayn K, et al. Oral glucose tolerance and ambient temperature in non-diabetic subjects. Diabetologia. 1987;30:431–433.
- Frayn KN, Whyte PL, Benson HA, et al. Changes in forearm blood flow at elevated ambient temperature and their role in the apparent impairment of glucose tolerance. Clin Sci. 1989;76:323–328.
- Moses RG, Patterson MJ, Regan JM, et al. A non-linear effect of ambient temperature on apparent glucose tolerance. Diabetes Res Clin Pract. 1997;36:35–40.
- Dumke CL, Slivka DR, Cuddy JS, et al. The effect of environmental temperature on glucose and insulin after an oral glucose tolerance test in healthy young men. Wilderness Environ Med. 2015;26:335–442.
- Faure C, Charlot K, Henri S, et al. Impaired glucose tolerance after brief heat exposure: a randomized crossover study in healthy young men. Clin Sci. 2016;130:1017–1025.
- Antoine-Jonville S, Faure C, Hue O, et al. Ambient temperature-related exaggerated post-prandial insulin response in a young athlete: a case report and implications for climate change. Asia Pac J Clin Nut. 2018;27:487–489.
- Schmidt MI, Matos MC, Branchtein L, et al. Variation in glucose tolerance with ambient temperature. Lancet. 1994;344:1054–1055.
- Moses RG, Wong VC, Lambert K, et al. Seasonal changes in the prevalence of gestational diabetes mellitus. Diabetes Care. 2016;39:1218–1221.
- Aronoff S, Berkowitz K, Shreiner B, et al. Glucose metabolism and regulation: beyond insulin and glucagon. Diabetes Spectrum. 2004;17:183–190.
- Glund S, Deshmukh A, Long YC, et al. Interleukin-6 directly increases glucose metabolism in resting human skeletal muscle. Diabetes. 2007;56:1630–1637.
- Matsuda M, DeFronzo RA. Insulin sensitivity indices obtained from oral glucose tolerance testing: comparison with the euglycemic insulin clamp. Diabetes Care. 1999;22:1462–1470.
- Stunkard AJ, Messick S. The three-factor eating questionnaire to measure dietary restraint, disinhibition and hunger. J Psychosom Res. 1985;29:71–83.
- Gupte AA, Bomhoff GL, Swerdlow RH, et al. Heat treatment improves glucose tolerance and prevents skeletal muscle insulin resistance in rats fed a high-fat diet. Diabetes. 2009;58:567–578.
- Gupte AA, Bomhoff GL, Touchberry CD, et al. Acute heat treatment improves insulin-stimulated glucose uptake in aged skeletal muscle. J Appl Physiol. 2011;110:451–457.
- Kokura S, Adachi S, Manabe E, et al. Whole body hyperthermia improves obesity-induced insulin resistance in diabetic mice. Int J Hyperthermia. 2007;23:259–265.
- Meyer C, Dostou JM, Welle SL, et al. Role of human liver, kidney, and skeletal muscle in postprandial glucose homeostasis. Am J Physiol Endocrinol Metab. 2002;282:E419–427.
- Kaneko K, Shirotani T, Araki E, et al. Insulin inhibits glucagon secretion by the activation of PI3-kinase in In-R1-G9 cells. Diabetes Res Clin Pract. 1999;44:83–92.
- Horton TJ, Hill JO. Prolonged fasting significantly changes nutrient oxidation and glucose tolerance after a normal mixed meal. J Appl Physiol. 2001;90:155–163.
- Oh KJ, Han HS, Kim MJ, et al. Transcriptional regulators of hepatic gluconeogenesis. Arch Pharm Res. 2013;36:189–200.
- Khani S, Tayek JA. Cortisol increases gluconeogenesis in humans: its role in the metabolic syndrome. Clin. Sci. 2001;101:739–747.
- Krieger DT, Allen W, Rizzo F, et al. Characterization of the normal temporal pattern of plasma corticosteroid levels. J Clin Endocrinol Metab. 1971;32:266–284.
- Bridge MW, Weller AS, Rayson M, et al. Ambient temperature and the pituitary hormone responses to exercise in humans. Exp Physiol. 2003;88:627–635.
- Moller N, Beckwith R, Butler PC, et al. Metabolic and hormonal responses to exogenous hyperthermia in man. Clin Endocrinol. 1989;30:651–660.
- Stewart PM, Smith S, Seth J, et al. Normal growth hormone response to the 75g oral glucose tolerance test measured by immunoradiometric assay. Ann Clin Biochem. 1989;26: 205–206.
- Akanji AO, Oputa RN. The effect of ambient temperature on glucose tolerance. Diabet Med. 1991;8:946–948.
- Brunicardi FC, Chaiken RL, Ryan AS, et al. Pancreatic polypeptide administration improves abnormal glucose metabolism in patients with chronic pancreatitis. J Clin Endocrinol Metab. 1996;81:3566–3572.
- Sive AA, Vinik AI, van Tonder SV. Pancreatic polypeptide (PP) responses to oral and intravenous glucose in man. Am J Gastroenterol. 1979;71:183–185.
- Pedersen BK, Febbraio MA. Interleukin-6 does/does not have a beneficial role in insulin sensitivity and glucose homeostasis. J Appl Physiol. 2007;102:814–816.
- Choi HJ, Jeon SY, Hong WK, et al. Effect of glucose ingestion in plasma markers of inflammation and oxidative stress: analysis of 16 plasma markers from oral glucose tolerance test samples of normal and diabetic patients. Diabetes Res Clin Pract. 2013;99:e27–e31.
- Mastorakos G, Chrousos GP, Weber JS. Recombinant interleukin-6 activates the hypothalamic-pituitary-adrenal axis in humans. J Clin Endocrinol Metab. 1993;77:1690–1694.
- Stanford KI, Middelbeek RJ, Townsend KL, et al. Brown adipose tissue regulates glucose homeostasis and insulin sensitivity. J Clin Invest. 2013;123:215–223.
- Boon MR, Bakker LEH, van der Linden RAD, et al. Supraclavicular skin temperature as a measure of 18F-FDG uptake by BAT in human subjects. PLoS One. 2014;9:e98822
- Blauw LL, Aziz NA, Tannemaat MR, et al. Diabetes incidence and glucose intolerance prevalence increase with higher outdoor temperature. BMJ Open Diab Res Care. 2017;5:e000317.