Abstract
Purpose
High-intensity focused ultrasound (HIFU) is a potential noninvasive thermal ablation method for the treatment of peripheral artery disease. Dual-mode ultrasound arrays (DMUA) offer the possibility of simultaneous imaging and treatment. In this study, safety and feasibility of femoral artery robot-assisted HIFU/DMUA therapy was assessed.
Methods
In 18 pigs (∼50kg), angiography and diagnostic ultrasound were used to visualize diameter and blood flow of the external femoral arteries (EFA). HIFU/DMUA-therapy was unilaterally applied to the EFA dorsal wall using a 3.5 MHz, 64-element transducer, closed-loop-control was used to automatically adjust energy delivery to control thermal lesion formation. A continuous lesion of at least 25 mm was created by delivering 6–8 HIFU shots per imaging plane perpendicular to the artery spaced 1 mm apart. Directly after HIFU/DMUA-therapy and after 0, 3 or 14 days follow up, diameter and blood flow were measured and the skin was macroscopically examined for thermal damage. The tissue was removed for histological analysis.
Results
No complications were observed. The most frequently observed treatment effect was formation of scar tissue, predominantly in the adventitia and the surrounding tissue. No damage to the endothelium or excessive damage of the surrounding tissue was observed. There was no significant decrease in the mean arterial diameter after HIFU/DMUA-therapy.
Conclusion
HIFU/DMUA therapy successfully targeted the vessel walls of healthy porcine arteries, without causing endothelial damage or other vascular complications. Therefore, this therapy can be safely applied to healthy arterial walls in animals. Future studies should focus on safety and dose-finding in atherosclerotic diseased arteries.
Introduction
Peripheral artery disease (PAD) by atherosclerosis in the lower extremities may lead to progressive limitation of blood flow to the muscles and soft tissue, resulting in intermittent claudication or chronic limb ischemia (CLI) [Citation1]. Current treatment of PAD may include minimally-invasive interventions, like percutaneous transluminal angioplasty (PTA) and/or stenting, or open interventions, like arterial endarterectomy or bypass surgery [Citation1–3]. All these procedures carry a risk for associated perioperative complications, while the 5-year patency is limited to moderate [Citation4,Citation5]. To improve treatment outcomes and lower complication and mortality rates for patients, new treatment strategies for targeting atherosclerotic plaques are required. Currently, noninvasive thermal therapy with high intensity focused ultrasound (HIFU) is being explored as a potential treatment modality for atherosclerotic plaques.
In atherosclerosis, in vitro studies suggest that macrophages and fat-laden foam cells contribute to the accumulation of oxidized cholesterol, oxysterol, and cholesterol crystallization in plaques, which is toxic for the endothelium and smooth muscle cells and associated with plaque instability and rupture [Citation6–10]. Thermal therapy of in-vitro macrophages significantly reduced cholesterol crystallization and enhanced macrophage death [Citation11]. Furthermore, in response to macrophage activity and the subsequent hypoxic environment, proliferation of the vasa vasorum in the adventitial and plaque layer will occur. These newly formed vessels are often leaky and extravasation of inflammatory cells and red blood cells will lead to aggravation of local inflammation, increased cholesterol deposition and eventually plaque destabilization [Citation12–16].
HIFU is a noninvasive technique which can create sub-millimeter thermal (coagulation) lesions [Citation17–19]. HIFU in combination with Dual Mode Ultrasound Arrays (DMUA) offers a combined technique for both ultrasound (US) image guidance and subsequent delivery of HIFU therapy [Citation20]. Focusing HIFU beams will result in a rapid rise of local tissue temperature, which will result in local tissue damage through coagulative necrosis. Using the HIFU/DMUA system, real-time closed-loop control is used to monitor the lesion formation [Citation21,Citation22].
HIFU/DMUA-treatment of the atherosclerotic plaque and adjacent vasa vasorum might interrupt the cycle of cholesterol accumulation, inflammation, cholesterol crystallization and plaque destabilization, by attenuating and destroying the intraplaque macrophages and foam cells [Citation11,Citation23,Citation24] and by occluding the leaky vasa vasorum [Citation13–16]. It is hypothesized that ablation of the plaque will lead to renewed efferocytosis and healing by fibrosis [Citation25], caused by an influx of phagocytes from surrounding tissue, after which the newly formed fibrotic tissue will retract over time, leading to plaque stabilization and shrinkage [Citation21]. HIFU/DMUA has been shown feasible in targeting atherosclerotic plaques by creating discrete thermal lesions within the plaque, without damaging the vascular endothelium [Citation26]. It is hypothesized that the endothelium is protected by the heat-sink effect of the blood flow.
The aim of this study was to determine the safety and feasibility of HIFU/DMUA therapy on arteries and surrounding tissues and structures, using the HIFU/DMUA in combination with robotic arm navigation in an animal model.
Methods
All experiments were performed with prior approval from the Animal Experimentation Committee of the University Medical Center Utrecht and were performed according to the Guide for the Use and Care of Laboratory animals [Citation27]. In 18 pigs (± 50 kg, Topigs Norsvin) HIFU/DMUA (HIFU SynthesizerTM, International Cardio Corporation, Edina, MN, USA) therapy was applied unilaterally to the dorsal wall of the external femoral artery (EFA), using a 3.5 MHz, 64-element DMUA transducer (Imasonic SAS, Voray sur L’ognon, France). Acute (n = 7), short-term follow-up period (FUP) of three days (n = 5), and 14 days (n = 6), effects were studied with respect to safety.
Experimental protocol
The non-acute animals received antibiotics (amoxicillin/clavulanic acid, 12.5 mg/kg) starting three days before the procedure. At the day of the procedure, the animals were sedated, intubated and anesthetized according to a standardized protocol [Citation28]. Arterial access was gained through the carotid artery, and a 6 F multipurpose catheter was inserted to allow contrast injection. Before the procedure started, ultrasound- (US) and contrast angiography imaging were performed according to standardized protocol, to visualize and perform measurements (see ‘Measurements’) on the femoral arteries and veins.
HIFU/DMUA procedure
A six degrees-of-freedom UR3 robot (Universal Robots, Odense, Denmark) was used to navigate the HIFU/DMUA transducer to the target area (). This robotic device can precisely translate, rotate and keep the transducer stable at one position with an accuracy of ∼1 mm. During the procedure, the operator used a custom software program to control the movements of the robot.
Figure 1. (A) Example of the setup as used during the DMUA/HIFU procedure with the HIFU probe attached to a 6-degrees of freedom robotic arm. (B) Schematic image indicating the optimal focus from 45–55 mm from the transducer (A and A + D) with a focus range of 10 mm (D). The water bolus is approximately 15 mm (B), which results in an optimal focus range at 30–40 mm from the skin (C and C + D).
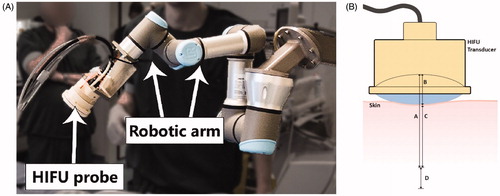
HIFU/DMUA therapy was applied with an initial intensity of approximately 6.25 kW/cm2, in situ, which was estimated based on array modeling and estimated tissue attenuation/absorption in the treatment region [Citation21]. At this intensity level, tissue boiling is expected to occur within 40–200 ms of the initiation of the HIFU shot [Citation29–31]. Tissue boiling results in significant increase in tissue echogenicity, as reported by numerous groups [Citation17,Citation32]. This is the basis for closed-loop control of HIFU-induced lesions described below.
The transducer was covered with a water bolus of 15 mm circulated with degassed water, to ensure US coupling between the transducer and the skin (). The optimal HIFU focus length is between 45–55 mm from the transducer apex, which practically results in a therapeutic range of 30–40 mm depth measured from the skin (). The HIFU/DMUA software allows for real-time target selection on the live ultrasound images and to deliver local HIFU therapy to the selected target using electronic beam steering as long as the target is located within the predefined therapeutic range.
Before the procedure started, the length of the target area was determined based on anatomical markers, such as vessel branches of the EFA and bone structure, but most importantly on vessel depth to ensure the dorsal vessel wall is within the optimal HIFU focus at 30–40 mm depth. The trajectory of the EFA within this range was selected as target area. The dorsal wall of the EFA was targeted to ensure targeting in the proximity of the vein, thereby theoretically increasing the risk on therapy induced vascular complications.
The HIFU/DMUA transducer was positioned perpendicular to the vessel, to obtain transversal imaging planes of the EFA (). Theoretically, a single lesion as created by the DMUA/HIFU system is a cigar shaped region of ∼1 mm2. Per transversal plane 6–8 HIFU/DMUA-shots, spaced 1 mm apart, were delivered to the dorsal side of the EFA. After delivery of these shots, the robot was used to move the probe 1 mm to target the next transversal plane, with the aim of creating a continuous lesion over the predetermined target trajectory. HIFU/DMUA therapy was performed in 25–35 (depending on the therapeutic range) transversal planes spaced 1 mm apart to create a continuous lesion of at least 25 mm. This resulted in at least 150 HIFU/DMUA-shots per procedure ().
Figure 2. (A) Schematic image of the EFA (red) and vein (blue) and the transversal imaging planes as imaged and treated during the procedure. 6–8 HIFU shots were delivered in between the artery and the vein (black asterisks) which form a continuous lesion (orange semicircle). The lower part of the scheme represents the simplified cutting process, differentiating between the target area and the sliced area. Area A contains the target area of 2.5–3.5 cm, while area B is outside the treatment range, which explains that we identified slides without histological changes. (B) (Diagnostic) ultrasound image of the transversal plane of the EFA (A) and femoral vein (V). The image view is the same as during the procedure while using the HIFU/DMUA imaging.
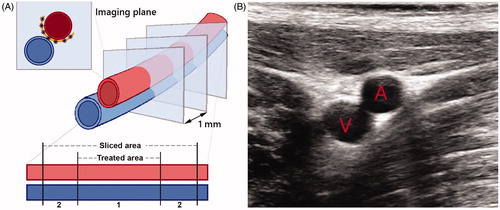
HIFU monitoring and feedback
The DMUA driver supports a specialized real-time imaging mode for monitoring HIFU, which is referred to as single transmit focus (STF) imaging [Citation29]. STF imaging is a high frame rate mode for real-time monitoring the various bioeffects such as tissue boiling. It is similar to the plane wave imaging used in conjunction with shear wave generation by focused ultrasound [Citation33]. In STF imaging mode, the same beamforming delays used for the therapy beam are used for the imaging beam with microsecond duration pulses suitable for imaging and at pressure levels consistent with diagnostic ultrasound.
STF imaging data are collected before, during and after HIFU shot delivery at 100 fps. For example, baseline data (with HIFU off) is collected for 1 s before turning HIFU on. While HIFU is turned on, STF transmissions are interleaved with HIFU bursts with strict timing constraints at a pulse-repetition frequency (PRF) of 100 Hz. The beamformed data is used to establish the baseline tissue echogenicity within a region of interest (ROI) around the target and at the bolus-skin interface. The baseline echogenicity estimate is obtained by computing the integrated back scatter (IBS) in 8 sub-blocks of the ROI, averaged for the pre-HIFU time interval (1 s typical). The system updates the IBS measurements following every HIFU burst at a PRF of 100 Hz. The updated IBS values are utilized as feedback for closed-loop control of HIFU application.
Closed-loop control of HIFU exposure
A closed-loop control (CLC) algorithm was designed to modulate the HIFU intensity for each burst based on echogenicity changes due to the application of HIFU on a burst-by-burst basis during the HIFU shot. The algorithm is described in detail by Aravalli et al. [Citation34]. Briefly, a HIFU shot is delivered over a user-specified exposure duration (e.g., 1 s) in bursts of 5 millisecond duration with a PRF of 100 Hz. The HIFU Synthesizer’s hardware/software architectures support simultaneous beamforming and signal processing of the echo data to provide real-time feedback on the state of the target tissue, within a certain therapeutic range, as well as the skin interface. A sudden increase in echogenicity at the skin interface causes immediate cessation of the HIFU application (subject to a user-selected threshold). An increase in target tissue echogenicity above a user-specified threshold causes the cessation of HIFU after a user-specified delay (e.g., 0 ms Standard CLC, or 300 ms Delayed CLC). The delayed CLC of HIFU exposure is accompanied by intensity modulation of the therapy beam down to a fraction of its initial intensity (e.g., 25%, user specified).
The closed-loop control protocol, target selection and time between shots and planes varied between the different subjects, according to three different protocols ().
Table 1. Characteristics of the difference protocols (1, 2 and 3) as used in this study.
In the acute experiments two worst-case scenarios were simulated on the contralateral EFA. First, delivery of numerous HIFU/DMUA-shots targeted at the same location, to simulate a situation in which the registration between targeted and untargeted tissue cannot be performed correctly. And second,by delivery of circumferential (around the EFA) HIFU/DMUA-shots for multiple transversal planes.
US and angiography imaging of the femoral arteries and veins were repeated directly after therapy and after the follow-up period of either 3 or 14 days for the non-acute animals. The skin was macroscopically examined for signs of thermal damage directly after therapy and after 3 or 14 days. The non-acute animals were monitored daily during their follow-up period for any signs of pain and discomfort.
After euthanization of the animals, the tissue from the inguinal region and the surrounding structures was removed bilaterally. To assure that all treated tissue was analyzed, the tissue was removed with wide margins (). The tissue was cut in 5–8 transversal segments of approximately 8–10 mm, and stained using tetrazolium-chloride (TTC), photographed and visually examined, subsequently the tissue was fixed in formaldehyde.
Measurements
Ultrasound
Diagnostic ultrasound was performed to visualize the EFA and vein before therapy, directly after therapy and after follow-up. The post-HIFU images were inspected for any signs of vascular complications, such as dissection, rupture or fistulas. Transversal and longitudinal images were captured over the whole trajectory of the EFA up to a depth of 50 mm from the skin. Longitudinal images were fused using Matlab (R2017a, The Mathworks Inc., Natick MA). The maximum diameter of the US recording was used for the segmentation of the ventral and dorsal wall, after which the mean vessel diameter over 30 mm of the target area was calculated (). Pre-HIFU, post-HIFU and after FUP mean vessel diameters were compared.
Figure 3. Example of diagnostic ultrasound image, as used for the calculation of the mean diameter of the target vessel. Two separate ultrasound images were fused (1 and 2), after which the diameter was determined (between the green and yellow lines).
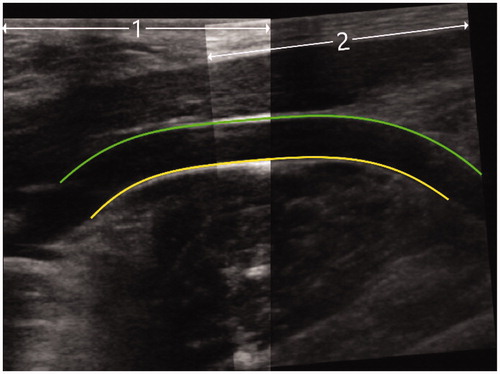
Independently, three observers (2 PhD candidates, 1 postdoc) visually examined the images for the occurrence of local stenosis and marked these with the following categories: no or minor stenosis (<30%), non-significant stenosis (30–50%) or significant stenosis (>50%).
Angiography
The 6 F multipurpose catheter was advanced from the carotid artery into the external iliac artery. Both the left and right EFA were visualized before therapy, directly after therapy and after follow up. Anterior-posterior contrast angiography was performed according to standardized protocol, by placing the catheter at the same position at all time points and by injecting 10 ml of contrast. The angiographic images were visually checked for any flow disturbances or other abnormalities, comparing pre- and post-treatment and FUP images.
Histology
According to standardized protocol, one side of each of the fixed segments was equalized, after which one histological slide per segment was cut. This results in a transversal slide containing the artery and vein and their surrounding tissue of the treated area. These slides were stained with H&E, Masson’s Trichrome, Elastic van Gieson and an ERG (endothelial marker) immunostain. All slides were examined by an experienced pathologist. The slides were examined for any damage to the arterial and venous vessel walls, the interstitial tissue and the surrounding muscle tissue, such as structural damage, inflammatory responses or development of scar tissue.
Statistical analysis
A three-way mixed ANOVA was performed to examine the effects of the different HIFU/DMUA-protocols, FUP time and side (treated, or untreated) on the change in mean arterial diameter (mm) measured with diagnostic US [Citation35]. Outliers were assessed by plotting and inspecting boxplots, normality was tested with Shapiro–Wilk’s test and homogeneity of variances was assessed with Levene’s test. Statistical significance was accepted at p < .05. The two-way interactions and main effects of HIFU/DMUA-protocols, FUP time or side on change in mean arterial diameter were analyzed separately as well. If significant two-way interactions or main effects were present, pairwise comparisons were performed and reported with 95% confidence intervals and Bonferroni-adjusted p values.
Results
An overview of the animal characteristics (protocol and follow-up time) is shown in . During all experiments the robotic guidance offered a feasible method to place and replace the HIFU/DMUA probe toward the target area. All experiments were completed without vascular complications, during or directly after delivery of HIFU/DMUA therapy. None of the animals showed any signs of discomfort or pain related to therapy, during the FUP of either 3 or 14 days. All animals were able to stand and walk within one day after the procedure.
Table 2. Mean diameters in mm of the EFA pretreatment (pre), post-treatment (post) and after the follow-up period (after FUP), per control-/treatment side per animal, categorized per protocol and follow-up period in days.
In one acute animal, the intentional excessive delivery of >100 HIFU/DMUA shots in one location led to signs of overtreatment at the skin surface. The tissue in the pre-focal area showed signs of tissue boiling forming a shape corresponding to the shape of the HIFU/DMUA beam.
Ultrasound analysis
The US-images of 14 out of 18 animals were analyzed. In the other 4 cases analysis of the images could not be performed, due to measurement difficulties caused by repositioning of the animals after HIFU-therapy or after follow up. Consequently, the resulting post-HIFU images could not be matched with the pre-HIFU images, leading to an unreliable comparison.
The mean US diameters data contained no outliers, data were normally distributed (p ≥ .05) and there was homogeneity of variances (p = .135). The three-way interacting effects between HIFU/DMUA-protocols, follow-up time and side on change in mean arterial diameter were not statistically significant, F(2,6)=0.270, p = .772, partial η2=0.083 (). None of the two-way interactions were statistically significant (p > .744). There was a non-significant trend toward a decrease in mean diameter directly after therapy (mean decrease in diameter of −13%), which normalized after a FUP of either 3 or 14 days (mean decrease in diameter of −6%). Post-HIFU images showed no signs of vascular complications.
Figure 4. Three-way interacting effects between HIFU/DMUA-protocols, follow-up time and side on change in mean arterial diameter (in mm) of the treated EFA.
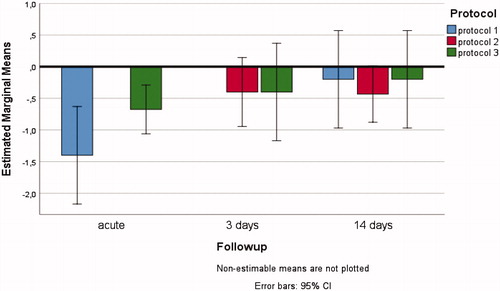
A total of 74 US images of 14 animals (pre HIFU, directly after HIFU therapy and after the FUP period, both the treated and untreated side) were visually scored by three blinded observers for potential stenosis severity. None of the images showed a functional obstruction of more than 50% decrease in diameter. Six images from 6 animals were scored with >30% decrease in diameter, directly after HIFU therapy ().
Table 3. Characteristics of the ultrasound images that were scored as mild stenosis (30–50%) directly post HIFU therapy.
Angiography
Directly after therapy, visual examination of the angiography images showed decreased flow on both the treated side as well as the contralateral side. In accordance with the US analysis, mild local stenoses were observed directly after therapy at the expected treated area in 6 animals (), which resolved after FUP. Total occlusion did not occur in any of the cases.
Histology
Acute histology of the treated arteries in animals without follow up revealed no or only very limited alterations of the tissue. No significant damage to the endothelium was observed, for either the standard protocols as well as the ‘worst-case’ scenarios.
For the animals with a follow up period, not all slices showed histological effects, as one would expect considering the cutting process. However, for all FUP animals at least one slide per animal showed histological changes. In the cases where the effect of the treatment was histologically visible, different stages of tissue damage and scar tissue formation were observed after 3 and after 14 days FUP. After 3 days FUP, fibrinoid changes with presence of macrophages were observed in the soft tissue between the femoral artery and vein and the adventitial layer of the femoral artery (). In addition, focal necrosis of the adjacent striated muscle tissue with inflammatory reaction was observed. In one artery, pyknosis of some smooth muscle cells in the outer layer of the media was observed. After 14 days FUP, discrete scar tissue lesions with abundant fibroblasts were observed in the soft tissue around the artery, the adventitial layer and in a few cases the outer part of the media. In some animals, small scars were observed in the adjacent striated muscle tissue (). In one animal there was limited histological damage to a nerve next to the artery with giant cell reaction.
Figure 5. Histology after 3 days FUP. At the upper left panel an overview of the EFA, vein and part of the surrounding connective tissue and muscle is shown (H&E). (A) Higher magnification of the intimal layer of the EFA that was completely intact with presence of endothelial cells (A1. H&E and A2. ERG immunostain) (B) Higher magnification of the medial and adventitial layer of the EFA and the connective tissue in between the artery and vein that revealed fibrinoid, reactive, changes with activated fibroblasts and macrophages (B1. H&E and B2. Elastic van Gieson stain). (C) Higher magnification of the striated muscle with an inflammatory infiltrate with macrophages around some necrotic striated muscle cells (C1. H&E and C2. Elastic van Gieson stain).
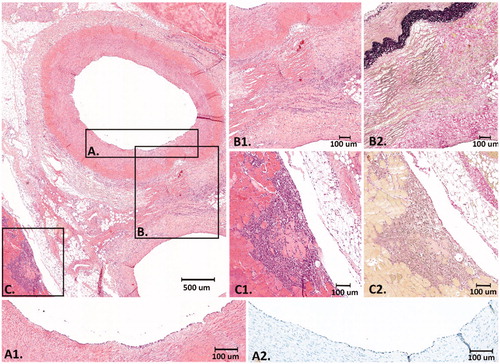
Figure 6. Histology after 14 days FUP. At the left panel an overview of the EFA, vein and the surrounding connective tissue and striated muscle is shown. The connective tissue between the artery and the vein is (partly) replaced by scar tissue with activated fibroblasts and collagen deposition. (A) Higher magnification of the intimal layer of the EFA with presence of endothelial cells and the medial layer that was largely unaffected (A1. H&E and A2: ERG immunostain). (B) The scar tissue extends into the surrounding striated muscle tissue with macrophages and remnant atrophic muscle cells (B1: H&E, B2: Elastic van Gieson stain and B3: Masson’s Trichrome stain).
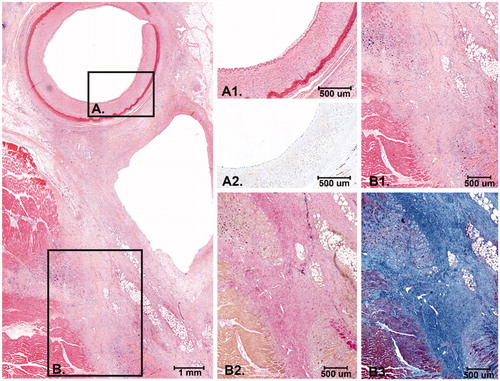
Discussion
In the present study the safety of HIFU/DMUA therapy on the EFA and the surrounding tissues and structures was investigated. HIFU/DMUA therapy was delivered to the dorsal vessel wall of the EFA in 18 pigs for a trajectory of 25–35mm, to examine the acute-, short-term- and long-term effects. The robotic guidance enabled precise positioning of the HIFU/DMUA probe throughout the procedures. All experiments were completed without any vascular complications. Histological analysis demonstrated that after the short-term FUP of 3 days the inflammatory response, as a result of the thermal HIFU/DMUA therapy, was at its peak. After the long-term FUP of 14 days the inflammatory activity decreased, and the scar tissue maturated ().
In one case, after a worst-case protocol, severe damage to the skin and the pre-focal tissue damage was observed directly after treatment. The intention was to simulate a worst-case scenario by targeting >100 HIFU/DMUA-shots at the same location, not within the optimal HIFU/DMUA-range and without correct closed loop control. Even in this scenario, no damage to the endothelium, arteriovenous fistula, rupture, dissection or total occlusion was observed, indicating that even for this worst-case scenario no severe damage to the artery and vein could be induced. These findings support the hypothesis that the heat-sink effect of the flowing blood protects the endothelium and vessel walls of both the artery and vein from severe thermal damage, as observed in previous research [Citation36].
Diameter EFA
Decrease in vessel diameter is an important safety parameter for the treatment of PAD patients, since the patency of the affected arteries is already decreased. The vessel diameter before and after HIFU therapy were compared, to study whether there is a significant (long term) decrease in diameter.
As shown in , changes in the mean arterial diameter were not significantly different between pigs with different HIFU/DMUA-protocols and FUP’s, nor were changes in diameter different between treated and untreated arteries per pig. However, a trend toward nonpermanent reduction of mean arterial diameter directly post treatment was observed in both untreated and treated femoral arteries. In addition, the angiography images showed a decreased flow directly after therapy in both the treated and untreated side. An explanation might be the influence of the general anesthesia and the duration of the procedure of up to six hours. Both the heart rate and the blood pressure decreased during this period. The effect normalized over time and was therefore most likely caused by temporary vasoconstriction and/or spasm in reaction to the HIFU/DMUA-therapy and/or general anesthesia.
In six cases an insignificant (mild) stenosis was scored in the US images. In three out of these six cases, the worst-case scenario protocol with excessive (circumferential) targeting was used, which does not represent realistic clinical conditions. Moreover, even after this worst-case protocol, no significant stenosis (>50%) was induced. In two out of these six cases, local stenosis was observed after a normal protocol was used. However, these stenoses resolved after FUP of 14 days, indicating that local stenosis is a temporary effect most likely caused by vasoconstriction and spasm as mentioned above.
Although the vasoconstriction and spasms never resulted in an (acute) occlusion or stenosis severity of >50% in this study, it is not known what the effect of vasoconstriction and spasms would be in patients with a near-occlusive stenosis. If this were to happen during the procedure, the advantage of the HIFU/DMUA technique is that the anatomical effects of the delivered therapy can be monitored simultaneously with the delivery of the therapy. Therefore, it is possible to pause the delivery of HIFU/DMUA until the spasm resolves.
Histology
On the acute histology no sign of macroscopic nor microscopic scar formation was seen, as would be expected due to tissue fixation. Moreover, no severe mechanical damage to the vascular structures and surrounding tissue was found, indicating acute safety. Histological analysis of the follow up slides that did show histological changes, demonstrated that HIFU therapy did not damage the endothelium. No severe structural damage of the arterial and venous vessel walls, such as ruptures or dissections were seen. After either 3 or 14 days FUP the process of an inflammatory response around the EFA could be observed, revealing the effect of thermal therapy after HIFU therapy. After 14 days FUP, discrete HIFU lesions were observed in the media and adventitia of the EFA. In part of the slides fibrotic lesions were found in the adjacent muscles, however these regions are small and therefore likely not of clinical relevance.
Clinical implications
The results of this study imply that HIFU/DMUA-therapy can be safely used to target the EFA without inducing endothelial damage or other vascular complications in an animal model. In a part of the treated animals, histology showed mild thermal effects around the EFA and discrete HIFU lesions in the medial and adventitial wall-layers of the EFA. In diseased arteries, these therapeutic effects might disrupt plaque growth and decrease plaque size by occluding the small, leaky vasa vasorum and by attenuating and destroying the intraplaque macrophages and foam cells, followed by efferocytosis and healing by fibrosis. However, the potential therapeutic effects of HIFU-treatment in PAD-patients remain unknown; therefore, additional human trials on safety, dose-finding and effectiveness are required.
If proven safe and effective, HIFU/DMUA therapy could replace the current, invasive, treatment strategies. HIFU/DMUA therapy is noninvasive and does not require general anesthesia. Therefore, this therapy might reduce the risk of complications and the need for hospitalization.
Limitations
Part of the histological slides of animals with a follow up period of 3 and 14 days did not demonstrate any changes, presumably caused by the difficult cutting process of the treated areas, due to high mobility of the surrounding muscles and the presence of a large amount of fatty tissue around the arteries. The sliced area was larger as compared to the treated area, increasing the probability that the treated area was analyzed, but also resulting in histological slices of the non-treated area (). During histological preparation, it was not possible to delineate between treated and untreated tissue. It is therefore possible that per animal, some of the treated areas were only partially histologically analyzed.
The present study was performed in healthy, young pigs with no history or symptoms of atherosclerotic plaques and therefore their vessels were not diseased. The present study suggests that the endothelium of the artery is not damaged after HIFU/DMUA therapy. Another study on stable atherosclerotic plaques in a porcine model found that the endothelium overlaying the plaques remained intact after HIFU/DMUA-therapy [Citation36]. However, it is unclear whether HIFU treatment can safely be applied to unstable or calcified plaques. Therefore, future research should also focus on safety of HIFU treatment in unstable and calcified plaques.
Conclusion
In this study it was demonstrated that it is safe to target a long trajectory of the arterial wall without inducing acute or long-term vascular complications in pigs. Future studies should focus on dose finding for optimal targeting and should demonstrate the feasibility and safety of targeting human atherosclerotic plaques using HIFU/DMUA therapy.
Acknowledgements
We would like to thank Marlijn Jansen, Joyce Visser, Martijn van Nieuwburg and Evelyn Velema for their work during the animal experiments.
Disclosure statement
Dr. Ebbini reports grants, personal fees and non-financial support from International Cardio Corporation, during the conduct of the study; grants and personal fees from International Cardio Corporation, grants and personal fees from National Institutes of Health, outside the submitted work; In addition, Dr. Ebbini has a patent Dual mode ultrasound transducer (DMUT) system and method for controlling delivery of ultrasound therapy with royalties paid to International Cardio Corporation, a patent Vascular characterization using ultrasound imaging with royalties paid to International Cardio Corporation, and a patent ULTRASOUND IMAGE FORMATION AND/OR RECONSTRUCTION USING MULTIPLE FREQUENCY WAVEFORMS with royalties paid to International Cardio Corporation.; Dr. Doevendans reports grants from International Cardio Corporation, during the conduct of this study and outside the submitted work; Dr. van Es has a patent NL2016800B1 issued, and a patent US20190125289A1 pending. Other authors have nothing to disclose.
Additional information
Funding
References
- Vahl AC, Reekers JA. The guideline ‘Diagnosis and treatment of peripheral artery disease of the lower extremities’ of The Netherlands Surgical Society. Ned Tijdschr Geneeskd. 2005;149(30):1670–1674.
- Hankey GJ, Norman PE, Eikelboom JW. Medical treatment of peripheral arterial disease. J Am Med Assoc. 2006;295(5):547–553.
- Lane R, Ellis B, Watson L, et al. Exercise for intermittent claudication. Cochrane Database Syst Rev. 2014;7(7):CD000990.
- Gallagher KA, Meltzer AJ, Ravin RA, et al. Gender differences in outcomes of endovascular treatment of infrainguinal peripheral artery disease. Vasc Endovascular Surg. 2011;45(8):703–711.
- Acin F, de Haro J, Bleda S. Primary nitinol stenting in femoropopliteal occlusive disease: a meta-analysis of randomized controlled trials. J. Endovasc. Ther. 2012;19(5):585–595.5.
- Brown AJ, Jessup W. Oxysterols and atherosclerosis. Atherosclerosis. 1999;142(1):1–28.
- Brown AJ, Leong SL., Dean RT, et al. W. 7-Hydroperoxycholesterol and its products in oxidized low density lipoprotein and human atherosclerotic plaque. J. Lipid Res. 1997;38(9):1730–1745.
- Lyons MA, Brown AJ. 7-Ketocholesterol. Int J Biochem Cell Biol. 1999; 31(3-4):369–375.
- Harada-Shiba M, Kinoshita M, Kamido H, et al. Oxidized low density lipoprotein induces apoptosis in cultured human umbilical vein endothelial cells by common and unique mechanisms. J. Biol. Chem. 1998;273(16):9681–9687.
- Nishio E, Watanabe Y. Oxysterols induced apoptosis in cultured smooth muscle cells through CPP32 protease activation and bcl-2 protein downregulation. Biochem. Biophys. Res. Commun. 1996;226(3):928–934.
- Geng Y-J, Phillips JE, Mason RP, et al. Cholesterol crystallization and macrophage apoptosis: implication for atherosclerotic plaque instability and rupture. Biochem Pharmacol. 2003;66(8):1485–1492.
- Moore KJ, Tabas I. Macrophages in the pathogenesis of atherosclerosis. Cell. 2011;145(3):341–355.
- Sluimer JC, Daemen MJ. Novel concepts in atherogenesis: angiogenesis and hypoxia in atherosclerosis. J Pathol. 2009;218(1):7–29.
- Sluimer JC, Gasc JM, van Wanroij JL, et al. Hypoxia, hypoxia-inducible transcription factor, and macrophages in human atherosclerotic plaques are correlated with intraplaque angiogenesis. J Am Coll Cardiol. 2008;51(13):1258–1265.
- Kolodgie FD, Gold HK, Burke AP, et al. Intraplaque hemorrhage and progression of coronary atheroma. N Engl J Med. 2003;349(24):2316–2325.
- Moreno PR, Purushothaman K-R, Sirol M, et al. Neovascularization in human atherosclerosis. Circulation. 2006;113(18):2245–2252.
- Ebbini ES, Ter Haar G. Ultrasound-guided therapeutic focused ultrasound: current status and future directions. Int J Hyperthermia. 2015;31(2):77–89.
- ter Haar G. HIFU tissue ablation: concept and devices. Adv Exp Med Biol. 2016;880:3–20.
- Liu D, Casper A, Haritonova A, et al. Adaptive lesion formation using dual mode ultrasound array system. AIP Conf. Proc. 2017;1821:1–6.
- Ebbini ES, Yao H, Shrestha A. Dual-mode ultra sound phased arrays for image-guided surgery. Ultrason Imaging. 2006;28(2):65–82.
- Almekkaway MK, Shehata IA, Ebbini ES. Anatomical-based model for simulation of HIFU-induced lesions in atherosclerotic plaques. Int J Hyperthermia. 2015;31(4):433–442.
- Casper A, Liu D, Ebbini ES. Realtime control of multiple-focus phased array heating patterns based on noninvasive ultrasound thermography. IEEE Trans Biomed Eng. 2012;59(1):95–105.
- Lepock JR. Cellular effects of hyperthermia: relevance to the minimum dose for thermal damage. Int J Hyperthermia. 2003;19(3):252–266.
- Roti JL. Cellular responses to hyperthermia (40-46 °C): cell killing and molecular events. Int J Hyperth. 2008;24(1):3–15.
- Kojima Y, Weissman IL, Leeper NJ. The role of efferocytosis in atherosclerosis. Circulation. 2017;135(5):476–489.
- Ballard JR, Casper AJ, Liu D, et al. Dual-mode ultrasound arrays for image-guided targeting of atheromatous plaques. AIP Conf Proc 2012;1503:124–128.
- National Research Council (US) Committee. Guide for the care and use of laboratory animals. Washington (DC): The National Academies Press; 2011.
- Jansen Of Lorkeers SJ, Gho JMIH, Koudstaal S, et al. Xenotransplantation of human cardiomyocyte progenitor cells does not improve cardiac function in a porcine model of chronic ischemic heart failure. Results from a randomized, blinded, placebo controlled trial. PLoS One. 2015;10(12):e0143953.
- Casper AJ, Liu D, Ballard JR, et al. Real-time implementation of a dual-mode ultrasound array system: in vivo results. IEEE Trans Biomed Eng. 2013;60(10):2751–2759.
- Khokhlova VA, Bailey MR, Reed JA, et al. Effects of nonlinear propagation, cavitation, and boiling in lesion formation by high intensity focused ultrasound in a gel phantom. J Acoust Soc Am. 2006;119(3):1834–1848.
- McLaughlan J, Rivens I, Leighton T, et al. A study of bubble activity generated in ex vivo tissue by high intensity focused ultrasound. Ultrasound Med Biol. 2010;36(8):1327–1344.
- Vaezy S, Shi X, Martin RW, et al. Real-time visualization of high-intensity focused ultrasound treatment using ultrasound imaging. Ultrasound Med Biol. 2001;27(1):33–42.
- Bercoff J, Tanter M, Fink M. Supersonic shear imaging: a new technique for soft tissue elasticity mapping. IEEE Trans Ultrason Ferroelectr Freq Control. 2004;51(4):396–409.
- Aravalli RN, Helden D, Van, Liu D, et al. Precision targeted ablation of fine neurovascular structures in vivo using dual-mode. Ultrasound Arrays Sci Rep. 2020;10(1)9249. DOI: 10.1038/s41598-020-66209-0
- Laerd Statistics Premium. Three-way mixed ANOVA. 2013; [cited 2018 Oct 22]. Available from: https://statistics.laerd.com/.
- Shehata IA, Ballard JR, Casper AJ, et al. Feasibility of targeting atherosclerotic plaques by high-intensity-focused ultrasound: an in vivo study. J Vasc Interv Radiol. 2013;24(12):1880–1887.e2.