Abstract
Purpose
When doxorubicin (DOX) is administered via lyso-thermosensitive liposomes (LTLD), mild hyperthermia enhances localized delivery to heated vs. unheated tumors. The optimal LTLD dose and the impact of different doses on systemic drug distribution are unknown.
Materials and methods: In this study, we evaluated local and systemic DOX delivery with three LTLD doses (0.1, 0.5, and 2.5 mg/kg) in a Vx2 rabbit tumor model. Temporally and spatially accurate controlled hyperthermia was achieved using a clinical MR-HIFU system for the intended heating duration (40 min).
Results: DOX concentration in tissues delivered from LTLD combined with MR-HIFU mild hyperthermia are dose-dependent, including heated/unheated tumor, heart, and other healthy organs. Higher DOX accumulation and tumor-to-heart drug concentration ratio, defined as the ratio of DOX delivered into the tumor vs the heart, were observed in heated tumors compared to unheated tumors in all three tested doses. The DOX uptake efficiency for each mg/kg of LTLD injected IV of heated tumor was significantly higher than that of unheated tumor and heart within the tested dose range (0.1-2.5 mg/kg). The DOX uptake for the heart linearly scaled up as a function of dose while that for the heated tumor showed some evidence of saturation at the high dose of 2.5 mg/kg.
Conclusions: These results provide guidance on clinical protocol design of hyperthermia-triggered drug delivery.
Introduction
Image-guided targeted drug delivery using the combination of mild hyperthermia and thermally-sensitive nanoparticles applies localized heating within a target volume of tissue to trigger release of drugs from circulating nanoparticles [Citation1–11]. Effective targeted drug delivery requires two components: 1) a nanoparticle capable of stable blood circulation and rapid release of its encapsulated contents when exposed to heat, and 2) a method of heating capable of achieving precise and stable spatiotemporal temperature elevations above the threshold for drug release, but below the threshold for tissue damage.
MR-guided high-intensity focused ultrasound (MR-HIFU) has many characteristics that make it suitable for generating mild-hyperthermia. Ultrasound energy can be focused to a millimeter-sized tissue volume deep in the body and can be electronically scanned to cover arbitrary 3D volumes. To control the delivery of focused ultrasound energy such that tissue is heated and maintained within the target temperature range (typically 41–45 °C) for tens of minutes, some form of temperature measurement is required. MR thermometry using the proton resonance frequency (PRF)-shift method offers a noninvasive method for monitoring temperature within the body with a precision of 1 °C or better [Citation12]. Although it has limitations in moving organs (e.g. liver) [Citation13,Citation14], PRF-based MR thermometry performs well in stationary tissues. When MR thermometry is combined with HIFU, a powerful closed loop form of image-guided thermal therapy can be realized, which has been applied to ablative treatments in the uterus, breast, prostate, and brain [Citation15–18], and is being developed for mild hyperthermia [Citation19].
One of the most mature and studied classes of nanoparticles responsive to heat are lyso-thermosensitive liposomes containing doxorubicin (LTLD), formulated for prolonged plasma half-life and rapid heat-triggered doxorubicin release [Citation9,Citation20–23]. Needham et al. reported that 50% of DOX can be released within a timescale of seconds, which allows high concentrations of DOX to be released intravascularly, quickly leading to extravasation into tumors through diffusion of the drug [Citation20]. In humans, LTLD extends the plasma half-life of doxorubicin to about 1 h, from only a few minutes for free doxorubicin [Citation24]. This circulation time allows continued release inside the tumor during prolonged heating and results in a high drug concentration gradient to facilitate the drug accumulation in tumor cells. Animal studies have shown that mild hyperthermia-triggered release of DOX encapsulated in temperature sensitive liposomes (LTLDs) can greatly enhance the average intratumoral concentration and ultimate therapeutic efficacy [Citation11,Citation25–28] compared to LTLD alone at the same dose per weight. This is consistent with the fact that thermosensitive liposomes are designed to be used in combination with hyperthermia, and therefore release very little drug at normal temperatures. The recent TARDOX clinical trial demonstrated the feasibility and safety of combining HIFU (without MR thermometry) with LTLD in patients with chemo-refractory liver tumors. After receiving HIFU exposures, an average 3.4-fold enhancement in intratumoral doxorubicin delivery was achieved compared to prior to HIFU exposures [Citation9,Citation29]. Other clinical studies combining LTLD with radiofrequency ablation of liver cancer [Citation22] or microwave hyperthermia of recurrent breast cancer on the chest wall [Citation30] have demonstrated promising rates of local control when adequate heating is delivered.
One of the primary concerns associated with DOX is its dose-dependent toxicity to multiple organ systems including cardiotoxicity, mucositis and myelosuppression. Most notably, patients treated with DOX are at risk of life-threatening cardiomyopathy and congestive heart failure, and this risk increases with the total lifetime exposure to doxorubicin [Citation31–33]. Because of this cardiotoxicity, the lifetime dose of DOX for a patient is limited. Preclinical studies indicate that the tissue distribution of DOX including heart and muscle/tumor is dependent on the injected dose [Citation34–36]. In rats, increasing DOX dose from 1 mg/kg to 4 mg/kg caused DOX deposition in the heart to increase from 4.3 to 30 µg/g at 1 h after the drug administration, resulting in a high risk of heart failure [Citation35].
Liposomal doxorubicin (i.e. Doxil®) has been demonstrated to decrease the risk of cardiotoxicity by preferentially distributing to tumors with increased vascular permeability rather than other organs including the heart with intact vasculature [Citation31]. Its effectiveness in reducing the risk of cardiotoxicity has been confirmed in several clinical trials [Citation37]. Additionally, decreasing doxorubicin dose, especially when administered in liposomal form, has been shown to improve the drug concentration ratio between drug deposition in tumors vs. normal tissues [Citation34]. However, the optimal LTLD dose that maximizes anti-tumor response while minimizing uptake in normal tissues remains unknown. The relationship between tumor dose and systemic dose may not be linear since drug is actively released at the site of heating through intravascular release and diffusion. There might be a possibility that the high concentration of DOX released from LTLD may cause tumor intracellular uptake saturation despite the elevated extracellular concentrations, which would suggest that further increases in administered dose would have diminishing returns on the tumor-to-heart drug concentration ratio (THDCR), defined as the ratio of DOX delivered into the tumor vs. the heart. However, this relationship between the administered LTLD dose and drug deposition in tumor vs. normal tissues has not been comprehensively investigated.
Recently, our group demonstrated that prolonging heating from 10 to 40 min increased DOX concentration in heated tumors without increasing exposure in heart, muscle, liver, kidney, spleen, or lung [Citation38]. In the current study, we use a Vx2 rabbit tumor model to investigate the effect of three different LTLD doses (0.1, 0.5 and 2.5 mg/kg) on DOX delivery to tumors and other healthy organs, the THDCR between tumors and heart, and tumor delivery enhancement (defined as the ratio between the DOX concentration in heated tumor and unheated tumor) due to mild hyperthermia.
Materials and methods
Animal preparation
Female New Zealand white rabbits (3.5–4.5 kg, n = 35) were used in this study. All procedures were approved by the UT Southwestern Institutional Animal Care and Use Committee. For tumor inoculation, 1–2 million Vx2 carcinoma cells suspended in 0.5–1 ml of sterile Hank’s balanced salt solution (HBSS), were injected into both posterior thigh muscles of each rabbit, 12-13 days prior to MR-HIFU treatment.
Animals were assigned randomly into three dose groups: 0.1 mg/kg (n = 10), 0.5 mg/kg (n = 10), and 2.5 mg/kg (n = 7). The other 8 animals were treated, but excluded either due to muscle spasm during the treatment or inadequate heating. On day of treatment, animals with bilateral tumors were anesthetized with a mixture of 2–3.5% isoflurane and 1-2 L/min of 100% oxygen. An intravenous catheter was placed in the ear vein of the rabbit for the administration of drugs and contrast agents. A pulse oximeter (Nonin 8600 V, Plymouth, MN, USA) was attached to the animal’s paw to monitor heart rate, oxygen saturation, and depth of anesthesia. A rectal temperature probe (T1, Neoptix, Quebec, Canada) was used to monitor and record the core body temperature throughout the treatment. Hair over the animal’s thighs was removed using an electric trimmer and depilatory cream. After preparation, the animal was transferred to the MR-HIFU tabletop with a temperature-controlled water bath to maintain the animal’s body temperature during treatment. The animal was positioned on its side, with ultrasound gel applied at the interface between the thigh and the acoustic window, and between the hind legs to avoid undesired reflections of the ultrasound beam. A saline bag was placed between the legs to prevent any heating of the tumor in the contralateral leg. Two extra temperature probes were placed in the water bath and on the animal’s skin. The animals body temperature was between 36 and 37 °C before the treatment started to avoid any unintentional drug release.
LTLD (ThermoDox®, Celsion Corporation, Lawrenceville, NJ, USA) was diluted for injection in an equal volume of sterile 5% dextrose. LTLD with 0.1, 0.5, or 2.5 mg/kg dose was infused into the animal intravenously through the ear vein (22 G angiocath) using an MR-compatible power injector (Medrad Spectris Solaris EP, Bayer Healthcare, Whippany, NJ, USA). The total injection time was 5 min. During the hyperthermia treatment, the infusion was initiated once the average temperature within the targeted location reached 41 °C. Three hours after LTLD infusion, animals were deeply anesthetized and unabsorbed drug was cleared from the vasculature by transcardiac perfusion with saline. Immediately after perfusion was complete, tissues from a variety of organs (liver, lung, kidney, spleen, muscle, heated tumor, unheated tumor, and heart) were collected, snap frozen in liquid nitrogen, and stored at −80°C until ready for further analysis.
MR-HIFU hyperthermia treatment
The experimental protocol was similar to the one described in our previous paper [Citation38] and is illustrated in . Briefly, mild hyperthermia treatment was performed on the tumor in one leg of the rabbit using an MR-HIFU system (Sonalleve V2, Profound Medical Inc, Mississauga, Canada) incorporated into a 3.0 T MRI scanner (Ingenia, Philips Healthcare, Best, The Netherlands). Customized control software was used for mild hyperthermia treatment [Citation19]. The tumor on the contralateral leg served as an unheated control. The output power, sonication frequency, and the duration of the HIFU treatment were 40–60 W, 1.2 MHz, and 40 min, respectively. MR thermometry based on the PRF-shift method with a customized drift correction algorithm [Citation39] was used for monitoring the target temperature during the hyperthermia treatments and continued for 10 min after treatment to observe tissue cooling. The target temperature of the hyperthermia treatment was set to 42.5 °C, which was selected to maintain temperatures above a drug release threshold of 40 °C, while avoiding potentially damaging exposures of greater than 44 °C. Thermal damage was predicted using the concept of thermal dose (measured in cumulative equivalent minutes at 43 °C, CEM43) [Citation40,Citation41]. The thermal dose coverage to 10% (CEM43T10), 50% (CEM43T50) and 90% (CEM43T90) of the target area were calculated. A threshold of 30 CEM43 predicts the initial onset of rabbit muscle tissue damage [Citation42]. To demonstrate the hyperthermia quality, the temporal mean and standard deviation of the spatial mean temperature within the target region (Tmean), the temperature that 10% of the target region exceeds (T10), and the temperature that 90% of the target region exceeds (T90) were calculated.
Figure 1. Time sequence for drug delivery in rabbits with Vx2 tumors using LTLD and MR-HIFU mild hyperthermia. Three different doses of LTLD (0.1, 0.5, and 2.5 mg/kg) were administered in the first 5 min. Temperature mapping was continued for 10 min after treatment to observe tissue cooling (during which period the temperature of the heated region returned to baseline). The heated tumors, contralateral unheated tumor, and other organs were harvested 3 h after the start of LTLD infusion for drug quantification using silver nitrate/chloroform extraction with LC-MS/MS readout.
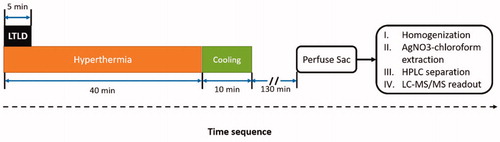
Quantification of DOX concentration in tissue
The DOX quantification protocol used in this study was adopted from a previous published report [Citation38]. Total DOX concentration in tissues, which may include both the released drug and intact LTLDs, was quantified by LC-MS/MS using a high-performance liquid chromatograph (Prominence, Shimadzu Scientific Instruments Inc., Columbia, MD) coupled to a mass spectrometer (AB SCIEX 4000 QTRAP, Applied Biosystems, Foster City, CA). Tissue sample preparation and drug quantification procedures were the same as previously described [Citation38].
Statistical analysis
Differences in the DOX accumulated concentration and DOX uptake efficiency for each tissue type, THDCR, and tumor DOX delivery enhancement were compared among three dosage groups using multiple t-tests with Holm-Sidak correction. A p-value less than 0.05 was considered statistically significant.
Results
shows a representative MR-HIFU mild hyperthermia treatment planning and MR thermometry including images and temperature measurements. As shown in , the three experimental groups had no significant differences in body weight, tumor size, or core body temperature at the beginning of treatment (p > 0.2). After excluding animals with motion during heating, adequate heating (above 40 °C) was achieved for all three groups for the intended duration (40 min) in the target region, with minimum overheating (above 44 °C, <1 min). Similar to our earlier report [Citation38], good mild hyperthermia quality was achieved with this system for all the groups, as summarized in .
Figure 2. Treatment planning and temperature measurements during a 40 min mild hyperthermia treatment. Treatment planning was performed based on T2-weighted MR images indicating the tumor location (A,B). (C) Temperature maps across and along the ultrasound beam show the heating pattern in the middle of the treatment. The treated ROI is indicated by the white circle and the black contour indicates the 42 °C isotherm. The mean temperature, T10, and T90 within the ROI are shown in D confirming stable temperature control at 42 °C during the treatment. The beginning and end of the treatment area marked with the green vertical lines. Scale bar = 2 cm.
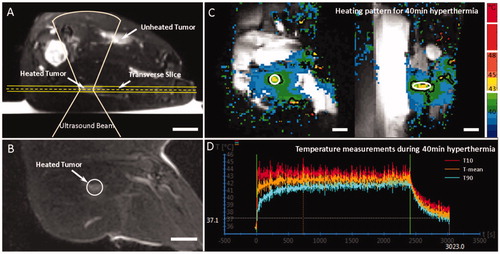
Table 1. Characteristics and heating quality in rabbits administered with LTLD at different doses: 0.1, 0.5 and 2.5 mg/kg, with 40 min MR-HIFU hyperthermia treatment.
shows the dose-dependent DOX accumulation in different organs 3 h after treatment. The limit of quantification (LOQ) of DOX concentration quantification with LC-MS/MS was determined to be 0.2 µg/g in this study for all tissues evaluated (heated/unheated tumor, heart, muscle, liver, kidney, spleen, and lung). With an increase in administered dose of LTLD, there was a significant increase in DOX concentration across all tissues among the three groups (p < 0.05), although the muscle DOX amount at 0.1 mg/kg dose was below LOQ.
Figure 3. DOX accumulated concentration in rabbits treated with different injected doses of LTLD and MR-HIFU hyperthermia. Significantly higher DOX concentration was observed in tumors and other organs with increased injected LTLD. DOX concentration for muscle at 0.1 mg/kg was below the LOQ. *p ≤ 0.05, **p ≤ 0.01, ***p ≤ 0.001, ****p ≤ 0.0001.
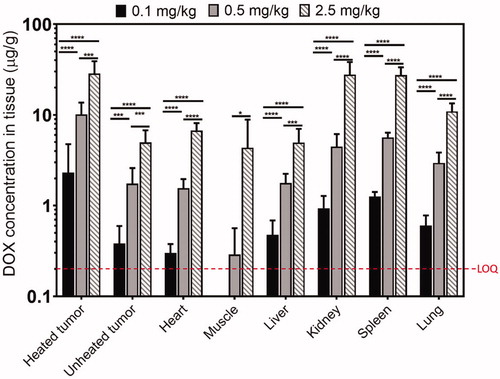
shows the DOX uptake efficiency (defined as the DOX accumulation in a tissue per mg/kg of LTLD injected intravenously) for various tissues, for three different injected doses. No significant difference was found for heated tumor, unheated tumor, heart, muscle, kidney, spleen, and lung across three dose groups, although heated and unheated tumors, and lung showed a trend toward an uptake efficiency decrease at the highest dose (2.5 mg/kg). Liver showed a significant decrease in the fraction of injected dose that accumulated as the administered dose increased (0.1 vs. 2.5 mg/kg: p = 0.03; 0.5 vs. 2.5 mg/kg: p = 0.02).
Figure 4. DOX uptake efficiency, defined as tissue doxorubicin accumulation divided by administered dose, for the three administered doses of LTLD investigated in this study. For organs with constant efficiency across all doses, accumulated DOX is proportional to injected dose. Diminishing returns at higher doses for some tissues might be related to saturation of cellular uptake. Only liver showed a significant difference in the uptake efficiency with increasing dose. Muscle showed an increase in efficiency; however the absolute DOX levels were close to the LOQ at low doses, so this might not be an accurate trend. *p ≤ 0.05. The data labeled with ¤ was from a previous study using a similar Vx2 tumor model that was treated with LTLD (5 mg/kg) plus MR-HIFU hyperthermia (30 min in three heating and cooling cycles) [Citation11].
![Figure 4. DOX uptake efficiency, defined as tissue doxorubicin accumulation divided by administered dose, for the three administered doses of LTLD investigated in this study. For organs with constant efficiency across all doses, accumulated DOX is proportional to injected dose. Diminishing returns at higher doses for some tissues might be related to saturation of cellular uptake. Only liver showed a significant difference in the uptake efficiency with increasing dose. Muscle showed an increase in efficiency; however the absolute DOX levels were close to the LOQ at low doses, so this might not be an accurate trend. *p ≤ 0.05. The data labeled with ¤ was from a previous study using a similar Vx2 tumor model that was treated with LTLD (5 mg/kg) plus MR-HIFU hyperthermia (30 min in three heating and cooling cycles) [Citation11].](/cms/asset/45d9bd2c-be03-41e9-bbca-3b6727d7202e/ihyt_a_1812737_f0004_c.jpg)
The heated tumor had a significant increase in DOX accumulation compared to the contralateral unheated tumor in all dosing groups as shown in (0.1 mg/kg: 9.1 ± 7.7 fold, p = 0.04; 0.5 mg/kg: 8.0 ± 6.1 fold, p = 0.0003; 2.5 mg/kg: 5.8 ± 1.3 fold, p = 0.0004). THDCR defined as the ratio of DOX accumulation in tumors to heart was plotted in . In all three groups the THDCR of unheated tumors was close to one (0.1 mg/kg: 1.4 ± 1.0; 0.5 mg/kg: 1.1 ± 0.5; and 2.5 mg/kg: 0.8 ± 0.3). In the heated tumor, the THDCR had a significant nearly 6-fold increase in all groups (0.1 mg/kg: 7.4 ± 6.4, 5.7 fold increase, p = 0.02; 0.5 mg/kg: 6.9 ± 3.6, 6.3 fold increase, p = 0.0008; and 2.5 mg/kg: 4.4 ± 1.8, 5.5 fold increase, p = 0.0006).
Figure 5. Tumor DOX delivery enhancement with MR-HIFU hyperthermia defined as the ratio of doxorubicin accumulation in heated tumor and unheated tumor for different administered doses of LTLD. A ratio larger than 1 indicating an enhanced drug delivery effect using LTLD combined with mild hyperthermia. There were no significant differences in DOX delivery enhancement among three dose groups, although there was a trend that the enhancement is increasing with lower administered doses.
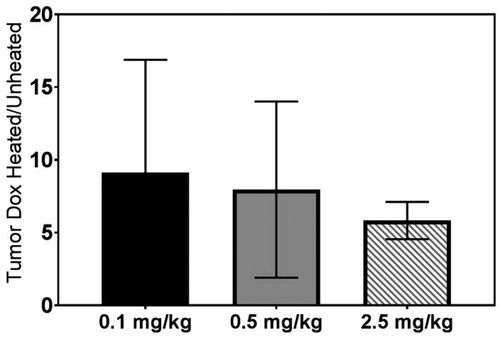
Figure 6. THDCR of DOX accumulation in the tumors and the heart in rabbits treated with different doses of LTLD and MR-HIFU hyperthermia. A nearly 6-fold increase in THDCR index was observed in heated tumors compared to unheated tumors for all groups. The data labeled with ¤ was from a previous study using a similar Vx2 tumor model that was treated with LTLD (5 mg/kg) plus MR-HIFU hyperthermia (30 min in three heating and cooling cycles) [Citation11].
![Figure 6. THDCR of DOX accumulation in the tumors and the heart in rabbits treated with different doses of LTLD and MR-HIFU hyperthermia. A nearly 6-fold increase in THDCR index was observed in heated tumors compared to unheated tumors for all groups. The data labeled with ¤ was from a previous study using a similar Vx2 tumor model that was treated with LTLD (5 mg/kg) plus MR-HIFU hyperthermia (30 min in three heating and cooling cycles) [Citation11].](/cms/asset/a1fe4a3f-7e8a-4885-9abc-1784fbb6b87c/ihyt_a_1812737_f0006_c.jpg)
Discussion
The issue of cardiotoxicity of DOX is especially relevant in pediatric oncology, where patients cured of their disease often have a life expectancy of >20 years. An increased risk of heart failure is correlated to the lifetime exposure to doxorubicin, and these effects can manifest later in life. Thermosensitive liposomes encapsulating DOX (LTLD) offer the potential to maintain reduced cardiotoxicity while achieving increased DOX delivery to heated tumors. This study evaluated the effect of administered LTLD doses on DOX deposition in tumors and other healthy organs.
The DOX uptake efficiency was calculated as the ratio of the accumulated tissue DOX concentration to the injected dose. A higher drug uptake efficiency was observed in heated tumor compared to the heart and unheated tumor across all three doses (). We believe this is due to: 1) increased delivery of DOX to the heated tumor due to direct release of drug into the tumor vessels from thermosensitive liposomes; 2) hyperthermia possibly enhancing the tumor vascular permeability and making it easier for drug to enter the tumor extracellular space; and 3) heat increasing the uptake of DOX by tumor cells, either by increasing cellular membrane permeability [Citation43] or through some other mechanisms. In addition, the results in also showed that the DOX uptake efficiency in heart, kidney, and spleen remained nearly constant as the injected dose increased, indicating a linear relationship between injected dose and DOX accumulation in those three tissues across this dose range.
However, for heated tumor, unheated tumor, liver, and lung, a lower DOX uptake efficiency was observed at the highest dose level (2.5 mg/kg) compared with lower doses, indicating diminishing returns at that dose, possibly due to cellular uptake saturating at higher extracellular concentrations. In a prior study by Ranjan et al. that used a similar rabbit Vx2 tumor model, with the combination of LTLD (5 mg/kg) and MR-HIFU mild hyperthermia (total duration: 30 min, in three heating and cooling cycles), a DOX uptake efficiency of 6.0 ± 1.8 in heated tumor was observed [Citation11], which is smaller than the 11.4 ± 4.2 seen at 2.5 mg/kg in this study (). Despite the slight differences in experimental conditions, this might further indicate the possible saturation effect on the DOX uptake capability at higher doses. If this trend is confirmed, it would be worth considering the use of fractionated low doses of LTLD combined with mild hyperthermia to enhance the therapeutic effect while minimizing the systemic exposure in future clinical applications.
Doxorubicin concentration in tumors and other organs in rabbits was demonstrated to be dose dependent in this study (). A significantly higher doxorubicin concentration was observed with increased doses in all evaluated tissues. When the liposomes release their contents in the tumor microvessels upon heating, the higher the dose they initially contained, the more they will release, making more drug available to transport into the tissue. Drug reaches all the unheated tissues either as free drug in the circulation coming from the released drug in the heated tumor, or as intact liposomes accumulating in the tissue. Since the experimental method measured total doxorubicin, whether free or liposomal, it is clear that a larger initial amount inside the liposomes will lead to higher tissue levels.
Similar to other preclinical/clinical studies, compared to the unheated contralateral tumor, there was a significant intratumoral DOX delivery enhancement (6-9 fold) and a significant THDCR increase (4-7 fold) in the heated tumor with MR-HIFU mild hyperthermia. This held true for all tested LTLD doses in this study. The tumor delivery enhancement due to mild hyperthermia tends to decrease with the dose increase (9.1 ± 7.7 vs. 8.0 ± 6.1 vs. 5.8 ± 1.3 fold for 0.1, 0.5 and 2.5 mg/kg dose, ), however, strong intratumoral variations in measured doxorubicin concentrations at lower doses led to a large standard deviation (SD) and therefore no statistical significance (p > 0.3). This trend toward less enhancement with hyperthermia at higher doses might be due to nonlinearities resulting from the complicated interplay between drug release kinetics, plasma pharmacokinetics, diffusion, and kinetics of cellular uptake. In particular, it is possible that at high doses and high temperatures, extracellular drug concentrations in regions nearer the microvessels reach high enough levels for long enough times to cause intracellular drug uptake to saturate. Previous experimental studies have shown such saturation of cellular uptake of doxorubicin as either extracellular concentration or exposure time are increased [Citation44]. There is evidence that a low dose of DOX can still achieve dramatic tumor cell killing effect in vitro and this killing effect becomes modest when the DOX concentration is above a certain threshold [Citation45]. Therefore, there may be value in further exploration of split dosing of LTLD to exploit these benefits. Meanwhile, there was a trend that the THDCR increased with the dose reduction in the heated tumor (), but no significance was found potentially due to large SD at lower doses (p > 0.4). In the above mentioned study by Ranjan et al., in which they used a LTLD dose of 5 mg/kg in a similar rabbit Vx2 tumor model combined with MR-HIFU hyperthermia (30 min), the THDCR in heated tumor was 2.7 ± 0.3 as indicated in . Despite the differences in the experimental conditions, the trend seems to be held in different studies. It will be important to explore whether the trend toward decreased enhancement and THDCR with heating at higher LTLD doses seen in our in vivo data is observed in patients and thus whether fractionated doses of LTLD might be of benefit. Note that the plasma concentrations may not scale linearly with the administered dose, and those may be more directly related to cardiac and hematological toxicities. Herein, when comparing the concentration of DOX accumulated in the tumor and heart, the term “THDCR” was defined and used instead of the term “therapeutic ratio” in our prior studies [Citation38], because it describes the metric more precisely.
In a recent study by Besse et al. [Citation46], tumor growth, survival, and qualitative drug distribution were evaluated for three injected doses (2.5, 5, 10 mg/kg) of LTLD combined with 1 h water bath mild hyperthermia (42 °C) and two other DOX formulations without hyperthermia on a mice human fibrosarcoma tumor model. No significant differences in survival were observed in the LTLD plus hyperthermia group among three injected doses. In addition, no significant difference was found in the tumor growth between 5 and 10 mg/kg dose groups and they hypothesized the efficacy of LTLD does not increase at doses higher than 5 mg/kg. They also found a low dose of LTLD (2.5 compared to 5 or 10 mg/kg) was beneficial to the toxicity indicated by no weight loss. These findings support our observations suggesting therapeutic benefits associated with administering low doses of LTLD when combining with mild hyperthermia in tumor treatments. Benefits may be further enhanced by using the longest practicable heating durations [Citation38].
In summary, we have demonstrated that tissue DOX concentrations achieved with LTLD combined with MR-HIFU mild hyperthermia are dose-dependent in a Vx2 tumor rabbit model, including heated/unheated tumor, heart, and other healthy organs. Higher DOX accumulation and THDCR were observed in heated tumors compared to unheated tumors in all three tested doses. The DOX uptake efficiency with respect to the LTLD dose of heated tumor was significantly higher than that of unheated tumor and heart within the tested dose range (0.1-2.5 mg/kg). The DOX uptake for the heart linearly scaled up as a function of dose while that for the heated tumor showed some evidence of saturation at the high dose of 2.5 mg/kg. These results provide guidance on clinical protocol design of hyperthermia-triggered drug delivery.
Acknowledgements
The authors thank Mr. Cecil Futch, Mr. Joris Nofiele, and Miss Yu Hong for their help in the experiment.
Disclosure statement
Robert M Staruch is a paid employee of Profound Medical Inc. Rajiv Chopra is a shareholder of Profound Medical Inc. Celsion Corporation provided the thermo-sensitive liposomal doxorubicin for this study as an in-kind contribution.
Additional information
Funding
References
- de Smet M, Heijman E, Langereis S, et al. Magnetic resonance imaging of high intensity focused ultrasound mediated drug delivery from temperature-sensitive liposomes: an in vivo proof-of-concept study. J Control Release. 2011;150(1):102–110.
- Staruch R, Chopra R, Hynynen K. Localised drug release using MRI-controlled focused ultrasound hyperthermia. Int J Hyperth. 2011;27(2):156–171.
- Dabbagh A, Abdullah BJJ, Abdullah H, et al. Triggering mechanisms of thermosensitive nanoparticles under hyperthermia condition. J Pharm Sci. 2015;104(8):2414–2428.
- Gasselhuber A, Dreher MR, Partanen A, et al. Targeted drug delivery by high intensity focused ultrasound mediated hyperthermia combined with temperature-sensitive liposomes: computational modelling and preliminary in vivovalidation. Int J Hyperthermia. 2012;28(4):337–348.
- Hijnen N, Kneepkens E, de Smet M, et al. Thermal combination therapies for local drug delivery by magnetic resonance-guided high-intensity focused ultrasound. Proc Natl Acad Sci USA. 2017;114(24):E4802–E4811.
- Staruch R, Chopra R, Hynynen K. Hyperthermia in bone generated with MR imaging-controlled focused ultrasound: control strategies and drug delivery. Radiology. 2012;263(1):117–127.
- Hijnen N, Langereis S, Grüll H. Magnetic resonance guided high-intensity focused ultrasound for image-guided temperature-induced drug delivery. Adv Drug Deliv Rev. 2014;72:65–81.
- Bing C, Nofiele J, Staruch R, et al. Localised hyperthermia in rodent models using an MRI-compatible high-intensity focused ultrasound system. Int J Hyperthermia. 2015;31(8):813–822.
- Lyon PC, Gray MD, Mannaris C, et al. Safety and feasibility of ultrasound-triggered targeted drug delivery of doxorubicin from thermosensitive liposomes in liver tumours (TARDOX): a single-centre, open-label, phase 1 trial. Lancet Oncol. 2018;19(8):1027–1039.
- Partanen A, Yarmolenko PS, Viitala A, et al. Mild hyperthermia with magnetic resonance-guided high-intensity focused ultrasound for applications in drug delivery. Int J Hyperthermia. 2012;28(4):320–336.
- Ranjan A, Jacobs GC, Woods DL, et al. Image-guided drug delivery with magnetic resonance guided high intensity focused ultrasound and temperature sensitive liposomes in a rabbit Vx2 tumor model. J Control Release. 2012;158(3):487–494.
- Quesson B, de Zwart JA, Moonen CT. Magnetic resonance temperature imaging for guidance of thermotherapy. J Magn Reson Imaging. 2000;12(4):525–533.
- Aubry J-F, Pauly KB, Moonen C, et al. The road to clinical use of high-intensity focused ultrasound for liver cancer: Technical and clinical consensus. J Ther Ultrasound. 2013;1:13.
- Bing C, Cheng B, Staruch RM, et al. Breath-hold MR-HIFU hyperthermia: phantom and in vivo feasibility. Int J Hyperthermia. 2019;36(1):1084–1097.
- Elias WJ, Lipsman N, Ondo WG, et al. A randomized trial of focused ultrasound thalamotomy for essential tremor. N Engl J Med. 2016;375(8):730–739.
- Siddiqui K, Chopra R, Vedula S, et al. MRI-guided transurethral ultrasound therapy of the prostate gland using real-time thermal mapping: initial studies. Urology. 2010;76(6):1506–1511.
- Schmitz AC, Gianfelice D, Daniel BL, et al. Image-guided focused ultrasound ablation of breast cancer: current status, challenges, and future directions. Eur Radiol. 2008;18(7):1431–1441.
- Rabinovici J, Inbar Y, Revel A, et al. Clinical improvement and shrinkage of uterine fibroids after thermal ablation by magnetic resonance-guided focused ultrasound surgery. Ultrasound Obstet Gynecol. 2007;30(5):771–777.
- Tillander M, Hokland S, Koskela J, et al. High intensity focused ultrasound induced in vivo large volume hyperthermia under 3D MRI temperature control. Med Phys. 2016;43(3):1539–1549.
- Needham D, Dewhirst MW. The development and testing of a new temperature-sensitive drug delivery system for the treatment of solid tumors. Adv Drug Deliv Rev. 2001;53(3):285–305.
- Poon RT, Borys N. Lyso-thermosensitive liposomal doxorubicin: an adjuvant to increase the cure rate of radiofrequency ablation in liver cancer. Future Oncol. 2011;7(8):937–945.
- Tak WY, Lin S-M, Wang Y, et al. Phase III HEAT study adding lyso-thermosensitive liposomal doxorubicin to radiofrequency ablation in patients with unresectable hepatocellular carcinoma lesions. Clin Cancer Res. 2018;24(1):73–83.
- Lyon PC, Griffiths LF, Lee J, et al. Clinical trial protocol for TARDOX: a phase I study to investigate the feasibility of targeted release of lyso-thermosensitive liposomal doxorubicin (ThermoDox®) using focused ultrasound in patients with liver tumours. J Ther Ultrasound. 2017;5:28.
- Wood BJ, Poon RT, Locklin JK, et al. Phase I study of heat-deployed liposomal doxorubicin during radiofrequency ablation for hepatic malignancies. J Vasc Interv Radiol. 2012;23(2):248–255.e7.
- Landon CD, Park J-Y, Needham D, et al. Nanoscale drug delivery and hyperthermia: the materials design and preclinical and clinical testing of low temperature-sensitive liposomes used in combination with mild hyperthermia in the treatment of local cancer. Open Nanomed J. 2011;3:38–37.
- Dromi S, Frenkel V, Luk A, et al. Pulsed-high intensity focused ultrasound and low temperature-sensitive liposomes for enhanced targeted drug delivery and antitumor effect. Clin Cancer Res. 2007;13(9):2722–2727.
- Staruch RM, Hynynen K, Chopra R. Hyperthermia-mediated doxorubicin release from thermosensitive liposomes using MR-HIFU: therapeutic effect in rabbit Vx2 tumours. Int J Hyperthermia. 2015;31(2):118–133.
- Staruch RM, Ganguly M, Tannock IF, et al. Enhanced drug delivery in rabbit VX2 tumours using thermosensitive liposomes and MRI-controlled focused ultrasound hyperthermia. Int J Hyperthermia. 2012;28(8):776–787.
- Gray MD, Lyon PC, Mannaris C, et al. Focused ultrasound hyperthermia for targeted drug release from thermosensitive liposomes: results from a phase I trial. Radiology. 2019;291(1):232–238.
- Zagar TM, Vujaskovic Z, Formenti S, et al. Two phase I dose-escalation/pharmacokinetics studies of low temperature liposomal doxorubicin (LTLD) and mild local hyperthermia in heavily pretreated patients with local regionally recurrent breast cancer. Int J Hyperthermia. 2014;30(5):285–294.
- Geisberg CA, Sawyer DB. Mechanisms of anthracycline cardiotoxicity and strategies to decrease cardiac damage. Curr Hypertens Rep. 2010;12(6):404–410.
- Chatterjee K, Zhang J, Honbo N, et al. Doxorubicin cardiomyopathy. Cardiology. 2010;115(2):155–162.
- QuanJun Y, GenJin Y, LiLi W, et al. Protective effects of dexrazoxane against doxorubicin-induced cardiotoxicity: a metabolomic study. PLoS One. 2017;12(1):e0169567.
- Sadzuka Y, Nakai S, Miyagishima A, et al. The effect of dose on the distribution of adriamycin encapsulated in polyethyleneglycol-coated liposomes. J Drug Target. 1995;3(1):31–37.
- Peters JH, Gordon GR, Kashiwase D, et al. Tissue distribution of doxorubicin and doxorubicinol in rats receiving multiple doses of doxorubicin. Cancer Chemother Pharmacol. 1981;7(1):65–69.
- Pacciarini MA, Barbieri B, Colombo T, et al. Distribution and antitumor activity of adriamycin given in a high-dose and a repeated low-dose schedule to mice. Cancer Treat. Rep. 1978;62:791–800.
- O’Brien MER, Wigler N, Inbar M, et al. Reduced cardiotoxicity and comparable efficacy in a phase III trial of pegylated liposomal doxorubicin HCl (CAELYXTM/Doxil®) versus conventional doxorubicin for first-line treatment of metastatic breast cancer. Ann. Oncol. 2004;15(3):440–449.
- Bing C, Patel P, Staruch RM, et al. Longer heating duration increases localized doxorubicin deposition and therapeutic index in Vx2 tumors using MR-HIFU mild hyperthermia and thermosensitive liposomal doxorubicin. Int J Hyperth. 2018;36(1):196–203.
- Bing C, Staruch RM, Tillander M, et al. Drift correction for accurate PRF-shift MR thermometry during mild hyperthermia treatments with MR-HIFU. Int J Hyperthermia. 2016;32(6):673–687.
- Sapareto SA, Dewey WC. Thermal dose determination in cancer therapy. Int J Radiat Oncol Biol Phys. 1984;10(6):787–800.
- Perez CA, Sapareto SA. Thermal dose expression in clinical hyperthermia and correlation with tumor response/control. Cancer Res. 1984;44(10 Suppl):4818s–4825s.
- McDannold NJ, King RL, Jolesz FA, et al. Usefulness of MR imaging-derived thermometry and dosimetry in determining the threshold for tissue damage induced by thermal surgery in rabbits. Radiology. 2000;216(2):517–523.
- Hayat H, Friedberg I. Heat-induced alterations in cell membrane permeability and cell inactivation of transformed mouse fibroblasts. Int J Hyperthermia. 1986;2(4):369–378.
- Kerr DJ, Kerr AM, Freshney RI, et al. Comparative intracellular uptake of adriamycin and 4’-deoxydoxorubicin by nonsmall cell lung tumor cells in culture and its relationship to cell survival. Biochem Pharmacol. 1986;35(16):2817–2823.
- Sharma A, Özayral S, Caserto JS, et al. Increased uptake of doxorubicin by cells undergoing heat stress does not explain its synergistic cytotoxicity with hyperthermia. Int J Hyperth. 2019;36:712–720.
- Besse H, Barten-van Rijbroek A, van der Wurff-Jacobs K, et al. Tumor drug distribution after local drug delivery by hyperthermia, in vivo. Cancers (Basel). 2019;11(10):1512.