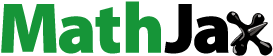
Abstract
Purpose
To evaluate the antitumor efficacy in local and distant tumors induced by local hyperthermia with CTLA-4 blockade
Methods
A mouse breast cancer cell line was inoculated into both sides of the legs of mice. The mice were treated with three administrations of CTLA-4 blockade, a single application of local hyperthermia (42.5 °C for 20 min) to the tumor on one side of the leg, or the combination of the two. Tumor growth in locally heated tumors (HT tumors) and unheated distant tumors (UnHT tumors) and overall survival were evaluated.
Results
In the combination group, tumor volume significantly decreased for both HT and UnHT tumors compared with the tumors in the untreated and local hyperthermia monotherapy groups. Remarkable efficacy was only observed in the combination therapy group, in which 7 of 18 mice responded to HT and UnHT tumors, with significant prolonged overall survival.
Conclusions
Combination therapy enhanced the antitumor response not only in HT tumors but also in UnHT tumors and prolonged overall survival.
Introduction
Breast cancer is a high morbidity disease in women due to the common development of distant metastases [Citation1–3]. The development of therapeutic methods for controlling metastasis has remained a challenging issue for decades. Local hyperthermia (local HT) has been used to treat breast cancer and other locally advanced neoplasms in combination with radiation therapy (RT) or chemotherapy as tumors are more susceptible to heat compared with normal tissues [Citation4–8]. While normal tissues can release heat via blood vessel expansion, the angiogenic vessels in tumors are more likely to accumulate heat within themselves, eventually leading to necrotic and apoptotic cancer cell death [Citation9,Citation10]. Previous studies have reported that local HT induces antitumor immune responses via several mechanisms, including the expression of heat shock protein 70 (HSP70) on cell surfaces or its release into the extracellular milieu as well as a direct cell-killing effect [Citation10–16]. However, no study to date has reported that local HT monotherapy can successfully inhibit distant metastases.
Recently, immune checkpoint inhibitors (ICI), such as anti-cytotoxic T-lymphocyte antigen protein 4 (CTLA-4) and anti-programmed cell death protein 1 (PD-1)/programmed death-ligand 1 (PD-L1) antibodies, have gained attention for the treatment of some types of tumors, such as melanoma, lung, bladder, and head and neck cancers [Citation17]. CTLA-4, a cell surface receptor, is expressed on activated T cells and regulatory T cells (Treg), leading to the inhibition of T cell function [Citation17–19]. Therefore, CTLA-4 blockade (C4) enhances the antitumor immune response [Citation18,Citation19]. Although C4 monotherapy in preclinical and clinical studies has demonstrated a durable long-term response in patients with metastatic tumors, only a small percentage of patients respond to this treatment, indicating that the combination of other treatments, including RT, chemotherapy, and local HT, is crucial for achieving a broader response [Citation20–26]. However, the effect of local HT in combination with C4 therapy on the antitumor immune response and the immune microenvironment in local and distant tumors has not been fully elucidated.
In the present study, we demonstrated that this combination therapy enhances not only the local antitumor response but also the distant antitumor response, known as the abscopal effect.
Materials and methods
Ethics statement
All protocols for animal experiments were approved by the Osaka University Institutional Animal Care and Use Committee (30-013-004). For local HT, mice were immobilized on an in-house jig under anesthesia with 30 mg/kg pentobarbital via intraperitoneal (i.p.) injection.
Mice were sacrificed via CO2 inhalation when they met the humane endpoint criteria, which were prostration, breathing difficulties, or when the longest dimension of the tumor was ≥ 20 mm.
Cell lines
The mouse breast cancer cell line 4T1 was purchased from American Type Culture Collection (Manassas, VA). The cells were maintained in RPMI1640 supplemented with 10% fetal bovine serum (FBS), 5 mM penicillin/streptomycin, and 1% L-glutamine at 37 °C in a 5% CO2 atmospheric incubator.
RF hyperthermia
We used an RF-I.V. local HT device (Yamamoto Vinita Co. Ltd., Osaka, Japan), which allows for local heating of cells or rodents, with a radiofrequency of 8 MHz ().
Figure 1. The device used for local RF-induced hyperthermia treatment and heating methods for in vitro and in vivo experiments. (A) In vitro experimental setup. (B) In vivo experimental setup. The tumor on one side of the leg was heated at 42.5 °C for 20 min under anesthesia. The temperature on the tumor surface was monitored during heating.
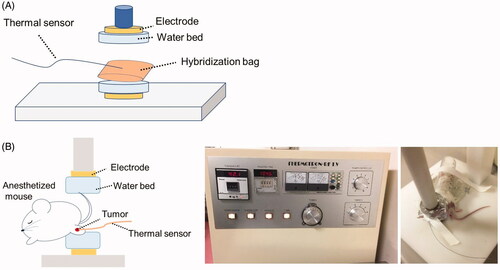
For in vitro experiments, a hybridization bag filled with 5 × 105 4T1 cells in 2 ml of RPMI 1640 medium was placed between two electrodes and then heated at 37 °C (N = 3), 41 °C (N = 3), 42.5 °C (N = 3), or 43.5 °C (N = 3) for 30 min (). To ensure that the temperature was consistent throughout the procedure, a thermal sensor was placed on the hybridization bag.
For in vivo experiments, one leg of the mouse was placed between two electrodes under anesthesia and local HT was applied at 42.5 °C for 20 min to the tumor on one side of the leg by holding the tumor between electrode plates (). The temperature at the tumor surface was monitored during local HT using an equipped thermal sensor. The temperature standard deviation during local HT was within 0.01 °C (Supplementary Figure 1).
Colony formation assay
Immediately after heating at 37 °C (N = 3), 41 °C (N = 3), 42.5 °C (N = 3), or 43.5 °C (N = 3) for 30 min, cells were trypsynized and seeded into φ60 dishes. After incubation for 2 weeks, the cells were fixed with a 10% formaldehyde solution and then stained with a crystal violet solution. The number of colonies containing at least 50 cells was counted to analyze the survival fraction.
In vivo experiments
Six- to eight-week-old male BALB/cAJcl mice were purchased from Nihon-Clea (Tokyo, Japan) and maintained in a specific pathogen-free area at Osaka University.
The overall experimental scheme is shown in . We subcutaneously inoculated 1 × 105 4T1 cells into both sides of the leg of each mouse. Treatments were initiated when the tumor length reached 5 mm (Day 1). The mice were divided into four groups: an untreated group (NoTx group, N = 16); a group receiving 150 µg of C4 via i.p. injection (Clone: 9H10, BioXCell, NH, USA) on days 1, 4, and 7 (C4 group, N = 17); a group receiving local HT to a tumor on one side of the leg on day 4 (HT group, N = 19); and a combination group receiving both local HT and the same dose of C4 via i.p. injection (HT + C4 group, N = 18).
Figure 2. In vivo experimental scheme. (A) Scheme of tumor volume analysis. BALB/c mice were divided into the NoTx (N = 16), HT (N = 19), C4 (N = 17) and HT + C4 (N = 18) groups. Treatments were initiated on day 1, when the average of the longest length and the perpendicular length in tumors reached 5 mm. (B) Experimental scheme for the analysis of lung metastasis. Lungs were harvested from mice in the C4 (N = 7) and HT + C4 (N = 9) groups on day 13. (C) Experimental scheme of FTY720 treatment. FTY720 was injected via i.p. on day 0 and repeated thereafter every 2 days until day 18. Tumor volumes and survival rates were compared between the HT + C4 + FTY720 (N = 12) and HT + C4 groups (N = 24). Abbreviations. InOc: Inoculation; HT: Hyperthermia; C4: CTLA-4 blockade; FTY: Fingolimod.
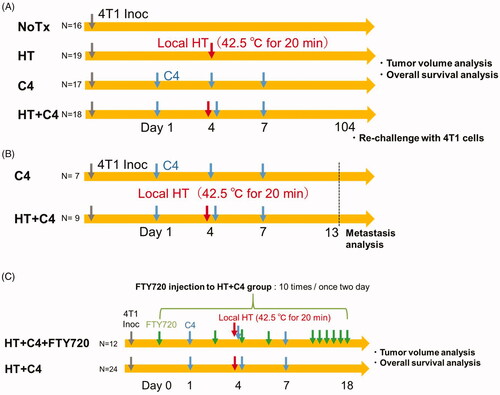
Tumor rechallenge was conducted for those mice who got cured in the HT + C4 group by subcutaneously inoculating 1 × 105 4T1 cells into both sides of the legs.
To analyze lung metastasis, we harvested the lungs from mice in the C4 (N = 7) and HT + C4 (N = 9) groups on day 13 and counted the number of metastatic nodules using a stereoscopic microscope (SZX12; Olympus, Tokyo, Japan) ().
Fingolimod (FTY720) (Cayman CHEMICAL, MI, USA), an immunosuppressive agent derived from ISP-1 (myriocin), prevents lymphocyte egress into the peripheral blood [Citation27,Citation28]. We intraperitoneally administered approximately 5 µg FTY720 dissolved in saline (50 µg/ml) into the mice in the combination therapy group (HT + C4 + FTY720 group) every 2 days from day 0 to day 18. Tumor volumes and survival rates were compared between the HT + C4 + FTY720 (N = 12) and HT + C4 groups (N = 24) ().
Tumor growth analysis
For tumor growth studies, tumor length (L) and width (W) were measured at least every 3 days. Tumor volume was calculated using EquationEquation (1)(1)
(1) as follows:
(1)
(1)
Furthermore, the number of animals that responded to the treatments between the C4 and C4 + HT groups was analyzed, wherein responders were defined as follows:
Statistical analysis
Differences in 4T1 cell survival between each temperature treatment were evaluated using the Tukey–Kramer honestly significant difference test. Tumor volume differences between the NoTx, HT, C4, and HT + C4 groups was compared using the Steel–Dwass test. The Wilcoxon rank-sum test was used to compare tumor volume differences between the HT + C4 + FTY720 and HT + C4 groups. Fisher’s exact test was used to compare the number of treatment-responsive mice between the C4 and HT + C4 groups. The Student’s t-test was used to compare the number of metastatic nodules in the lungs between the C4 and HT + C4 groups. The Kaplan–Meier method was used to evaluate overall survival, and statistical significance was determined using the log-rank test.
Results
Colony formation assay
The survival fraction of 4T1 cells decreased in a temperature-dependent manner (). Survival fractions at 41 °C, 42.5 °C, and 43.5 °C were 57%, 38%, and 19%, respectively.
Effect of local HT on the antitumor response in HT tumors
Although mice in both the NoTx and HT groups showed rapid tumor growth, significant tumor growth suppression was observed in the HT + C4 group compared with that in the NoTx (p = 0.017) and HT (p = 0.040) groups (). On the other hand, only slight inhibition in tumor volume was observed in the C4 group, indicating no significant difference compared with the NoTx (p = 0.291) and HT (p = 0.345) groups ().
Figure 4. Effect of local HT on the antitumor response in locally heated (HT) tumors. (A) Tumor growth curves for the HT tumors (NoTx: N = 16, HT: N = 19, C4: N = 17, and HT + C4: N = 18). (B) Quantitative analysis of tumor volume change on day 28. (C) Number of mice who responded in the C4 and HT + C4 groups. A responder is defined as a mouse with a smaller tumor volume on day 28 than on day 1. Data are combined from two independent experiments. Error bars represent SEM. *p < 0.05.; **p < 0.01. Abbreviations. Inoc: Inoculation; HT: Hyperthermia; C4: CTLA-4 blockade.
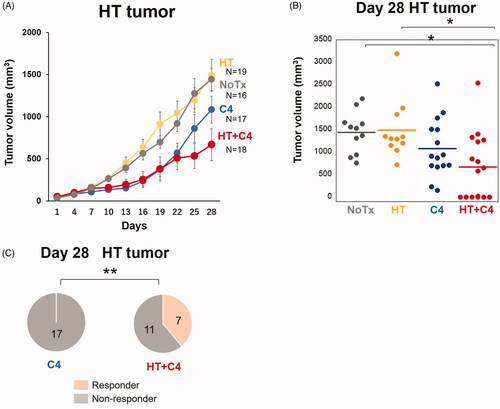
Notably, 7 of 18 mice with HT tumors (39%) responded to the treatment in the HT + C4 group (p=0.009), whereas no mice responded to the treatment in the C4 group = 0.009) ().
Effect of local HT on abscopal tumors
The growth of UnHT tumors in the HT + C4 group was significantly inhibited compared with the growth of UnHT tumors in the NoTx (p = 0.023) and HT (p = 0.006) groups (). In contrast, the mice in the C4 group experienced slight inhibition compared with that in the NoTx (p = 0.291) and HT (p = 0.080) groups, which did not reach statistical significance. Notably, 7 of 18 mice with UnHT tumors (39%) responded to the treatment in the HT + C4 group (p=0.009) (), whereas C4 monotherapy failed to induce responders.
Figure 5. Effect of local HT on the abscopal effect. (A) Tumor growth curves for UnHT tumors (NoTx: N = 16, HT: N = 19, C4: N = 17, HT + C4: N = 18). (B) Distribution of the tumor volume in the mice of each group on day 28. (C) Number of responders in the C4 and HT + C4 groups. A responder is defined as a mouse with a smaller tumor volume on day 28 than on day 1. Data are combined between two independent experiments. Error bars represent SEM. *p < 0.05, **p < 0.01. Abbreviations. HT: Hyperthermia; C4: CTLA-4 blockade.
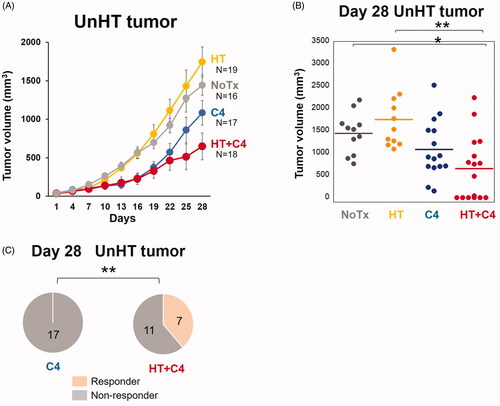
Local HT in combination with C4 inhibits lung metastasis and prolonged overall survival
We evaluated the number of gross metastatic nodules in the lungs in the C4 and HT + C4 groups on day 13 and overall survival based on the same treatment regimen ().
Evaluation of the number of gross metastatic nodules in the lungs revealed that combination therapy had a stronger ability to inhibit metastasis than C4 monotherapy (p = 0.040) ().
Figure 6. Local HT with C4 inhibits lung metastasis and prolongs overall survival. (A) Representative graphs of the metastases in the C4 and HT + C4 groups. (B) The number of metastases in the lungs in the C4 (N = 7) and HT + C4 (N = 9) groups at day 13. (C) Overall survival of the mice in the C4 group (N = 17) and the HT + C4 group (N = 18). Data are combined between two independent experiments. (D) Experimental scheme for the analysis of rechallenge with 4T1 cell inoculations. (E) Overall survival after rechallenge with 4T1 cell inoculations in the five cured mice in the HT + C4 group. Error bars represent SEM. *p < 0.05. HT: Hyperthermia; C4: CTLA-4 blockade.

Although local HT monotherapy did not prolong overall survival (Supplementary Figure 2), compared with the NoTx group, combination therapy significantly prolonged overall survival compared with the C4 group (). Median survivals were 33 and 38 days for mice in the C4 and HT + C4 groups, respectively. The longest survival rate was 50 days in the C4 group, whereas 6 out of 18 mice (33%) in the HT + C4 group survived for more than 80 days (p = 0.024). The rechallenge of 4T1 cells to bilateral legs in the five cured mice revealed that the tumors were rejected in all mice ().
Abscopal effect was due to lymphocytes trafficking to tumors in the combination therapy
To examine whether the observed abscopal effect and the prolonged survival in the HT + C4 group was due to lymphocyte trafficking to the peripheral tumors, we evaluated tumor volumes and overall survival using FTY720.
Although tumor volume changes in HT tumors were identical in both the HT + C4 and HT + C4 + FTY720 groups (), the addition of FTY720 abolished tumor growth delay (p = 0.001) () and increased survival benefit (p = 0.0006) (). Notably, 22 of 24 mice (92%) in the HT + C4 group survived till day 28. In contrast, only 3 of 12 mice (25%) in the HT + C4 + FTY720 group survived till day 28, which was significantly less than those in HT + C4 group (). These results suggest that lymphocyte trafficking to peripheral tumors is associated with the observed abscopal effect and even with a survival benefit.
Figure 7. Tumor growth and overall survival in the HT + C4 + FTY720 (N = 12) and HT + C4 (N = 24) groups. (A) Tumor growth curves in HT tumors. (B) Tumor growth curves in UnHT tumors. (C) Overall survival. Data are combined from two independent experiments. Error bars represent SEM. **p < 0.01. Abbreviations. HT: Hyperthermia; C4: CTLA-4 blockade; FTY720: Fingolimod.
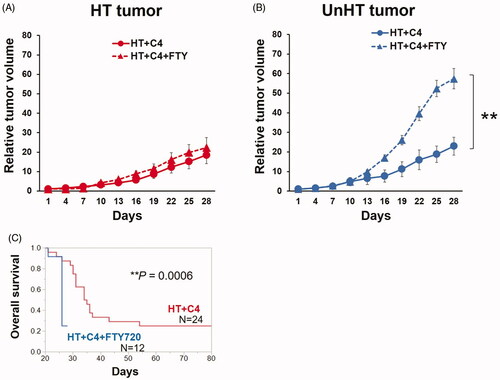
Discussion
Although no preclinical and clinical evidence suggests that local HT has the ability to induce a systemic antitumor response, recent studies have demonstrated that antitumor immunity is stimulated by local HT via HSP induction [Citation10,Citation12,Citation29]. A recent study reported that exosomes released from breast cancer cells treated with HT possessed an immunogenic potential [Citation30]. Together with the strong enhancement of T cell activity and Treg blocking function by C4 [Citation17], we hypothesized that local HT in combination with C4 could enhance systemic antitumor efficacy and alter immune cell microenvironments not only in local tumors but also in distant tumors.
To determine the heating temperature for in vivo experiments, we conducted the colony formation assay using 4T1 cells heated at four different temperatures for 30 min. Consistent with the results of previous reports using Chinese hamster ovary cell lines [Citation31], our data revealed that heating at ≥42.5 °C significantly reduced survival compared with heating at 37 °C. To avoid serious adverse effects in mice, we selected 42.5 °C for further in vivo experiments.
By heating at this temperature for 20 min, we found that local HT failed to inhibit local tumor growth; however, the addition of C4 significantly enhanced the antitumor response. Surprisingly, the combined effect was not limited to the local tumor but was expanded to distant sites, as evidenced by the inhibition of abscopal tumor growth, lung metastasis, and prolonged overall survival. Recently, several groups have reported the combined effects of local HT with ICI. Ando et al. reported that the addition of C4 to local HT at 42.5 °C for 60 min in a water bath did not significantly inhibit the growth of HT tumors compared with local HT monotherapy in EL-4 lymphoma-bearing mice [Citation32]. Chao et al. reported that magnetic HT at 50 °C for 10 min with PD-L1 blockade and poly (lactic-co-glycolic) acid R837 nanoparticles induced both local and abscopal effects in back skin tumors in B16-bearing mice [Citation33]. Although there has been an increase in preclinical evidence for the use of local HT in combination with ICI, to the best of our knowledge, this is the first report that has demonstrated the effects of local HT in combination with C4 in a bilateral breast cancer-bearing model, allowing us to evaluate the abscopal tumor volume changes over time in detail under the same principles and temperatures as are widely used in clinics [Citation34]. More recently, Oei et al. also reported that a significant antitumor efficacy was only found in locally-heated tumors but not in lung metastases, in combination with a dual ICI (43 °C for 20 min with anti-PD-1 and anti-CTLA-4 antibodies; P1C4) using magnetic iron oxide nanoparticle HT in 4T1-bearing mice [Citation35]. In contrast, our data revealed that even the use of a single immune checkpoint blockade with local HT enhanced both local and abscopal effects, together with the inhibition of lung metastasis and prolonged overall survival. One of the possible reasons for the discrepancy in the antitumor efficacy between our study and the study of Oei et al. is the difference in tumor volume at the initiation of the treatments. In their treatment regimen, treatments were initiated when the primary tumor volume reached 100 mm3. In contrast, in the present study, treatments were initiated when the tumor volume reached 65 mm3, suggesting that the abscopal effect is less likely to occur when the primary tumor volume is large (e.g., >100 mm3).
Increasing preclinical evidence suggests that the combination of RT and ICI enhances the abscopal effect as well as the local effect, leading to strong inhibition in distant tumor volume and prolongation of survival, with immune microenvironment changes in irradiated and abscopal tumors [Citation26,Citation35–39]. Therefore, the combination of RT and local HT with C4 for large tumors is likely to have a greater impact on the abscopal effect. Further studies will be needed to explore strategies to obtain an improved antitumor response.
Finally, we evaluated tumor growth and survival in HT + C4-treated mice with or without FTY720 administration. Our data revealed that no significant difference in HT tumor volume change was observed between the HT + C4 + FTY720 and HT + C4 groups, suggesting that not only the immune response but also physical damage by local HT has an impact on HT tumors to some extent. Importantly, UnHT tumor growth increased even more rapidly in the HT + C4 + FTY720 group than in the HT + C4 group. Furthermore, prolonged overall survival in the HT + C4 group was abolished by the addition of FTY720, suggesting that the abscopal effect was significantly affected by lymphocyte trafficking to the peripheral tumors. Further detailed studies are necessary to identify the immune microenvironment changes, specific immune cells (e.g., cytotoxic T cells, helper T cells, NK cells, NK T cells) and cytokine release, which contribute to the abscopal effect in HT with C4 therapy.
In conclusion, we found that local HT in combination with C4 inhibited tumor growth not only in HT tumors but also in distant tumors, leading to prolonged overall survival in a murine breast cancer model. This treatment efficacy was not achieved using C4 monotherapy. Although we used a highly metastatic mouse breast cancer cell line to observe the abscopal effect, it remains unclear whether the abscopal effect is induced in other cancer cell lines. Future study is warranted to address this issue.
Supplemental Material
Download PDF (65.8 KB)Acknowledgments
This study was supported by the Center for Medical Research and Education, Graduate School of Medicine, Osaka University. We thank S. Katsuki and J. Yamamoto in the Department of Medical Physics and Engineering at Osaka University for their technical support.
Disclosure statement
The authors declare that they have no conflicts of interest and that only these authors are responsible for the contents of this manuscript.
Additional information
Funding
References
- Akinyemiju TF, Al Lami FH, Alam T, et al. Global, regional, and national cancer incidence, mortality, years of life lost, years lived with disability, and disability-adjusted life-years for 29 cancer groups, 1990 to 2016: a systematic analysis for the global burden of disease study. JAMA Oncol. 2018;4(11):1553–1568.
- Weigelt B, Peterse JL, van’t Veer LJ. Breast cancer metastasis: markers and models. Nat Rev Cancer. 2005;5(8):591–602.
- Swain SM, Sorace RA, Bagley CS, et al. Neoadjuvant chemotherapy in the combined modality approach of locally advanced nonmetastatic breast cancer. Cancer Res. 1987;47(14):3889–3894.
- Overgaard J, Gonzalez DG, Hulshof MC, et al. Randomised trial of hyperthermia as adjuvant to radiotherapy for recurrent or metastatic malignant melanoma. European Society for Hyperthermic Oncology. Lancet. 1995;345(8949):540–543.
- Masunaga S, Hiraoka M, Takahashi M, et al. Clinical results of thermoradiotherapy for locally advanced and/or recurrent breast cancer-comparison of results with radiotherapy alone. Int J Hyperthermia. 1990;6(3):487–497.
- Costa MA, Fardilha C, Fonseca G, et al. Radiotherapy and hyperthermia in the treatment of locoregional recurrent cancer-Report of the first patients treated in portugal. Sci J Clin Med. 2019;8(1):1–5.
- Ohtake M, Umemura M, Sato I, et al. Hyperthermia and chemotherapy using Fe(Salen) nanoparticles might impact glioblastoma treatment. Sci Rep. 2017;7:42783.
- Vujaskovic Z, Kim DW, Jones E, et al. A phase I/II study of neoadjuvant liposomal doxorubicin, paclitaxel, and hyperthermia in locally advanced breast cancer. Int J Hyperthermia. 2010;26(5):514–521.
- Dudar TE, Jain RK. Differential response of normal and tumor microcirculation to hyperthermia. Cancer Res. 1984;44(2):605–612.
- Toraya-Brown S, Fiering S. Local tumour hyperthermia as immunotherapy for metastatic cancer. Int J Hyperthermia. 2014;30(8):531–539.
- Zhang HG, Mehta K, Cohen P, et al. Hyperthermia on immune regulation: a temperature’s story. Cancer Lett. 2008;271(2):191–204.
- Skitzki JJ, Repasky EA, Evans SS. Hyperthermia as an immunotherapy strategy for cancer. Curr Opin Investig Drugs. 2009;10(6):550–558.
- Bendz H, Ruhland SC, Pandya MJ, et al. Human heat shock protein 70 enhances tumor antigen presentation through complex formation and intracellular antigen delivery without innate immune signaling. J Biol Chem. 2007;282(43):31688–31702.
- Todryk S, Melcher AA, Hardwick N, et al. Heat shock protein 70 induced during tumor cell killing induces Th1 cytokines and targets immature dendritic cell precursors to enhance antigen uptake1. J Immunol. 1999;163(3):1398–1408.
- Rovere-Querini P, Capobianco A, Scaffidi P, et al. HMGB1 is an endogenous immune adjuvant released by necrotic cells. EMBO Rep. 2004;5(8):825–830.
- Rovere-Querini P, Capobianco A, Scaffidi P, et al. Local hyperthermia combined with radiotherapy and-/or chemotherapy: recent advances and promises for the future. Cancer Treat Rev. 2004;41(9):742–753.
- Topalian SL, Drake CG, Pardoll DW. Immune checkpoint blockade: a common denominator approach to cancer therapy. Cancer Cell. 2015;27(4):450–461.
- Walunas TL, Lenschow DJ, Bakker CY, et al. CTLA-4 can function as a negative regulator of T cell activation. Immunity. 1994;1(5):405–413.
- Lee KM, Chuang E, Griffin M, et al. Molecular basis of T cell inactivation by CTLA-4. Science. 1998;282(5397):2263–2266.
- Soldevilla MM, Villanueva H, Meraviglia-Crivelli D, et al. ICOS costimulation at the tumor site in combination with CTLA-4 blockade therapy elicits strong tumor immunity. Mol Ther. 2019;27(11):1878–1891.
- Elsas AV, Hurwitz AA. and, Allison JP. Combination immunotherapy of B16 melanoma using anti-cytotoxic t lymphocyte-associated antigen 4 (CTLA-4) and granulocyte/macrophage colony-stimulating factor (GM-CSF)-producing vaccines induces rejection of subcutaneous and metastatic tumors accompanied by autoimmune depigmentation. J Exp Med. 1999;190(3):355–366.
- Affolter T, Llewellyn HP, Bartlett DW, et al. Inhibition of immune checkpoints PD-1, CTLA-4, and IDO1 coordinately induces immune-mediated liver injury in mice. PLoS One. 2019;14(5):e0217276.
- Nolan E, Savas P, Policheni AN, et al. Combined immune checkpoint blockade as a therapeutic strategy for BRCA1-mutated breast cancer. Sci Transl Med. 2017;9(393):eaal4922.
- Formenti SC, Rudqvist NP, Golden E, et al. Radiotherapy induces responses of lung cancer to CTLA-4 blockade. Nat Med. 2018;24(12):1845–1851.
- Liu Y, Dong Y, Kong L, et al. Abscopal effect of radiotherapy combined with immune checkpoint inhibitors. J Hematol Oncol. 2018;11(1):104.
- Takahashi Y, Yasui T, Minami K, et al. Carbon ion irradiation enhances the antitumor efficacy of dual immune checkpoint blockade therapy both for local and distant sites in murine osteosarcoma. Oncotarget. 2019;10(6):633–646.
- Chamoto K, Chowdhury PS, Kumar A, et al. Mitochondrial activation chemicals synergize with surface receptor PD-1 blockade for T cell-dependent antitumor activity. Proc Natl Acad Sci USA. 2017;114(5):E761–E770.
- Brinkmann V, Davis MD, Heise CE, et al. The immune modulator FTY720 targets sphingosine 1-phosphate receptors. J Biol Chem. 2002;277(24):21453–21457.
- Chen T, Guo J, Han C, et al. Heat shock protein 70, released from heat-stressed tumor cells, initiates antitumor immunity by inducing tumor cell chemokine production and activating dendritic cells via TLR4 pathway. J Immunol. 2009;182(3):1449–1459.
- Sen K, Sheppe AEF, Singh I, et al. Exosomes released by breast cancer cells under mild hyperthermic stress possess immunogenic potential and modulate polarization in vitro in macrophages. Int J Hyperthermia. 2020;37(1):696–710.
- Dewey WC, Hopwood LE, Sapareto SA, et al. Cellular responses to combinations of hyperthermia and radiation. Radiology. 1977;123(2):463–474.
- Ando K, Suzuki Y, Kaminuma T, et al. Tumor-specific CD8-positive T cell-mediated antitumor immunity is implicated in the antitumor effect of local hyperthermia. Int J Hyperthermia. 2018;35(1):226–231.
- Chao Y, Chen G, Liang C, et al. Iron nanoparticles for low-power local magnetic hyperthermia in combination with immune checkpoint blockade for systemic antitumor therapy. Nano Lett. 2019;19(7):4287–4296.
- Chi MS, Yang KL, Chang YC, et al. Comparing the effectiveness of combined external beam radiation and hyperthermia versus external beam radiation alone in treating patients with painful bony metastases: a phase 3 prospective, randomized, controlled trial. Int J Radiat Oncol Biol Phys. 2018;100(1):78–87.
- Oei AL, Korangath P, Mulka K, et al. Enhancing the abscopal effect of radiation and immune checkpoint inhibitor therapies with magnetic nanoparticle hyperthermia in a model of metastatic breast cancer. Int J Hyperthermia. 2019;36(1):47–63.
- Victor CT, Rech AJ, Maity A, et al. Radiation and dual checkpoint blockade activate non-redundant immune mechanisms in cancer. Nature. 2015;520(7547):373–377.
- Vanpouille-Box C, Alard A, Aryankalayil MJ, et al. DNA exonuclease Trex1 regulates radiotherapy-induced tumour immunogenicity. Nat Commun. 2017;8:15618.
- Dovedi SJ, Cheadle EJ, Popple AL, et al. Fractionated radiation therapy stimulates antitumor immunity mediated by both resident and infiltrating polyclonal T-cell populations when combined with PD-1 blockade. Clin Cancer Res. 2017;23(18):5514–5526.
- Takahashi Y, Yasui T, Minami K, et al. Radiation enhanced the local and distant anti-tumor efficacy in dual immune checkpoint blockade therapy in osteosarcoma. PLoS One. 2017;12(12):e0189697.